Final GNSSS PDF
Document Details
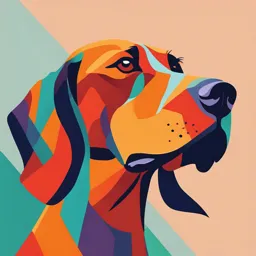
Uploaded by IrreplaceablePentagon3772
University of Ilorin
Abimbola, I.O.
Tags
Summary
This document explores the structure of science, scientific methods, and the scientific revolution. It defines science, identifies its components and products, and discusses its limitations. The chapter also examines the history of scientific societies and the major scientists of the 17th century.
Full Transcript
Chapter One THE STRUCTURE OF SCIENCE, SCIENTIFIC METHODS AND REVOLUTION Abimbola, I.O. Department of Science Education, University of Ilorin, Ilorin, Nigeria. INTRODUCTION All disci...
Chapter One THE STRUCTURE OF SCIENCE, SCIENTIFIC METHODS AND REVOLUTION Abimbola, I.O. Department of Science Education, University of Ilorin, Ilorin, Nigeria. INTRODUCTION All disciplines have their own structures. However, not all disciplines have their structures properly described. This situation may be due to the different ages of disciplines. Science has a structure that we can say cuts across all disciplines known by name. An attempt will be made in this chapter to describe the structure of science. Knowing the structure of science has its value. Science students and all those interested in the subject will know the components of the discipline and they will be able to relate to the discipline in a conscious manner so that during the learning processes, they will know what they are learning. The knowledge of the structure of science will also enable them to know how they can become scientifically literate persons through a judicious combination of knowledge and application of the components of the structure of science. Also, science teachers will understand what they are teaching their students better if they understand the structure of their subject matter. This knowledge will assist them in knowing how to properly organise science content for instruction. Evaluation of students’ learning outcome will be enhanced through this knowledge. First, different definitions of science are presented to demonstrate an awareness of various misconceptions about science to properly situate an acceptable definition of science. Second, the three components of the structure of science are presented in great details, one after the other, to demonstrate what a scientifically literate person needs to possess for knowledge, skills, and attitudes. Finally, the chapter concludes with information on the functions and limitations of science. OBJECTIVES At the end of the chapter, students are expected to: (i) define science; (ii) identify the components of the structure of science; (iii) identify and describe the products of science; (iv) identify and describe the processes of science; (v) identify and describe the ethics of science; (vi) describe the functions of science; and (vii) discuss the limitations of science. (viii) identify the three characterisations of the 17th Century; (ix) describe the history of the two major forms of scientific methods; (x) describe briefly, the life histories and achievements of the major scientists of the 17th Century; 1 (xi) describe the organisation and the roles of the major early scientific societies or academies during the scientific revolution; (xii) discuss the roles of the early scientific societies or academies as the forerunners of scientific societies in the contemporary world; (xiii) list major four major scientific societies or academies in Nigeria; and (xiv) discuss the relevance of the three characterisations of the 17th Century based on the major events of the Century. DEFINITIONS OF SCIENCE Definitions of science vary slightly from scientist to scientist and from one subject area to another. Some people still describe science as an ordered body of knowledge while others define it as a search for explanations to natural objects and phenomena. Generally, we can define science as a body of knowledge, a way of investigating or method, and a way of thinking in the pursuit of an understanding of nature. We can therefore view science as a noun, a verb, and as an adjective. We can define science with its products: Knowledge in the form of concepts, facts, generalisations, principles or rules or laws, and theories, subject to error and change. We can define science with its processes or methods or skills: how scientific knowledge comes about. We can also define science with its motives, or ethics or attitudes that guide the day-to-day activities of scientists. Selecting any of these aspects to define science would be inadequate and misleading. We can consider science at present as an enterprise in which human beings participate to study and give parsimonious explanations of the materials and forces of nature. Science employs a variety of techniques. It has as its motive a desire to know. It assumes orderliness in nature. Understandable and acceptable ethical principles govern the activities of scientists. Science ends with credible concepts in the form of theoretical, empirical and rational constructs. We should take science as a human enterprise, the consequences of which have human implications. In the teaching and learning of science therefore, all aspects of science need attention. A scientifically literate person should therefore possess a body of scientific knowledge, a set of scientific skills, and behave scientifically in his day-to-day activities. PRODUCTS OF SCIENCE Products of science include concepts, facts, generalisations, laws, and theories. Concept A concept is the meaning that we attach to a given symbol or label. Words, formulae and mechanical models are symbols for concepts. There are three types of concepts: Empirical concepts, theoretical concepts, and relational concepts. (a) Empirical concepts are concepts by inspection. They are concrete and observable concepts. Examples include: cell, pulley, aluminium, and so on. Philosophers and scientists do not necessarily share the same meaning for what they regard as observable thing or event. While philosophers usually limit their ideal of observation to what they can perceive with human sense organs, scientists extend their meaning of observation to what they can perceive using extensions of sense organs. Such extensions of the sense organs may include the use of microscope and lenses to see tiny objects; the use of telescope to view distant objects; the use of public address system to extend the sense organ of hearing; the use of trained dogs to detect smell 2 and taste for humans; and the use of dissecting instruments to extend the sense organ of touch. Also, scientists include what they can measure directly using various type of instruments as part of their conceptions of observations. Therefore, they regard what they can measure as an observable thing or event. This is why scientists would treat a thermometer reading of 500C as if it is the degree of hotness of a particular environment at a particular time of the day as human beings really experience it. (b) Theoretical concepts are concepts by definition. They are abstract and observable concepts. Examples include: molecule, absolute zero, gene, and so forth. (c) Relational concepts are concepts that relate two or more concepts together. They cannot exist on their own. Examples include: less, more, equal, inverse, proportional, and so forth. We should note that whether a concept is observable or not is arbitrary. No sharp hue can be drawn separating the two. Also, it is a matter of degree, varying from what can be directly observed using sense organs or that can be measured using simple instruments, to things that can only be measured or observed indirectly. Fact A fact is an event that occurred in the past that people recorded with no disagreement among observers. This does not mean that a disagreement may not occur in the future, in which case the fact of the past may not be a fact in the present if there are changes or revisions in observations, the object of observation, or the meaning attached to the observation. An example of a fact is: “An insect has three pairs of legs.” Law A scientific law or principle or rule or generalisation is a brief statement or mathematical formula predicting inter-relationships among concepts. Scientists usually base their predictions on all instances of the concepts of the phenomena that had been observed up to that time. Laws, therefore, describe regularity and order in our observations of natural phenomena and events. They do not prescribe. Scientific laws are not laws of society that members of the society cannot break. For this reason, scientific laws are not “broken” because they are not commands. It is not even necessary to break them. They are subject to modification and abandonment if scientists find them to be inaccurate. There are two types of laws; Empirical law and theoretical law. An empirical law is one that refers to observable concepts or concepts by inspection that does not provide explanation for the relationship it predicts. An observable concept is one that people can observe directly. An example of an empirical law is Ohm’s law: “The electric current in a circuit is directly proportional to the electromotive force and inversely proportional to the resistance”. All the concepts in this statement are either directly observable or measurable or, at least, we can observe their manifestations indirectly. A theoretical law is more advanced than an empirical law. A theoretical law refers to theoretical concepts and provides explanation besides prediction. Therefore, a theoretical law predicts as well as explains. The value of a theoretical law lies in its ability to predict new empirical law. A theoretical law is usually not generalised from observations, it is invented. Also, it is usually more general than an empirical law. An example of a theoretical law is: “At all temperatures other than absolute zero, the motion of gas molecules is random concerning both rate and direction”. While it is easier for an empirical law to contain key concepts that are wholly observable, a theoretical law is usually not easy to formulate without using a few concepts that are observable or measurable. Hence, in the above example, only “absolute 3 zero” and “molecule” are theoretical concepts, while “temperatures” are observable because they are measurable. The presence of observable concepts in a theoretical law makes it possible to derive empirical laws about things that are observable from theoretical laws about things that are unobservable. Correspondence rules or bridge principles guide the connection of theoretical terms with empirical terms. The assumption in this is that theoretical laws are based on observational facts. The fact that we can view concepts like “temperature” as both empirical and theoretical makes this assumption to break down. Theories are supposed to determine what we observe because observations are theory laden. The line separating observable from non-observable, again, is arbitrary. If no clear-cut distinctions exist between empirical and theoretical terms, may be, there is really nothing to bridge! Theory A scientific theory is more advanced than a law. A theory combines several laws in its formulation. A classical definition of theory states it as a system of logical reasoning that scientists carefully construct to explain the unknown, based on valid assumptions about natural phenomena. It contains both observable and unobservable terms. We cannot directly interpret the unobservable terms. A modern view of theory holds that it is a way of viewing the world. In formulating a theory, scientists usually employ a model that is easy to understand, and some logical relationships from which they can make deductions. Students should note that theories are not laws in waiting. Laws and theories perform different functions in science. Laws are not necessarily better than or more superior to theories. In fact, when theories are used in explanations, concepts, facts, and laws are employed to make valid explanations. Scientific laws use more mathematics than scientific theories for precision sake. This situation explains why there are more laws in chemistry and physics than biology. Additionally, biology has more theories than laws because of its nature. Biology, as the study of life, is difficult to describe in abstract mathematical terms, because of the complexities of life. An example of a theory is the kinetic theory of gases as Body (1970) stated it: 1. All gases are composed of many small molecules that are in a state of perpetual rapid motion in straight lines. 2. At normal pressures, the total volume of the molecules is very small compared to the total volume of the gas. 3. The molecules are spherical, elastic, and smooth. 4. The pressure exerted by the gas is the force per unit area due to the impact of the individual molecules on the wall of the containing vessel. 5. Two gases are at the same temperature when the mean kinetic energy of the individual molecules of the-two gases is the same (p.183). Another example is Darwin’s theory of evolution that we can state as follows: 1. There is an overproduction of all living things. 2. This results in a struggle for existence (competition) with the survival of the fittest. 3. Those best fitted are the ones with variations that adapt them to their environment. 4. Natural selection destroys the ones that adapt poorly. 5. Those, which survive, reproduce and pass on their variations to their offspring (Abimbola, 2002, p. 26). 4 All theories must take, into account the known facts, however, scientists never prove theories true or false; they are only adequate or inadequate to explain a phenomenon. Theories, therefore, vary in credibility. This credibility criterion is probably based on the cumulative view of scientific progress. However, a theory can still be credible even when it is not consistent with known facts, especially if it is from a different perspective that may lead to a scientific revolution. This is the only way by which scientific progress is possible. When a scientist has a choice of two theories, one complex and one simple, and both are adequate for use in explaining the phenomenon, he, or she selects the simplest theory. When a scientist has a choice between a theory that can change and one that cannot change, and both are adequate in explaining the phenomenon, he, or she selects the theory that can change. A theory serves as a basis for deducing empirical consequences from it. A theory is useful in explaining observed data. We can also use a theory to predict future events. PROCESSES OF SCIENCE Processes of science are the methods and skills that scientists employ in their work. Processes of science are the scientific activity per se. We can state them in a noun form or in a verb form. However, in listing scientific processes, we must not mix together the noun form and the verb form. The following processes are in the verb form: Observing, classifying, inferring predicting, measuring, communicating, interpreting data, making operational definitions, formulating questions and hypotheses, experimenting, and formulating models. Observing Observing takes places in a variety of ways using all the sense organs. We observe using direct sense experience with the aid of our sense organs. Where direct observation is not adequate or feasible, we use indirect methods of observation. We observe the qualities and quantities of objects and events. The precision of observations is important if eventually we are going to infer from such observations. We improve the precision of observations by making quantitative observations. Observations vary with the background knowledge of the observer. Classifying Classifying is the grouping or ordering of phenomena according to a scheme that we establish for that purpose. We may classify objects and events based on observations. We base classification schemes on observable similarities and differences in properties that we arbitrarily select. We use classification schemes to group items within a scheme as well as to retrieve information from a scheme. Inferring The process of inferring, while based on observation, requires evaluation. Inferences that are based upon one set of observations may suggest further observations, which in turn, require modification of original inferences. Inferences lead to prediction. Predicting The process of predicting involves the formulation of a result that we expect based on experience. The reliability of prediction depends upon the accuracy of past observations and upon the nature of the event being predicted. We base prediction on inference. Progressive series of observations and, in particular, graphs are important tools of prediction in science. An experiment can support or contradict a prediction. 5 Measuring We measure properties of objects and events by direct comparison or by indirect comparison with arbitrary units. We need to standardise these units to make for easy communication. We can relate together identifiable characteristics that we can measure to provide other quantitative values that are valuable in the description of physical phenomena. Communicating To communicate observations, we need to keep accurate records that we can submit for checking and re-checking by others. We can represent in many ways, accumulated records and their analyses. Scientists often use graphical representations since they are clear, concise and meaningful. Complete and understandable experimental reports are essential to scientific communication. Interpreting Data Interpreting data requires the application of other basic process skills–in particular, processes of inferring, predicting, classifying and communicating. It is through this complex process that scientists determine the usefulness of data in answering the question that they are investigating. Interpretations are always subject to revision considering new or more refined data. Making Operational Definition We make operational definitions to simplify communication concerning phenomena that we are investigating. In making such definitions, it is necessary to give the minimum amount of information that we need to differentiate that which we are defining from other similar phenomena. We may base operational definitions upon the operations that we are performing. Operational definitions are precise and, in some cases, based upon mathematical relationships. Formulating questions and hypotheses We formulate questions based upon the observations that we make and they usually precede an attempt to evaluate a situation or event. Questions, when we state them precisely, are problems that we want to solve through application of the other processes of science. The attempt to answer one question may generate other questions. The formulation of hypothesis depends directly upon questions, inferences and predictions. The process consists of devising a statement that we can experiment. When a set of observations suggests more than one hypothesis, we must state each of them separately. We state a workable hypothesis in such a way that, upon testing, we can establish its credibility. Experimenting Experimenting is the process of designing data-gathering procedures as well as the process of gathering data to test a hypothesis. In a less formal sense, we may conduct experiments simply to make observations. However, even here, there is a plan to relate cause and effect. In an experiment, we must identify and control variables very much. We design an experimental test of a hypothesis to indicate whether to reject, modify, or not to reject the hypothesis. In designing an experiment, we must consider the limitations of method and apparatus because these play a role in determining what we observe and the veracity of the findings. ETHICS OF SCIENCE The ethical standards of the disciplines of the sciences guide the actions of the scientists. Scientists view their observations and conclusions as another approximation of what we commonly call truth; however, they see truth in the absolute sense to be impossible. The following are some of the ethnical attributes that scientists are supposed to possess. They are also attributes that a person that is scientifically literate should show in his or her behaviours. 6 Curiosity and Science Scientists must be very curious. Curiosity is spontaneous desire on their part to explore the environment and to learn about its phenomena. Curiosity concerning the physical universe is the fundamental driving force in science. It is the starting point for science. It is the same across the barriers of time, race and geography. It requires that one does not satisfy oneself with the status quo. This attribute is natural in children and students. All it may require is for somebody to encourage it. Open-mindedness Scientists should keep an open mind to things and events. There should be openness concerning investigation that such factors as religion, politics or geography do not limit. They should be willing to question the status quo. They should always maintain a doubting attitude of a sceptic and not allow themselves to be too gullible to spurious data. Positive Approach to Failure Scientists should maintain a positive approach to failure. They should make positive use of failure. For every failed attempt, scientists should know that they have learned at least one way of not doing the same thing the same way again. To achieve this attitude, one requires persistence and perseverance. It requires that one be not easily discouraged. Objectivity in Science Scientists are to view all within their discipline objectively; they must be impersonal, impartial, and detached from prejudice as they make observations and formulate conclusions. They must be willing to look at many sides of an issue without prejudice. There must be a general reluctance on the part of scientists not to let their personal emotions affect the decisions that they take. As long as we view science as a human enterprise, it may be difficult to be absolutely objective as we make observations. Human experience colours what they see things as. Respect for the Opinion of Others Scientists should have respect or tolerance for the ideas or opinions of others. This requires that scientists do not adopt an attitude where they appear as all-knowing and infallible. In fact, opinions of other experts and novices can be crucial in hitting on ideas that may be useful or damaging to an idea that one may strongly hold before. The science community relies so much on the ideas of members of the community to validate discoveries and inventions that scientists make within the community. Willingness to Suspend Judgment Scientists should always be willing to suspend judgment. This requires that they do not jump to hasty conclusions. They should wait for all necessary facts to come in before judging and concluding. Persons that possess this scientific attitude will not involve themselves in rumour mongering or tale bearing. Despite the fact that science progresses through revisions of old ideas that were once correct, the revisions that might be necessary for ideas that were hastily believed would be of a different kind. They are revisions that would have not been necessary if adequate care had been taken initially. Willingness to Accept Criticism Scientists should always be willing to allow others to question their ideas. Criticism contributes much to the progress of science. This attribute requires that individual scientists submit their works for others to criticise them. They should also be willing to consider the criticisms with open minds so that they can be willing to do something about them. Most academic and professional journals would not publish a research report until appropriate corrections suggested by blind reviewers have been implemented. There is the possibility that good works may be prevented from seeing the light of the day because of requirements like this. However, the integrity of science has to be guarded jealously to prevent frivolous discoveries from being published and recognised. Also, adequate care is taken by the editors 7 to ensure that reviewers are persons sufficiently knowledgeable in the areas in which they are given papers to review. Unwillingness to Believe in Superstitions A superstition is a folk idea, belief, or custom that is based on ignorance and irrational fear of the unknown. It started as an attempt to cope with a world full of stress and mysteries such as births and deaths, rain, flood, lightning, drought, eclipses, rainbow, and so forth. In spite of the progress made in science, no country of the world is completely free of superstitions. However crude, superstitions are an initial attempt to explain and deal with a mysterious world. Most of the science of the Middle Ages may now appear superstitious, but they provide the needed insight into the future that made subsequent progress in science possible. Superstitions may appear foolish to scientifically literate persons, but they are not, to persons believing them. To be able to disbelieve superstitions, it requires that one seeks to understand the superstitions and the scientific explanations for them. Providing scientific explanations for superstitions and teaching them early to students may serve to reduce the number of persons that will grow up with superstitions. This is the task of all science teachers. Science is Dynamic Scientists seek relationships among data and formulate generalisations in the form of concepts, laws and theories. More refined data comes with each new method of making observations. We may modify or reject the generalisations altogether as more data accumulate. This requires an openness of mind that allows for willingness to change one's opinion in the face of evidence. Concepts in science are dynamic, not static. They have only temporary status. Also, science is self-correcting. Cooperation among Scientists Scientists work alone or in groups. Most of the time, they find themselves working in groups. They, therefore, must learn and be willing to cooperate with others when working on group activities. Due credit must be given to individual's contribution to group activities. Selfish and narrow interests must give way to the larger interest of advancing the progress of science. Science is Anti-authoritarian Science is not subject to the whims and caprices of any other authority other than itself. The real authority of science resides in the logic of the discipline, the data from nature, and the force of the scientific community. The basis of scientific knowledge is consensus among self- appointed experts. Science begins with facts and ends with facts. This does not depend on what kinds of structures we build in between. For this reason, we do not expect anybody to forcefully pronounce a discovery or invention that is not scientific as scientific. Accuracy of Observations and Reports The practice of science requires that we make accurate observations and reports. Scientists must be unwilling to compromise with the truth. Observations must be very free from the bias of the observer. The nature of the hypothesis that we are testing determines the nature of the observations that we intend to make. Observations depend on theory. This means that scientists rarely make observations without a pre-planned purpose for making it. Morality and Science In pure scientific research, scholars have no obligation to concern themselves with the moral implications of their work. This is why scientists that worked on the description of the structure of atoms are not morally liable for the consequences of the atomic bomb that is capable of mass destruction. However, in applied scientific research such as in genetic engineering, scholars have obligation to concern themselves with the moral implications of their work. This is why scientists that developed the atomic bomb who should know the consequences of the use of the bomb are more liable. This is why the United States Government banned research into the cloning of human beings while scientists are free to clone plants and animals. Most scientists believe that a decision to participate or not 8 participate in research that could pose risks to society is a matter of personal ethics rather than professional ethics. Orderliness of Nature and Natural Laws Scientists appreciate that nature is orderly and natural laws are orderly. This appreciation is helpful to them in spotting anomalous events in nature. Their experiences have taught them to expect certain things to happen under certain conditions and at particular times. An awareness of anomaly is a first step that nature is orderly and its laws are orderly. The Beauty in Nature When there is orderliness in nature, there is bound to be beauty in it. For instance, things in nature have shapes, sizes, colours, textures, and arrangements that occur regularly with respect to each type of thing. A combination of these in nature provides both orderliness and disorderliness that together make things look beautiful. Scientists, in part, embark upon scientific inquiries because of the appreciation of the beauty in nature and the fascination that beautiful events, phenomena, and objects offer. We can find some examples of beauty in nature in the scenery provided by undulating mountains, the sky at night, the flora and fauna in the rain forest, ocean, and so forth. Universality of Science Science is universal. Scientists assume that the universe is a vast single system in which the basic rules are the same everywhere. All scientists have obligation to publicise their findings and formulations to the scientific community. Whichever findings scientists publicise first have priority of research. THE FUNCTIONS OF SCIENCE Science describes the world in form of qualities and attributes that human beings can understand using familiar descriptors such as numbers, shapes, sizes, weights and ratios. In doing this, science demonstrates that we are related to the whole universe by being a part of it and therefore, we also have a place in it. Science is knowledge. We can make the study of science as an end in itself. Science, therefore, performs an intrinsic function by serving as a subject matter for study that will provide individuals with a sense of intellectual satisfaction, especially when we make particular observations that demonstrate the regularity and unity in nature's design. Science satisfies our desire to know. When science describes the world adequately, such a description enables us to predict what will happen if we take particular actions. Science, therefore, through its method, guides us through particular courses of actions that would lead to particular outcomes. The method is also useful in evaluating the outcomes to be sure that they are valid outcomes. The range of things that we can achieve now or in the future through science is limitless, but the use to which we shall put these things is not within the subject matter of science. THE LIMITATIONS OF SCIENCE The method of science is only one of the methods of knowing that yields knowledge. Therefore, science is one kind of knowledge that human beings base decisions upon. It does not take decisions by itself. Hence, science, especially pure science, does not concern itself with moral issues. However, scientists in applied science have to contend with moral issues that relate to the knowledge arising from their work. For instance, a scientist attempting to clone a human being cannot claim ignorance of the full implications of the outcome of his or her work. 9 Science summarises observations in the form of laws. These laws are as certain as the instruments that we use and the inferring techniques that we employ, allow. It assumes normal conditions while doing this. Science uses these summaries to predict unknown past and future events. If there is any change in the conditions that scientists assumed initially, predicted events may not occur. It is difficult for scientists to deduce, with certainty, any past or future event because past and future conditions are difficult to be wholly known. The farther away these past and future events are, the more difficult they are to deduce with certainty. There is always the possibility of changes occurring in the laws that we apply now that we cannot anticipate at present. Even when these laws applied to past events, we have no way of saying, with certainty, that they will apply to future events, and vice versa. Science does not deal with final causes. Hence, it does not accommodate miracles in its subject matter. Unexpected events do occur during scientific investigations. These events are usually explained in the end using appropriate theories and laws. Whatever scientists are unable to explain remains a challenge to the scientific community and their members will not rest until they are able to reduce the unknown to the familiar. Scientific laws are generalisations that summarise observations. They are not decrees or a legal enactment that are enforceable. The only authority of science resides in the logic of human beings and the force of the scientific community. The scientific community sanctions what it considers to be a major scientific discovery or invention. It does not lay down any set of rules for arriving at a discovery or invention because it is not a mechanical process. REVOLUTION IN SCIENTIFIC METHODS The 17th century is very significant in many respects. The many names by which the century is characterise attest to this significance. We characterise the century variously as the century of "The Scientific Revolution," “The Century of Genius,” and “The Century of Scientific Societies.” (Mendelsohn, 1982) This chapter is therefore organised in a similar fashion to the major developments of the century. The chapter opens with the history of the development of the scientific method by Francis Bacon and Rene Descartes. Next, is the description of the life and achievements of the century's geniuses, such as: Galileo Galilei, Johannes Kepler, William Harvey, and Isaac Newton. Finally, the chapter closes with a description of the early scientific societies or academies—their organisation and their role as the forum for the dissemination and exchange of ideas. THE HISTORY OF THE SCIENTIFIC METHOD The scientists of the earlier centuries had interest in making discoveries and inventions. They placed very little emphasis on the practice of science. Perhaps, this is understandable because even in this modem time, theorising about the practice of science is not the business of scientists. Philosophers of science have taken it upon themselves to describe what scientists do, the standards they follow, and should follow, and they describe the standards that their discoveries and inventions should meet. Occasionally, some philosophers of science are former scientists who left practical science. It does appear that a good knowledge of science is a prerequisite for being a knowledgeable philosopher or historian of science. 10 During the 17th century, the movement to study science by observation and experimentation that began in the 16th century now became a scientific revolution. It was the period that scientists paid the greatest attention to the scientific method. The sheer number of persons that paid attention to method then, indicated the need for an acceptable method of conducting science. Among the people that paid great attention to scientific method during this period were: Francis Bacon and Rene Descartes. Westfall (1977) has credited Robert Boyle with, perhaps the best statement of the experimental method that focused on "the activity of investigation that distinguishes the experimental method of modern science from logic”. (p. 115). Pascal, Gassendi, and Newton also wrote on scientific method. Westfall (1971), put the date, when experimental method of modem science started to have influence on the scientific community, at about the year 1590. This was the time, scientists started to base their work on deliberately contrived experiments. Taylor noted that Galileo Galilei (1564-16431 was the first scientist to employ the modem scientific method fully in physics and astronomy. Before then, Aristotle's syllogistic method of reasoning, described in his organon dominated science. Also, Westfall (1977) stated that Galen's writing in his physiology contained examples of experimental investigation. Westfall also claimed that Robert Grosseteste of the medical school, and the logicians based at the University of Padua, Italy in 16th Century, discussed the precursors of hypothetico-deductive method, Furthermore, during the period before the scientific revolution, natural history characterised science during which scientists made observations and recorded them carefully. This method led to a general feeling of disillusionment among scientists. The general feeling of disillusionment had to do with results of scientific investigations that did not match the efforts put into them. The Scientists of the time blamed the method of conducting science, for the low output. However, the emphasis on method during the period of the 17th century paid off with several discoveries and inventions during the period and beyond thereby giving the impression, albeit unintentionally, that science is synonymous with its method. Francis Bacon wrote a book on the philosophy and method of scientific investigation titled, Novum Organum (the New Instruments, 1620). He was perhaps the first in the 17th century to formulate a series of steps to account for the scientific method in his book (Taylor, 1963). The book was a reaction to Aristotle's treatise in logic referred to as the Organum. Aristotle's Organum is the “old method of reasoning” while Bacon's Novum Organum is the "new method of reasoning.” Bacon based his method on the inductive method of objective observation and experimentation without preconceptions. He wrote Novum Organum in two books. The first book classifies idols that are impediments to learning; (1) idols of the tribe that are errors inherent in human nature; (2) idols of the cave that are errors that result from individual background and experience; (3) idols of the market place that are errors resulting from the use and misuse of language; and (4) idols of theatre that are errors arising from false philosophical systems. The second book contains an examination of the inductive method of experimental science for use in remedying these idols, and to serve as a basis for making progress. Francis Bacon's account of the scientific method in his Novum Organum (1620) has four steps that include: 1. To collect reliable tested information, especially by means of experiments. 2. To classify this material by "tables of invention" so that all the instances of the phenomenon studied could be compared. 11 3. By these tables, he would arrive at minor generalisations, which we would call theorems or rules, and by comparing these, he would rise to general Scientific Laws. 4. These laws, when found, must confirm themselves by pointing out new instance of the phenomenon studied. (Taylor, 1963. p.97) The formulation of these steps in the scientific method marked a major landmark in the systematisation of science. Francis Bacon himself was not a practicing scientist. Also, he made no scientific discoveries, He was a lawyer by profession and his legal background probably inspired him to write on how to systematise science. He also proposed a system for forming a scientific organisation. In a fiction called The New Atlantis, Bacon wrote of an academy of science where scientists worked together on projects and gave reports on their work before the whole academy. English scientists set up the institution that later became the Royal Society of London in 1660. They set it up according to Bacon's ideas. Another person that worked on the systematisation of science was Rene Descartes (1569- 1650). Descartes was a mathematician and philosopher as well as a scientist who believed in reasoning. His “Discourse on Method” was also a new way of finding the principles of nature. His proposed rules of investigation as follows: The first was never to take anything as true that I did not know evidently to be so. The second, to divide each of the difficulties which I might examine into many portions as should be possible and should be necessary, the better to resolve them. The third, to conduct my thinking in an orderly manner, beginning with the objects most simple and most easy to understand, in order to rise little by little, as if by steps, up to the knowledge of the most complex; supposing moreover that there is an order even among those that do not proceed naturally one from the other. And the last, always to make enumeration so complete and reviews so general, but I should be certain of having omitted nothing. (Descartes,1960, p.15.) While Bacon advocated experimentation and induction, Descartes preferred mathematical reasoning and deduction. Bacon disregarded the role of prior conceptual knowledge about the data that scientists are to collect and placed too much emphasis on gathering facts. These facts need to be relevant to the problem. Also, he underestimated the role of mathematics in the physical sciences. Descartes, on his part placed too much emphasis on mathematical reasoning about facts. He placed little emphasis on observation. In any case, the two opposing methods were available for scientists to integrate, and fortunately, Newton did just that. A CENTURY OF GENIUSES The next several paragraphs will describe the works of notable scientists of the 17th century that are products of the scientific revolution. The list includes great scientists such as: Galileo, Harvey and Newton. Galileo Galilei (1565-1643) We usually freely refer to Galileo as the first modern scientist because, as earlier mentioned, Galileo was the first person to employ the scientific method in its fullness, and Galileo was 12 an Italian astronomer and physicist born in Pisa in 1364. His two great books are Dialogue on the Two Chief systems on the World (1632) and Discourses on the Two New Sciences (1632). Although Galileo was best known for his mathematical studies of the motion of bodies on earth, he also invented a number of new scientific instruments. For example, he invented the first thermometer, called, air thermometer. He also made larger and more powerful telescopes. He used these new telescopes to make observations of the moon and the planets. Galileo's telescope studies of the moon revealed that the moon was not a smooth sphere shining by its own light as had been believed. Instead, the moon's surface showed great mountains and craters and it showed only reflected light. Galileo also observed that the planet Jupiter had moons revolving around it, just as our moon revolves around the earth. He also demonstrated that the Milky Way was really made up of many stars, which previously had not been visible. His studies of the heavenly bodies increased his conviction that the sun was the centre of the solar system as stated by Copernicus (the heliocentric theory). Galileo began opposing the old description of the universe because the earth appeared to him to move. It was not stationary as stated in the Aristotle-Ptolemy theory of the universe. We usually refer to the Aristotle-Ptolemy theory as the geocentric theory of the universe. The Copernican theory of the universe (and its modern modifications) is known as the heliocentric theory, which posits that the sun is at the center of the universe. The belief in the heliocentric theory got Galileo into trouble with the Church and supporters of Aristotle's theory. Johannes Kepler (1571-1630) and the Laws of Planetary Motion Johannes Kepler was a German and one of the outstanding astronomers the world has ever produced. His work helped explain the motion of the planets around the sun. Kepler accepted the Copernican theory of the universe and made changes in it. His main work was the study of motion of mars. He proved, mathematically, that the planets orbit the sun (being a Copernican) in elliptical paths rather than in perfect circles. This was after he had tried various kinds of "oval" paths that led him to the idea of an elliptical path. Kepler formulated the following three laws of planetary motion to explain, more accurately, the motions of the planet: 1. The planets describe ellipses about the sun, the sun being in one focus. 2. The planets move so that the lines joining the sun to the planet sweep out equal areas of the ellipse in equal times. 3. The squares of the periodic times of the planets are proportional to the cubes of the major axes of their orbits (The periodic time of the planet is the time of one revolution about the sun: the major axis or an ellipse is part of the straight line passing through its foci that is cut off by its circumference) (Taylor, 1963, pp. 115-116). These laws are still in use today in astronomy and in planning the orbits of artificial satellites. Kepler wrote a number of books among which are: Mysterium Cosmographicum ("Cosmic Mysteries"), Astronomia Nova ("New Astronomy"), and Harmonices Mundi ("The Harmony of the Universe. He died on November 15, 1630. William Harvey (1578-1657) and the Circulation of the Blood William Harvey was an English Doctor and Scientist. He was born on April 1, 1578. Before Harvey, the heart had been thought of as the source of life, and the seat of the emotions. 13 Harvey showed it to be a pump that kept the blood in circulation through the body in a closed system of blood vessels. Harvey was unable to establish the connections between the arteries and the veins. He gave a series of lectures on the circulation of the blood before the Royal College of Physicians in 1616. He published the lecture in a book, titled: De Motu Cordis et Sanguinis ("On the Motion of the heart and blood") in 1628. Harvey is usually regarded as the founder of the science of physiology. He died on June 3, 1657. Isaac Newton (1642-1727) Isaac Newton was one of the greatest scientists of all time. It is appropriate to have him discussed last in this section, because he clearly represents the height of the scientific revolution. He is the symbol of final change from early science to modern science. For example, he changed early empirical science into modern experimental science by merging the observation and experimentation of Bacon with the mathematical reasoning of Descartes. He is best known for his discovery of the law of universal gravitation and laws of motion. Isaac Newton was born prematurely on Christmas Day in 1642, in Woolsthorpe, England. His father, a farmer, died a few months before Isaac was born. When the boy was three years old, his mother remarried and moved to another town. Isaac stayed in Woolsthorpe with his grandmother. He attended King's School at Grantham. At first, he was a poor student but he later became an outstanding one. When Newton's stepfather died in 1656, his mother returned to Woolsthorpe. She withdrew Isaac from school to manage his father's farm with her. Newton turned out to be a very poor farmer and he was subsequently sent back to school. He graduated in 1661. The same year, at the age of eighteen, Isaac Newton entered the Trinity College, Cambridge. Newton graduated from Cambridge in 1665 at the age of 22 without honours or distinction. In addition, he worked out the basic formula for the binomial theorem that year. The spread of the Great Plague, the Black Death, caused the closing of Trinity College for 18 months and prevented Newton from continuing his studies uninterrupted. During these 18 months, Newton laid the foundation for most of his famous discoveries in mathematics and the physical sciences. For instance, it was during this time he hit upon the calculus, a new mathematical tool. The study of light was among Newton's earliest work in science. While on this forced vacation at Woolsthorpe, Newton used a prism to carry out a series of experiments with sunlight. He succeeded in showing that a beam of sunlight (white light) is made up of bands of many colours of light ranging from red to violet, as in rainbow. He called these bands of colours, the spectrum. At the end of the plague in 1667, Newton returned to Trinity College to continue work on light and colour. This work led him to the discovery of the reflecting telescope. The world's largest telescopes are reflectors. (The reader may be interested in knowing that the largest optical telescope is in the former Soviet Union. on Mount Pastuhov in the Caucasus. Its mirror is 600 centimetres (236.2 ins) in diameter. The second largest is located on Mount Palomar in California, USA. Its mirror is 508 centimetres (200 inches) in diameter. Newton's theories of the nature of light were very influential. Newton's particle-theory replaced Huyghens wave theory of light. In recognition of his work in Mathematics and 14 optics, Newton was appointed Professor of Mathematics at Trinity College in 1669 to succeed Isaac Barrow, his favourite teacher. His book, Optics was published in 1704. Newton's greatest and most influential book is the Principia or Mathematical Principles of Natural Philosophy (Philosophiae Naturalis Principia Mathematica), which was published in 1687. In this book, Newton was able to show the mathematical proof of the law of gravitation earlier figured out by Johannes Kepler. He also concluded a series of studies made by Gilbert, Galileo and others. He therefore brought into one science the motion of celestial bodies and the movement of bodies on the earth. During later years, Newton played a more active role in public life. He served as a member of Parliament (1689), Warden (1695), and Master of the Mint (1699). In 1703, he was elected President of the Royal Society, and served in this capacity until his death. He became a knight in 1705. Isaac Newton died in 1727 and he was buried in Westminster Abbey. His statue stands today in the hall of Trinity College, Cambridge University. ORGANISATION OF SCIENTIFIC SOCIETIES The formation of scientific societies was one of the most important developments of the 17th Century. These societies filled the vacuum left by the decline of early universities. There was a felt need to disseminate and exchange scientific information with like-minded colleagues, hence, the formation of these societies or academies. The earliest of these academies or societies was established in Rome in 1603. It was called the Academia deiLincei. This Academy replaced its 1560 counterpart, the Academia Secotorium Naturae founded in Naples and which was closed down for meddling with witchcraft. Galileo was one of the members of this new academy. The members of the society were the first to use the microscope for scientific studies. The name, "microscope," was coined by the members. The society split over the Copernican theory in 1615 and it was dissolved in 1667 when Leopold Medici was made a Cardinal. The Royal Society of London is today the oldest association of scientists. The society grew out of a series of informal meetings called, "Invisible College" that was held in London and Oxford. The society started meeting formally in 1660. In 1662, King Charles 11 gave the society his seal of approval with the name "Royal Society for the Improvement of Natural Knowledge." The society was privately sponsored. The society served as a forum for presenting papers on topics of scientific interest and for presenting demonstrations and experiments. The Royal Society started publishing a journal the Philosophical Transactions, in 1665. The journal still exists. In 1666, the French Academie Royale des Sciences (Royal Academy of Sciences) started its formal meetings in Paris. In 1662, it started to publish a journal. This society replaced an earlier one formed in 1654 under the auspices of Herbert de Montmor (1600-1679). It disbanded due to financial difficulties. The French King became interested in science and so the society became part of the Royal Court. The King paid the members of the society. The King also provided material support. The members of the society in turn carried out crown-supported research and became the inspectors of patents and the designers of new machines. 15 In 1609, the academy consisted of 70 members whose positions were arranged in a hierarchical order. The members' rights and privileges followed this order until the time of the French Revolution when the academy was re-organised. Members were subsequently granted equal rights and privileges. The Academy was responsible for the development and adoption of the metric system of measurement. Thus, France was the first to recognise the importance of national science. It is important to mention here that we in Nigeria have Nigeria Academy of Science (NAS). There is the need to find out more about the Nigeria Academy of Education (NAE). These are of professional interest to us as prospective scientists and teachers. Another one is Nigeria Academy of Letters (NAL) for Humanities professionals. Most of the early and modern scientific societies published scientific periodicals that carried reports of the latest experiments, calculations, tables, and diagrams. They replaced the book as the basic means of transmitting current scientific information. The scientific societies also provided a forum for checking scientific discoveries and they established unwritten rules for scientific work. It was only fairly recently that the modern philosophers of science began emphasising the important role the scientific community, through these societies, plays in providing appropriate checks and balances for the practice of science. However, space constraints in journals and time constraints in conferences do not seem to permit meaningful exchange of ideas as before. CONCLUSION We have made an attempt in this chapter to define science and describe its structure, functions and limitations. An adequate definition of science needs to include all the three components of the structure of science. These three components include, science products such as concepts, facts, laws and theories; science processes, and the ethics of science that include, attributes that characterise scientifically literate persons. Science describes the world as adequately as current instruments, inferring techniques, and knowledge allow. Science is therefore both knowledge and method but it is not everything. Science has its limits that set boundaries for what counts as a problem, the methods that will be appropriate, and what counts as a solution. More solutions lead to more problems in an ever-ending cycle. An attempt has been made in this chapter to present the history of the development of science during the period of the scientific revolution of the 17th Century. The century was marked by three major achievements, namely, the formulation of scientific methods, the production of rare scientific geniuses, and the organisation of scientific societies. This chapter therefore, first traced the history of the scientific method as formulated by Francis Bacon and Rene Descartes and used gainfully by Galileo and Newton and other scientists of the century. Second, the chapter described the life history and the major contributions of the century's men of science such as Galileo, Kepler, Harvey, and Newton. Finally, there was a description of the organisation of scientific societies in Italy, England, and France. These societies or academies set the standard and the pace for the present-day scientific societies. The Royal Society even exists till today. The scientific revolution of the 17th century provided the basis upon which most of the scientific practices of the 18th, 19th, and 20th centuries depended. 16 In general, the revolution of the scientific method led to the scientific revolution of the 17th century while the scientific revolution led to the industrial revolution of the late 18th and early 19th centuries. The term, "industrial revolution,” was used to describe the period of dramatic economic and technical change in Britain during the period 1760 to 1850. EVALUATION STRATEGIES Practice Questions 1. Look up the following words from the dictionary and find out their meanings, derivations (origins), related idioms and synonyms: structure, science, product, process, ethics, concept, fact, law, theory, inferring, predicting, interpreting, hypotheses, experiment, objectivity, superstitions, dynamic, universal, and limitations. 2. Use the words in several sentences with your study partner until you have clearly understood them. 3. What is science? 4. Describe, in detail, the basic components of the structure of science. 5. “Concept difficulty can be measured in terms of the degrees of complexity, sophistication and abstractness of the concept.”Explain what you understand by this statement using appropriate illustrations. What are the implications of the statement for the learning of science? 6. What do you understand by "the structure of science”? 7. State two differences between an empirical law and a theoretical law. 8. Identify three major types of concepts. Give one example each from your major subject. 9. Identify and state two laws and two theories from your major subject. 10. Identify and describe five science processes. 11. Identify and describe five scientific attitudes that characterise a scientifically literate person. 12. State two functions of science. 13. Discuss the limitations of science. 14. Why do we refer to the 17th Century as the century of "the Scientific Revolution"? 15. Justify the characterisation of the 17th Century as "the Century of Genius." 16. Why is the 17th Century usually referred to as, “the Century of Scientific Societies”? 17. Distinguish between the terms "Scientific Revolution" and "Industrial Revolution." 18. Why did Francis Bacon entitle his book, Novum Organum(New Instruments)? 19. State the basic steps of Francis Bacon's scientific method. 20. State the basic steps of Rene Descartes' scientific method. 21. Name one major scientific achievement associated with each of the following great scientists: Galileo Galilei; Johannes Kepler; William Harvey; and Isaac Newton. 22. Name one early scientific society formed in each of the following countries: Italy, Britain, France, and Nigeria. 23. What period year(s), do we refer to as the 17th Century? 17 REFERENCES Abimbola, I. O. (1981), Discovery teaching and learning in science education: A critique based upon the conceptions of discovery held by philosophers of science. Unpublished Master’s Research Paper. University of Wisconsin-Madison, Madison Wisconsin, U.S.A. Abimbola, I. O. (1983). The relevance of the new philosophy of science for the science curriculum. School Science and Mathematics. 83(3): 181-193 Abimbola, I. O. (1992). Conceptions of Sciencing held by Philosophers of Science: Implications for the teaching and learning of science at the university level. Ilorin Journal of Education. 12:113-128 Abimbola, I. O. (2002). Basic Concepts in S.S.C.E. & U.M.E. Biology. Osogbo: Olatunbosun Publishers. Abimbola, I. O. (2006). Philosophy of science for degree students. Osogbo: Olatunbosun Publishers. Abimbola, I. O., &Danmole, B. T. (1995). Origin and Structure of Science Knowledge: Implications for concept difficult in science. Ilorin Journal of Education. 15:47-59. Angeles, P. A. (1981). Dictionary of Philosophy. New York: Barnes & Noble Books. Brody, B. A. (1970). Readings in the philosophy of science. Englewood Cliffs, New Jersey: Prentice-Hill. Carnap, R. (1966). Three types of concepts in science. In: M. Gardner (Ed.), Rudolf Carnap: An introduction to the Philosophy of Science (pp.51-51). New York: Basic Books. Hempel, C. G. (1966). Philosophy of Natural Science. Englewood Cliffs, New Jersey: Prentice-Hall. HUNSBlog. (2006-2009). Four Laws of Biology. The Houston Urban Network for Science, Technology, Engineering and Mathematics. http://hunblog.typepad.com. ; Retrieved 09/02/17. Toulmin, S. (1977). Human understanding: The collective use and evolution of concepts. Princeton, New Jersey: Princeton University Press. Abimbola, I. O. (2013). Philosophy of Science for degree students. Ilorin: Author. Abimbola, I. O., & Omosewo, E. O. (2006). History of Science for degree Students. Ilorin: Butterfield, H. (1980). The Origins of Modern Science. London: Bell & Hyman Ltd. Crombie, A. C. (1959). Medieval and early Modern Science: XIII to XVII centuries, vol. 11. Garden City, New York: Doubleday. De Vancouleours, G. (1957). Discovery of the Universe. New York: Macmillan. Descartes, R. (1960). Discourse on Method and Meditations (L.J.Lafleur, Trans.). Indianapolis, Indiana: Bobbs-Merrill Educational Publishing Co. Fraser, C. G. (1948). The Story of Physics. New York; Reinhold Corporation. Hall, A. R. (1954). The Scientific Revolution, 1500-1800. Boston: Beacon Press. Hoyle, F. (1962). Astronomy. Garden City, New York: Doubleday. Jean, J. (1967). The Growth of Physical Science. Greenwich, Connecticut: Fawcett. Mason, S. F. (1962). A History of the Sciences.New York: Macmillan Company. Mendelsohn, E. (1982). Science, History of in: Grolier, Inc., The New Book of Knowledge, 17. Danbury, Connecticut: Grolier, Inc. Singer, C. (1959). A Short History of Scientific Ideas to 1960. New York: Oxford University Press. Taylor, F. S. (1963). A Short History of Science and Scientific Thought. New York: W.W. Norton & Co., Inc. Westfall, R. S. (1971). The Construction of Modern Science. New York: John Wiley. 18 Chapter Two PHILOSOPHY OF SCIENCE AND SCIENTIFC EXPLANATIONS Akanji, M.A. and Yakubu, M. T. Department of Biochemistry, University of Ilorin, Ilorin, Nigeria INTRODUCTION What is science? Is there a real difference between science and myth? Is science objective? Can science explain everything? Provision of answers to these questions provides a concise overview of the main themes of this chapter which is philosophy of science. OBJECTIVES At the end of the chapter, students are expected to: (i) account for the definitions of science; (ii) understand the concept of philosophy of science; (iii) describe the methods for studying philosophical science; (iv) give account of scientific explanation from the philosophical context; (v) account for the models used for scientific explanations with specific examples and their shortcomings; and (vi) explain the basis for the validity of scientific explanations and objectivity of observation in science. Science The word science is derived from the Latin word ‘scientia’ meaning knowledge. Science can be defined in various ways. These definitions include: the state or fact of knowing; knowledge or cognisance of something specified or implied; a branch or study which is concerned either with a connected body of demonstrated truths or with observed facts systematically classified and more or less colligated by being brought under general laws and which include trustworthy methods for the discovery of new truth within its own domain; an ordered body of knowledge or a search for explanations to natural objects and phenomena; Such knowledge is derived from the systematic study of nature and behaviour of materials of the physical universe based on observations, experimentations, measurements and the formation of laws to describe these facts. These observed facts can be systematically classified and brought under general principles. devotion of man to research or to the attainment of the kind of knowledge which establishes general laws governing a number of particular isolated facts. In common usage, the word science is applied to a wide variety of disciplines or intellectual activities which have certain features in common. Application of the term did not begin with any formal definition, rather, the various disciplines arose independently each in response to some particular need. It was then observed that some of these disciplines had enough traits in common to justify classifying them together as one of the sciences. Science probes into unknown and investigates established facts. The information that are thus obtained can be synthesised, classified as generalised and stated as norms, concepts, principles, theories and laws; an approach which has enabled man to establish the truth and appreciates his personal self and his environment better. 19 PHILOSOPHY OF SCIENCE Philosophy of science is concerned with the assumptions, foundations, methods and implications of science. In other words, it is concerned with the use and merit of science and which sometimes overlaps metaphysics (study of reality) and epistemology (study of theory of knowledge) by exploring whether scientific results are actually a study of truth. Philosophical science can simply be defined as critical discussion of the various developments in science. It may also be looked at from the exhibition of the working of philosophy in the scientific enterprise over the ages and its various effects on man’s destiny. For philosophy to rightly take its place in the sciences, it must make a very significant contribution to the advancement of the subject matter as a consequence of knowledge. Philosophical science is aimed at prodding scientists into an extremely healthy state of scepticism about many of the traditional foundations of their thinking. Studying the philosophical aspect of science will enable one to be accustomed with the development in science over the years; this will stimulate an active interest in the discipline. Philosophers of science therefore concern themselves with what science is all about; its goals, structure and its activities. Some philosophers of science also use contemporary results in science to reach conclusions about philosophy Philosophy of science has historically been met with mixed response from the scientific community. Though scientists often contribute to the field, many prominent scientists have felt that the practical effect on their work is limited as buttressed by a popular quote attributed to the physicist, Richard Feynman which states that "Philosophy of science is about as useful to scientists as ornithology is to birds." In contrast, some philosophers like Craig Callender have refuted this belief by pointing out that it is likely that ornithological knowledge would be of great benefit to birds, if and only when it is possessed. Furthermore, many philosophers of science have also considered problems that apply to particular sciences such as philosophy of biology, philosophy of chemistry etc. Philosophy of particular sciences In addition to addressing the general questions regarding science and induction, many philosophers of science are occupied with investigating philosophical or foundational problems in particular sciences. The late 20th and early 21st century witnessed an unprecedented rise in the number of practitioners of philosophy of a particular science such as philosophy of Biology, philosophy of Chemistry etc. Philosophy of Biology Philosophy of Biology deals with issues that focus on epistemology (study of theory of knowledge), metaphysics (study of reality) and ethics (study of norms of moral behaviour) contained in the biological and biomedical sciences. Although philosophers of science and philosophers in general like Aristotle, Renes Descartes, Leibin and Kant have long been interested in Biology, philosophy of Biology only emerged as an independent field of philosophy in the 1960s and 1970s. Philosophers of science began to pay increasing attention to developments in biology, from the rise of the modern synthesis in the 1930s and 1940s to the discovery of the structure of deoxyribonucleic acid (DNA) (a polynucleotide having a specific sequence of deoxyribonucleotide units and serving as the carrier of genetic information from the parents to the off-springs) in 1953 to more recent advances in genetic engineering (aspect of Biochemistry that deals with the manipulation of genes). Reduction of all life processes to biochemical reactions as well as the incorporation of psychology into a neuroscience also forms an important scope of philosophy of Biology. Research in current 20 philosophy of Biology is dominated by investigations about the foundations of evolutionary theory. Philosophy of Chemistry Philosophy of Chemistry is concerned with the methodology and the underlying assumptions of the science of Chemistry. This aspect is explored by philosophers, Chemists, and Philosopher-Chemist teams. Specific topics of interest which are normally addressed include the relationship between chemical concepts and reality where resonance structures are used in chemical explanations, and the reality of concepts such as nucleophiles and electrophiles. Others include whether chemistry studies atoms or reaction processes, symmetry in chemistry specifically the homo-chirality in biological molecules and whether quantum mechanics can offer explanation to all chemical phenomena. Philosophy of Mathematics Philosophy of Mathematics focuses on the philosophical assumptions, foundations, and implications of mathematics. Topics of concern include but are not limited to the sources of mathematical subject matter, what it means to refer to a mathematical object, character of a mathematical proposition, the relationship between logic and mathematics, the kinds of inquiry that play a role in mathematics, the objectives of mathematical inquiry, the source and nature of mathematical truth, the relationship between the abstract world of mathematics and the material universe. Others include what is a number, why does it make a sense to ask whether "1+1 = 2" is actually true and how can it be ascertained that a mathematical proof is correct. Philosophy of Physics Philosophy of Physics can be viewed as the study of diverse concerns which include the fundamental aspects of physics, philosophical questions concerning modern physics, the study and interaction of matter and energy. The main questions concerning the nature of space, and time, atoms and atomism as well as the interpretations of quantum mechanics, predictions of cosmology, foundations of statistical mechanics, causality, determinisms and the nature of physical laws are addressed under this concept. Philosophy of Psychology Philosophy of Psychology deals with issues relating to theoretical foundations of modern psychology. Some of these however are addressed from the epistemological perspectives of the methodology of psychological investigation; for example, the most appropriate methodology (mentalism, behaviourism or compromise) for Psychology, the reliability of self-reports as a data gathering procedure, the conclusions that can be drawn from the test of null hypothesis and the objective measurement of first-person experiences (emotions, desires, beliefs, etc.). Other concerns of philosophy of psychology include the philosophical questions about the nature of mind, brain, and cognition, and are perhaps more commonly thought of as part of cognitive science or philosophy of mind. Philosophy of psychology is a relatively young field, because psychology itself only became recognized as a discipline of its own in the late 1800s. METHODS USED IN STUDYING PHILOSOPHICAL SCIENCE Three different methods can be used to render science accessible to people and to actually make it an object for philosophical scrutiny. The methods are: - The Pedestrian method - The Critical method - Original Philosophical method 21 (a) The Pedestrian Method This method discusses topics in science. Such topics may include magnetism, electromagnetism, sub-atomic particles, enzymes, free radicals etc. In some cases, the contributions of notable scientists are mentioned alongside their discoveries. Examples of such discoveries include, linear propagation of light and weightlessness in space discovered by Sir Isaac Newton, electricity discovered by Michael Faraday, biological cells by Robert Hooke and Genetics by Gregory Mendel. In other words, anyone literate in the language of instruction would be competent to impact onto others on this aspect of knowledge. This method can therefore be described as barren and pedestrian. This method is of the view that even historians, linguists and artists can teach competently the philosophy of science. The bone of contention that just anyone can teach philosophy of science is aided and abetted by the slogan – “anything goes” syndrome. Unfortunately, this conception can no longer hold after centuries of the dismemberment of philosophy into independent disciplines, each with an object sphere of its own. (b) The Critical Method This involves taking up science and examining its fundamental assumptions and presuppositions, its competing theories, its method of inquiry and its relation or otherwise to other fields of study. A process which embraces this is known as meta science or meta scientific inquiries of the methodology of science. This method focuses more on the method and procedure and clarification of concepts. In this method, there are two classes of people namely a trained philosopher and a trained scientist that can disseminate this approach of critical method of philosophical science. What this may bore down to is that if what the entire philosopher does is to criticise the method, procedure, basic assumptions, presupposition and clarification of its terms, then the fundamental problem of a scientist combining his functions as a researcher with those of the philosopher as a critic, guide and guard will arise. (c) The Original Philosophical Method This method involves a trained philosopher injecting his apriori metaphysical, epistemological or ethical notions into science with the aim of uplifting its empirical content to the standard of it being adjudged the universal truth. In this regard, a philosopher is either metaphysising, epistemologising or ethicising science. In applying this method to render science accessible to people, the full meaning of the word philosophy, expresses in Greek, Philein Sophia which means “love of wisdom” must be put in mind. Wisdom is really needed to be able to explain what is true or false and to deal with facts and to judge experience in an uplifting and beneficial manner. Wisdom in its own sense can include making sense of our existence, our actions and of our destiny by a judicious balance of intuitive and discursive interpretations of our experience of being. In addition, the core areas of philosophy namely metaphysics, epistemology and ethics need to be considered as the watchwords when applying this method to render science accessible for people. SCIENTIFIC EXPLANATION The three cardinal aims of science are prediction, control, and explanation; but the greatest of these is explanation. Scientific explanation aims at understanding science. Philosophical Context The concept of scientific explanation is very important in philosophy of science because of several reasons: Most people and scientists intuitively believe that one of the goals of the science is to explain the phenomena in the world. Some people even believe that explanation is the main 22 goal of science. Whether philosophers accept this intuitive belief is not so important – just the fact that there is such belief is a good reason to analyse the concept of explanation and in particular scientific explanation. One further indication that such belief exists is that even an empiricist, who thinks that prediction of phenomena is the goal of science, still explains why they don’t accept explanation as goal of science. Scientific realists use the “inference of best explanation” (IBE) principle to solve the strong under-determination problem and this way to prove that science can create true knowledge even about non-observable (non-empirical) entities. The IBE principle, in short, says that between strongly empirically equivalent hypotheses, “the truth of the hypothesis which gives the best explanation of the phenomena should be inferred”. In addition to predicting future events, scientists more often than not use scientific theories to explain the events that occur regularly or have already occurred. Philosophers have investigated the criteria by which a scientific theory can be said to have successfully explained a phenomenon, as well as what gives a scientific theory credibility or explanatory power. Several models have been put forward to back up the explanatory power of scientific theories. MODELS FOR SCIENTIFIC EXPLANATION (a) Deductive-Nomological Model or D-N Account One early and influential theory of scientific explanation was put forward by Carl G. Hempel and Paul Oppenheim in 1948. Their model of explanation, Deductive-Nomological (D-N), says that a scientific explanation succeeds by subsuming a phenomenon under a general law. Hempel and Oppenheim (1948) proposed simply to describe what kind of things scientists tendered when they claimed to have an explanation, without asking whether such things were capable of providing “true understanding”. Since Hempel and Oppenheim (1948), the philosophy of scientific explanation has proceeded in this humble vein, seeming more like sociology of scientific practice than an inquiry into a set of transcendent norms. Scientific explanation as conceived by Hempel and Oppenheim (1948) can be viewed as a piece with prediction, requiring the same resources and giving a similar kind of satisfaction. The D-N Account of Hempel and Oppenheim (1948), is intended to capture the form of any deterministic scientific explanation of an individual event, such as the expansion of a particular metal bar when heated, the extinction of the dinosaurs, or the outbreak of the American Civil War. According to Hempel and Oppenheim (1948), such an explanation is always a deductive derivation of the occurrence of the event to be explained from a set of true propositions including at least one statement of a scientific law. Intuitively, the premises of a D-N explanation spell out the relevant initial, background, and other boundary conditions, together with the laws governing the behaviour of the system in which the explanandum (to expound observation related to the exploration of a new topic, technology, entity, idea or confounding concept in the on-going work against a state of boredom) occurred. Hempel and Oppenheim (1948) cite the following argument, for example, as a typical D-N explanation of the event of a thermometer’s mercury expanding when placed in hot water: The (cool) sample of mercury was placed in hot water, heating it. Mercury expands when heated, thus-The sample of mercury expanded. Because the law or laws that must be cited in a D-N explanation typically “cover” the pattern of behaviour of which the explanandum is an instance, the D-N account is sometimes referred to as the covering law account of explanation. 23 Many scientific explanations of events and other phenomena undoubtedly have the form proposed by the D-N account: they are logical derivations from laws and other information. Although ignored for a decade, this view was subjected to substantial criticism, resulting in several widely believed counter examples to the theory. Shortcomings of the D-N Account Three kinds of objections to the D-N account have been especially important for the subsequent development of the philosophy of explanation. These are: The first kind of objection, developed by Kyburg, Salmon, and others, points to the D-N theory’s inability to account for judgments of explanatory relevance. The paradigm is the following arguments, which satisfies all of the D-N account’s criteria for a good explanation of the event of a particular teaspoon of salt’s dissolving: The teaspoon of salt was hexed (meaning that certain hand gestures were made over the salt), The salt was placed in water, All hexed salt dissolves when placed in water, thus The salt dissolved. The explanation appears to attribute the salt’s dissolving in part to its being hexed, when in fact the hexing is irrelevant. The second important objection to the D-N account is the insufficient attention to the explanatory role of causal relations. For example, the height of a flagpole can be cited, along with the position of the sun and the law that light travels in straight lines, to explain the length of the flagpole’s shadow. The D-N account was able to explain by casting it in the form of a sound, law-involving argument for the height of the flagpole that cites, among other things, the length of the shadow. This consequence of the D-N account-that the height of a flagpole can be explained by the length of its shadow-seems obviously wrong, and it is wrong because a cause cannot be explained by its own effects. The third class of objection to the D-N account focuses on the requirements that every explanation cites a law, and that (except in probabilistic explanation) the law or laws be strong enough to entail, given appropriate boundary conditions, the explanandum. One way to develop the objection is to point to everyday explanations that cite the cause of an event as its explanation, without mentioning any covering law, as when you cite a patch of ice on the road as the cause of a motorcycle accident. More importantly for the study of explanation in science are varieties of explanation in which there is no prospect and no need for, either the entailment or the probabilification of the explanandum. Perhaps the best example of all is Darwinian explanation, in which a trait T of some species is explained by pointing to the way in which T enhanced, directly or indirectly, the reproductive prospects of its possessor. Attempting to fit Darwinian explanation into the D-N framework creates a host of problems, among which the most intractable is perhaps the following: for every trait that evolved because it benefited its possessors in some way, there are many other, equally valuable traits that did not evolve, perhaps because the right mutation did not occur, perhaps for more systematic reasons (for example, the trait’s evolution would have required a dramatic reconfiguration of the species’ developmental pathways). To have a D-N explanation of T, one would have to produce a deductive argument entailing that T, and none of the alternatives, evolved. One would have to be in a position, in other words, to show that T had to evolve. Not only does this seem close to impossible; more importantly, it seems unnecessary for understanding the appearance of T. 24 Inductive-Statistical Model or IS Account In addition to the D-N model, Hempel and Oppenheim offered other probabilistic explanation of events referred to as inductive-statistical or IS account. IS explanation is a law-involving argument giving good reason to expect that the explanandum event occurred. However, whereas a D-N explanation is a deductive argument entailing the explanandum, an IS explanation is an inductive argument conferring high probability on the explanandum. As with the D-N account of explanation, a number of objections to the IS account have exerted a strong influence on the subsequent development of the philosophical study of explanation. Versions of both the relevance and the causal objections apply to the IS account as well as to the D-N account. Shortcomings of the IS Account The important criticism of this model includes: There is too much to ask that explanations confer high probability on their explananda. A second objection to the IS account focuses on the requirement of maximal specificity. The requirement insists that all relevant background knowledge must be included in a probabilistic event explanation, but it does not require that relevant but unknown information be taken into account. However, Salmon attempted to provide an alternative model that will take care of the short comings of the models proposed by Hempel and Oppenheim (1948) by developing a statistical relevance model. Statistical Relevance Model In response to the D-N account’s relevance problem, Salmon suggests that the factors cited in an explanation must stand in a relation of statistical relevance to the explanandum. He does not intend this as a friendly amendment to Hempel and Oppenheim (1948) account, but rather as a radical re-conceptualisation of the nature of explanation: the function of an explanation, Salmon argues, is not to show that the explanandum was to be expected, but to describe factors-ideally, all the factors-statistically relevant to the occurrence of the explanandum. Statistical relevance is presented as an objective relation, that is, a relation holding independently of the explainer’s background knowledge or other context. Statistical relevance is a comparative concept. For example, to say that a factor A is statistically relevant to the occurrence of an event E is to say that the probability of E (or for the frequentist, of events of the same type as E) in the presence of A is greater than the probability of E in the absence of A. Thus, the determination of a relevance relation requires not only a reference class-a class of outcomes all of which occurred in the presence of A-but a contrast class, a class of outcomes all of which occurred in the absence of A. In addition to Salmon's model, others have suggested that explanation is primarily motivated by unifying disparate phenomena or primarily motivated by providing the causal or mechanical histories leading up to the phenomenon. BASIS FOR THE VALIDITY OF SCIENTIFIC EXPLANATIONS Empirical verification Science relies on evidence to validate its theories and models, and the predictions implied by those theories and models should be in agreement with observation. Unfortunately, one short 25 coming of observations is that it is dependent at times on the unaided human senses of sight, taste, touch and hearing. However, for this to be accepted by most scientists, several impartial, competent observers should agree on what is observed. Observations should be repeatable, for example, experiments that generate relevant observations can be (and, if important, usually will be) done again. Furthermore, predictions should be specific and scientists should be able to describe a possible observation that would falsify a theory or a model that implies the prediction. Nevertheless, while the basic concept of empirical verification is simple, in practice, there are difficulties as described in the following sections: Induction How is it that scientists can state, for example, that Newton's Third Law of Motion (to every action, there is equal and opposite forces) is universally true? After all, it is not possible to have tested every incidence of an action, and found a reaction. There have, of course, been several tests, and in each one a corresponding reaction has been found. But is it sure that future tests will continue to support this conclusion? One solution to this problem is to rely on the notion of induction. Inductive reasoning maintains that if a situation holds in all observed cases, then the situation holds in all cases. So, after completing a series of experiments that support the Third Law, and in the absence of any evidence to the contrary, one is justified to imply that the Law will hold in all cases. Although induction commonly works (e.g. almost no technology would be possible if induction were not regularly correct), explaining why this is so has been somewhat a herculean task. It is not easy to deduce, the usual process of moving logically from premise to conclusion, because there is no way of arguing in which two statements are used to prove that a third statement is true. Indeed, induction is sometimes mistaken. For example, the 17th century biologists observed many white swans (large graceful birds and none of other colours, but not all swans are white). Similarly, it is at least conceivable that an observation will be made tomorrow that shows an occasion in which an action is not accompanied by a reaction; the same is true of any scientific statement. One answer would be to conceive a different form of rational argument, one that does not rely on deduction. Deduction allows scientists to formulate a specific truth from a general truth: all crows are black; this is a crow; therefore this is black. Induction somehow allows scientist to formulate a general truth from some series of specific observations For example, this is a crow and it is black; that is a crow and it is black; no crow has been seen that is not black; therefore all crows are black. OBJECTIVITY OF OBSERVATIONS IN SCIENCE It is very important for science that the information about the surrounding world and the objects of study are as accurate and reliable as possible. For the sake of this, measurements which are the source of this information must be as objective as possible. Before the invention of tools like weights, meter rule, clock etc, the only source of information available to humans were their senses of vision, hearing, taste, tactile, sense of heat, sense of gravity, etc. Because human senses differ from person to person (due to wide variations in personal chemistry, deficiencies, inherited flaws, etc.) there were no objective measurements before the invention of these tools. The consequence of this was the lack of a rigorous science. 26 However, with the advent of exchange of goods, trades and agriculture, the need for such measurements, and science based on standardised units of measurement became imperative. To further abstract from unreliable human senses and make measurements more objective, science uses measuring devices such as spectrometers, voltmeters, interferometers, thermocouples, counters), and more recently, the computers. In most cases, the less the human involvement in the measuring process, the more accurate and reliable the scientific data is. Currently, most measurements are done by a variety of mechanical and electronic sensors directly linked to computers which further reduces the chance of human error/contamination of information. Another question about the objectivity of observations relates to the so called experimenters regress, problems identified from the sociology of scientific knowledge, the cognitive and social biases of the people that interpret the observations or experiments which unconsciously interpret and describe what they see in their own way. CONCLUSION Overall, the present chapter has made some attempt at several definitions of science with focus on knowledge. It has also addressed the concept of philosophy of science and scientific explanations adopting some models such as Deductive-Nomological (D-N), Inductive Statistical (IS) and Statistical Relevance (SR) with some specific examples. The basis for the validity of scientific explanations and objectivity of observation in science were also addressed. EVALUATION STRATEGIES Practice Questions 1. Scientia, which means knowledge is a A. Latin word B. Greek word C. Hebrew word D. Arabic word 2. One of these is not a method used in the study of philosophical science A. Analytical method B. Pedestrian method C. Critical method D. Original philosophical method 3. One of the most potent tools of the sciences for the discovery of new facts and more accurate understanding of existing facts is A. Explanation B. Exponential C. Experiment D. Exfoliation 4. Chemical balance, metre rule and spring balance are instruments of A. Measurement B. Marking C. Shaping D. Pinging 5. The scientist rejects authority as the ultimate basis for A. Trial B. Hypothesis 27 C. Truth D. Theory 6. The study of the theory of knowledge is referred to as A. Pedestrain B. Metaphysics C. Epistemology D. Biology 7. Ethics is the study of A. Moral Behaviour B. Ethnic Group C. Experience D. Reality 8. All the definitions of science revolve around A. Observation B. Experimentation C. Knowledge D. None of the above 9. Which of the following is not a model that can be for scientific explanation? A. IS B. BI C. DN D. SR 10. Which of the following is the most recent device? A. Spectrometer B. Galvanometer C. Computer D. Battery 28 REFERENCES Abimbola, I. O. (2006). Philosophy of science for degree students. Olatunbosun Publishers, Osogbo, Nigeria. Abimbola, I. O. and Omosewo, E. O. (2006). History of science for degree students. Oyinwola Printing Works, Ilorin, Nigeria. Burtt, E.A. (1980). The metaphysical foundation of modern science. Revised edition, The Humanities Press, New Jersey. (Revised edition). Godfrey-Smith P. (2009). Darwinian Populations and Natural Selection. Oxford: Oxford University Press. ^http://www.philostv.com/craig-callender-and-jonathan-schaffer/ http://plato.stanford.edu/entries/scientific-explanation/. Retrieved 2007-12-07. Hull D. (1969). What philosophy of biology is not, Journal of the History of Biology 2: 241- 268. Okasha S. (2006). Evolution and the Levels of Selection. Oxford: Oxford University Press. Oppenheim, P. (1948). Studies in the Logic of Explanation. Philosophy of Science15 (2): 135–175. Ruch, E. A. and Anyanwu, K. C. (1981). African Philosophy: an Introduction to the main philosophical trends in contemporary Africa. Catholic Book Agency. Rome Salmon, M., Earman, J., Glymour, C., Lenno, J. G., Machamer, P., McGuire, J. E., Norton, J. D., Salmon, W. C., Schaffner, K. F. (1992). Introduction to the Philosophy of Science. Prentice-Hall. Salmon, W. (1970). Statistical explanation. Reprinted in Statistical Explanation and Statistical Relevance, pp. 29–87. University of Pittsburgh Press, Pittsburgh. Salmon, W. (1971). Statistical Explanation and Statistical Relevance. Pittsburgh: University of Pittsburgh Press. Scriven, M. (1959). Explanation and prediction in evolutionary theory. Science 30:477–482. Unah, J. I. (1998). The general thrust of philosophical science. In: Philosophical Science. Unah J. (ed). Foresight Press. Lagos, Nigeria. pp 1-18. Woodward, J. (2003). “Scientific Explanation”. Stanford Encyclopedia of Philosophy. 29 Chapter Three CONCEPT OF MATTER Akoshile C.O1. and Abdus-Salam, N2 1 Department of Physics, University of Ilorin 2 Department of Chemistry, University of Ilorin INTRODUCTION This chapter discusses the concept of matter. Often, one keeps in the mind what he thinks matter is. It does not imply a universal definition. Concept is like an accepted norm or definition. So, while trying to understand matter, the adopted concept is what is perceived and accepted as matter. Interestingly, only two kinds of matter exist in the world. OBJECTIVES At the end of this chapter, students are expected to: (i) define matter; (ii) explain the scientific methods of studying matter; (iii) state some scientific propositions and laws; (iv) explain the kinetic theory of matter ; (v) analyse the relationship between kinetic energy, velocity, density, pressure, force; (vi) classify matter into states; (vii) Analyse the processes involved in change of state; (viii) classify matter into pure and mixture substances; (ix) define what an atom is and explain properties of sub atomic particles; and (x) define radioactive substance and describe its reactions; explain properties and applications of the radioactive particles What is Matter? Matter exists as a living and non-living entity. Living matter has the properties of respiration, growth, movement, metabolism (eating and excretion) and reproduction. Non-living matter does not exhibit the above properties. Growth in non-living matter only comes if there is an addition of the same or different matter by some processes to the matter. Matter is constituted. This means that matter is also made up of something else. Concept of Matter Attempt to develop the concept of matter involves many propositions and developing hypothesis. From such hypothesis, theory of matter emerges. The first step is to know the properties of matter. The simplified defin