Mechanical Properties of Cement Fiber Board with 5% NaOH-treated Banana Fiber PDF
Document Details
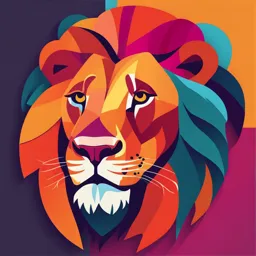
Uploaded by SuperGnome
Cebu Technological University
Fritzie Calunod, Chenee P. de los Santos, and Ivy L. Molinas
Tags
Summary
This study investigates the mechanical properties of cement fiber boards reinforced with banana fiber. The research examines the effects of alkali treatment on the fiber and varying fiber percentages. The study tested the material's properties under different conditions to determine suitable properties for materials engineering projects.
Full Transcript
1 Mechanical Properties of Cement Fiber Board with 5% NaOH-treated 2 Banana Fiber as Cellulose Reinforcing Fiber 3 by Fritzie Calunod, Chenee P. de los Santos, and Ivy L. Molinas 4 Biography: 5 Fritzie C. Calunod is a fourth-year student pursui...
1 Mechanical Properties of Cement Fiber Board with 5% NaOH-treated 2 Banana Fiber as Cellulose Reinforcing Fiber 3 by Fritzie Calunod, Chenee P. de los Santos, and Ivy L. Molinas 4 Biography: 5 Fritzie C. Calunod is a fourth-year student pursuing a Bachelor of Science in Civil 6 Engineering at Cebu Technological University-Main Campus located at M.J. Cuenco Ave., 7 Cor R. Palma St., Cebu, 6000. Fritzie was a Philippine Institute of Civil Engineers member 8 from 2022 to 2023. Her major interests are materials engineering and sustainable 9 infrastructure development, and she is determined to make a significant contribution to the 10 civil engineering profession. 11 Chenee P. de los Santos is an undergraduate student pursuing a Bachelor of Science in Civil 12 Engineering at Cebu Technological University-Main Campus. She is from Poblacion, Alcoy, 13 Cebu. From 2022 to 2023, she was a member of the Philippine Institute of Civil Engineers. 14 Her research interests focus on materials engineering, particularly in experimenting and 15 testing cement-based composites integrated with organic materials. 16 Ivy L. Molinas is a civil engineering intern at RCV Villamor Construction in Cogon West, 17 Carmen, Cebu. She is a 4th-year student pursuing a Bachelor of Science in Civil Engineering 18 at Cebu Technological University-Main Campus, located at M.J. Cuenco Ave, Cor R. Palm 19 Street, Cebu 6000. She was a member of the Philippine Institute of Civil Engineers from 20 2022 to 2023. Her research interests include using experimental and analytical methods to 21 predict the durability and service life of infrastructure materials such as concrete and cement 22 fiber board. 23 1 24 ABSTRACT 25 At present, the amount of organic waste, including banana stems, produced in the Philippines 26 is roughly 1.35 million tons. On the other hand, the construction industry demands innovation 27 in some of the construction materials, including cement fiber boards (CFB). The purpose of 28 this study is to address the increasing volume of organic waste, including banana stems, by 29 incorporating banana fibers into cement boards. The study examined the mechanical 30 properties of CFB reinforced with 3mm banana fibers treated with 5% NaOH, utilizing a 31 mixture ratio of 2:1 (sand:cement) and w/c ratio of 0.55 at varying fiber percentages of 0%, 32 1%, 4%, and 7%. The experimental procedures and testing adhered to the standards set by the 33 American Society of Testing and Materials (ASTM). The results indicate that alkali treatment 34 effectively mitigates water absorption, reduces moisture content, and enhances density and 35 tensile strength. However, the treatment's effect on flexural strength is complex and depends 36 on the fiber percentage. The best results for tensile strength parallel to the surface and 37 flexural strength at 10.45 MPa and 251.60 MPa, respectively, were observed at composites 38 with 4% and 1% banana fiber with 5% NaOH. Meanwhile, composites with 1% banana fiber 39 and 5% NaOH showed the best result for tensile strength perpendicular to the surface at 2.66 40 MPa. From the tested cement fiber boards, the cement fiber boards with 1% fiber 41 reinforcement showed the most promising results in both physical and mechanical properties 42 of the fiber cement board. 43 44 Keywords: Alkali Treatment, Banana Fiber, Cellulose Reinforcing Fiber, Cement Fiber 45 Board, Mechanical Properties 46 2 47 INTRODUCTION 48 The cement fiber board (CFB) plays a significant role in Philippine building practices, known 49 for its strength, durability, and resistance to fire, pests, and moisture. These boards are 50 commonly used in construction projects like structural walling, roofing, and cladding, 51 incorporating cement, reinforcing fibers, and other components for high strength and 52 durability. The incorporation of fiber in cementitious material composites as a reinforcement 53 has improvements in mechanical strength, compared to the mixtures with no fibers (Veigas et 54 al., 2022). This is because fibers stop cracks from starting and spreading. They avoid abrupt 55 rupture by slowing the growth of microcracks (Lilargem Rocha et al., 2022). 56 Synthetic fibers like carbon, glass, and polypropylene are traditionally used in reinforced 57 cement composites for industrial floors, roofs, and wall panels (Enfedaque et al., 2011). 58 However, petroleum byproducts like synthetic fiber are not biodegradable, take a long time to 59 decompose, and eventually pollute the environment. This poses challenges in terms of cost, 60 energy consumption, and negative environmental impact (Coutts, 2005), leading to a growing 61 interest of undergraduate researchers in sustainable alternatives like natural plant-based 62 cellulose fibers. 63 Cellulose fibers, derived from plants, are specifically recognized for their strong bond with 64 cementitious materials, improving adhesion and mechanical characteristics like flexural 65 strength and impact resistance (Sivaranjana & Arumugaprabu, 2021). The importance of 66 understanding the mechanical properties of natural plant fibers in composites is emphasized 67 in the research by Sethunarayanan et al. (n.d.), which examines factors like fiber types, 68 lengths, contents, surface treatments, and mix designs that impact these fibers' performance in 69 composites. 70 Table 1 includes some properties from the study of Karimah et al., (2021) comparing the 71 mechanical and physical properties of different types of natural plant fibers which can aid in 3 72 material selection such as tensile strength, modulus of elasticity, elongation, specific tensile 73 strength, specific modulus and toughness. The researchers have seen the potential of Banana 74 Pseudostem. Overall, banana fibers exhibit good tensile strength characteristics and can be 75 considered a strong natural fiber option for various applications. 76 The banana, scientifically known as Musa sinensis L., has been a staple for human 77 consumption for centuries and holds significant economic and nutritional value (Oyeyinka & 78 Afolayan, 2019). Being one of the most economically important fruit crops in the country, 79 bananas serve a multitude of purposes from their roots to fruits, as outlined in the Philippine 80 Banana Industry Road Map 2021. Despite their tropical nature and inability to thrive in 81 temperate regions, bananas are a vital commodity in global agriculture, with major producing 82 countries like India, China, Indonesia, and the Philippines. The Philippines, in particular, 83 stands out as one of the largest banana-producing nations, with a substantial volume of 84 bananas produced annually, reaching around 5.9 million metric tons in 2022 (FAO, 2023). 85 However, the extensive scale of banana production leads to a significant amount of waste, 86 with approximately 60% of banana biomass left unused after harvesting. This waste poses 87 environmental challenges if not managed effectively, contributing to greenhouse gas 88 emissions and pollution issues. Figure 1 shows the image of Banana while Figure 2 shows the 89 largest banana-producing countries in 2022 (in million metric tons). 90 The primary challenge lies in the utilization of banana pseudostems (BPS), which account for 91 a substantial portion of the banana plant mass (Green Banana Paper, 2017; Ma, 2015; Pérez, 92 1997). The BPS, a robust and durable component of the plant, contains bast fibers extracted 93 from the inner bark, known for their high tensile strength (Kiruthika, 2017). Banana fibers, 94 being renewable resources derived from plants, offer versatility in applications such as 95 cement-based composites. With their promising mechanical properties, banana fibers have 4 96 gained attention as reinforcing fibers for cement fiber boards, showcasing improved flexural 97 and tensile strength compared to traditional composites. 98 Fiber length and content are other crucial factor to consider in the manufacturing of cement 99 fiber boards. According to Abdalla et al. (2023), shorter fiber lengths offer several advantages 100 for cement fiber boards, including better dispersion within the cement matrix, improved 101 bonding and interlocking, and minimized risk of balling or clumping during the mixing 102 process. In the study of Daarol et al. (2023), blended fibers are used in which there is no 103 uniformity in fiber length. Highlighting the importance of fiber length uniformity, uniform 104 fiber lengths allow for better dispersion and distribution within the cement matrix, leading to 105 a more homogenous structure and improved mechanical properties. This uniformity enhances 106 the bridging effect, resisting crack propagation and resulting in higher composite strength 107 (Song et al., 2021). A study conducted by Kongkaew in 2016 on the mechanical properties of 108 banana and coconut-reinforced epoxy polymer matrix composites used fiber lengths of 3mm 109 with various weight proportions ranging from 3% to 30%. The research suggests that higher 110 fiber content in composite materials may result in void formation, leading to decreased tensile 111 strength and impact strength due to the brittleness caused by these voids. Canseco and Esper 112 (2020) investigated the use of Pineapple Leaf Fiber (PALF) in cement composites with fiber 113 percentages of 1%, 4%, and 7%, and a fiber length of 3mm with 1% fiber addition having the 114 best result in both the flexural and tensile strength. A study by Chandramouli et al. (2019) 115 investigated banana fiber reinforced concrete using fiber percentages of 1% to 6% with a 116 fiber length of 40mm. They observed that tensile strength increased gradually with up to 4% 117 banana fiber addition, reaching values of 5.94 MPa, 6.42 MPa, and 6.94 MPa after curing 118 periods of 28, 56, and 90 days, respectively. 119 Natural fibers are a potential alternative reinforcing fiber for cement boards. However, the 120 hydrophilic characteristic of cellulosic fibers has an impact on the fiber-matrix contact. 5 121 Furthermore, the reactive functional groups of the fiber are capped by waxy and pectin 122 substances, which prevent the fiber from interlocking with the matrix. The fibers' surface 123 must be modified with various chemical treatments to improve the efficiency of interfacial 124 bonding to achieve their maximum potential (Ali et al., 2016). Thus, proper fiber treatment 125 should be carried out. A study by Khan et al. (2019) uses NaOH treatment which roughens 126 the fiber surface and enhances the interfacial bonding of banana fibers with the cement matrix, 127 leading to improved mechanical properties. The utilization of a 5% NaOH concentration as 128 the optimal concentration from the studies (Wijianto, Ibnu & Adityarini, 2019, Nguyen, 2022) 129 is used. 130 With these existing studies, this study utilizes 3mm untreated and treated banana fibers as an 131 addition to cement at varying fiber percentages of 1%, 4%, and 7% to evaluate their effect on 132 the mechanical properties of CFBs. 133 The addition of banana fiber to cement composites has consistently demonstrated its 134 effectiveness in enhancing mechanical properties, particularly flexural and tensile strength. 135 Research by Ahsan Afraz and Majid Ali (2021) revealed that incorporating 2% banana fibers 136 into concrete significantly increased split and flexural strength compared to conventional 137 concrete after 28 days. Furthermore, the inclusion of 0.2% banana fiber improved tensile 138 strength, while modified fibers further enhanced flexural and split tensile strengths. This 139 research underscores the importance of sustainable construction practices and highlights the 140 potential of banana fiber reinforcement for developing eco-efficient and resilient concrete 141 structures. 142 Mir Firasath Ali et al. (2020) found that the maximum split tensile strength was achieved at 143 0.5% fiber content, reaching 4.65 N/mm2, a 9.1% increase compared to the reference 144 concrete without fiber. Similarly, the maximum flexural strength was attained at 0.5% fiber 6 145 content, reaching 6.3 N/mm2, representing a 16.64% improvement over the reference 146 concrete. 147 Minhas Shah and Majid Ali (2024) observed that the Splitting Tensile Strength (STS) values 148 for plain concrete (PC) and fiber-reinforced concrete (FRC) were 4.05 and 4.56 MPa, 149 respectively, indicating a positive correlation between higher banana fiber content and 150 increased STS. The STS value for FRC showed a 12.59% improvement, demonstrating the 151 enhanced splitting tensile strength achieved through banana fiber incorporation. 152 Suresh Kumar S. et al. (2019) investigated the effects of alkali treatment on banana fibers and 153 found that 3% NaOH-treated fibers exhibited the highest flexural strength compared to lower 154 concentrations. The study also revealed that 1% NaOH treatment increased average flexural 155 strength by 14.41, while 2% and 3% NaOH treatments resulted in further enhancements of 156 11.66 and 19.79, respectively. 157 These studies collectively demonstrate the significant potential of banana fiber as a 158 sustainable and effective reinforcement material for enhancing the mechanical properties of 159 cement composites, particularly in terms of flexural and tensile strength. The research 160 suggests that optimizing the use of banana fiber could lead to the development of more 161 durable, resilient, and environmentally friendly concrete structures. 162 163 RESEARCH SIGNIFICANCE 164 Plant-based cellulose fibers such as banana fiber offer eco-friendly reinforcement for cement 165 fiber boards, demonstrating effectiveness in structural walling, roofing, and cladding. 166 Moreover, NaOH treatment enhances the fibers' strength. However, there is limited literature 167 on the mechanical properties of cement fiber boards with NaOH-treated banana fibers. This 168 study aims to address this gap by investigating the enhancement of cement fiber boards 169 (CFBs) with banana fibers, thereby promoting environmental sustainability and advancing 7 170 construction material innovation. The research is significant for potentially revolutionizing 171 sustainable construction practices, reducing carbon footprints, and fostering eco-friendly 172 alternatives in the industry. 173 174 EXPERIMENTAL INVESTIGATION 175 The study's methodological flow, covering material acquisition and preparation, mixing 176 proportions, constructing the CFB, conducting tests, and analyzing data, were outlined in 177 Figure 3. 178 Acquiring and Preparation of Materials 179 Raw banana pseudostems sourced from Alcoy, Cebu, Philippines, were carefully stored for 180 transportation and quality preservation. Ordinary Portland Cement Type I conforming to 181 ASTM C150, sand, and potable water were procured for the study. The banana pseudostem 182 samples underwent screening, leaf removal, and extraction of fibers from the 11 outer leaf 183 sheaths. The sand met ASTM C33 standards, with particle size determined using wire-mesh 184 sieves. Manual extraction of banana pseudostem fibers involved hand scraping on a wooden 185 plank, followed by washing and sun-drying for three days. 186 Alkaline (NaOH) treatment 187 The banana fibers underwent a chemical treatment using a solution of sodium hydroxide 188 (NaOH). The treatment involved immersing the fibers in a 5% NaOH solution, maintaining a 189 liquor ratio of 20:1, for a duration of 6 hours at room temperature. Subsequently, the fibers 190 were thoroughly washed to eliminate any remaining chemicals or traces of NaOH. To ensure 191 complete removal of moisture, the fibers were oven dried at 80°C until a constant mass was 192 achieved. Following this, the fibers, now known as BP Fiber, were cut into lengths of 3 mm. 193 Mix Ratio and Proportion 8 194 The study employed various design mix ratios (w/w) for the fiber cement board samples, 195 adapting the design mix proportion of 2:1 (sand:cement) and a water-cement ratio of 0.55. 196 Different percentages of banana pseudo-stem fiber (1%, 4%, and 7% w/w cement) were 197 added based on the same study. A control group with 0% fiber was included for comparison. 198 A mix proportioning table (Table 2) was prepared to determine the amount of materials 199 required, including sand, cement, and BPF (Banana Pseudostem Fiber). This table served as a 200 guide for accurately measuring the quantities of each component by weight during the 201 preparation and mixing of each CFB (Cement Fiber Board) sample. 202 Fabrication and Curing of CFB 203 The entire process of manually producing the Cement Fiber Boards (CFB) was conducted. 204 Figure 4 shows the distribution of the Number of CFB for 28 Curing Days per Fiber 205 Percentage under 23±2°C. Figures 5 and 6 displayed the CFB dimensions conforming to 206 ASTM C1186, while Figures 7 and 8 adhered to ASTM C 209 – 98. 12 CFBs were fabricated 207 per mix, with an average duration of 5 minutes from mixing to molding. The filled molds 208 were left undisturbed on forming boards for 24 hours. Upon completion of CFB fabrication, 209 they were removed from the forms and transferred to the curing tanks. A total of 84 210 specimens were manufactured. 211 212 ANALYTICAL INVESTIGATION 213 Mechanical Tests 214 The evaluation of the mechanical properties of the Cement Fiber Board (CFB) with varying 215 mixtures of Banana pseudo-stem fiber is essential to ensure its compliance with the ASTM 216 Standards, specifically ASTM C1186 - Standard Specification for Flat Fiber-Cement Sheets 217 and ASTM C 209 – 98: Standard Test Methods for Cellulosic Fiber Insulating Board. To 218 assess the quality and functionality of the CFB, the researchers will conduct a series of 9 219 mechanical property tests. These tests will encompass the absorption test, measurement of 220 moisture content, assessment of density, and evaluation of flexural strength and tensile 221 strength parallel and perpendicular to the surface. By performing these tests, the researchers 222 will gain insights into the performance and suitability of the CFB for its intended application. 223 The equations below will be utilized. Treatment of data using Two-way ANOVA is used. − 224 , % = 100 (1) − 225 , % = 100 (2) 226 ( ), 3 = − (3) 3 227 ℎ, = 2 2 (4) 228 ℎ, = (5) 229 230 EXPERIMENTAL RESULTS AND DISCUSSION 231 Water Absorption 232 Figure 9 illustrates the water absorption behavior of different composite materials. Test 233 results suggested that with fiber addition, there is a high water absorption (WA). The fiber 234 addition in CFB increases the WA of treated and untreated samples compared to the control 235 sample, which has an absorption rate of 11.8%. There is a relationship between fiber 236 percentage and water absorption in composite materials, demonstrating a notable increase in 237 water uptake as the fiber percentage increases. The WA increases due to the addition of 238 banana fibers. These natural fibers absorb more water, resulting in higher WA than the 239 sample with 0% added fiber content. These results are similar to the study of Daarol et al., in 240 2023 about the properties of fiber cement board affected by AFPLF (abaca fiber and 241 pineapple leaf fiber). The effect of treated and untreated CFBs was also depicted with treated 242 fibers showing lower water uptake across different fiber percentages. WAs for CFB untreated 10 243 are 13.18%, 13.19%, and 14.03% for fiber addition of 1%, 4%, and 7% respectively, and for 244 CFB treated are 13.18%, 13.19%, and 14.03% for fiber addition of 1%, 4%, 7% respectively. 245 The data underscores the efficacy of treatment in mitigating water absorption, as treated 246 composites display lower water uptake levels. This is because untreated fiber has a high 247 percentage of hydrophilic lignin, which makes it hydrophilic and causes it to absorb a lot of 248 water when immersed in it (Oun et al., 2022). However, treated fibers are less likely to absorb 249 water which is similar to the effect of alkali treatment on water absorption of single cellulosic 250 abaca fiber (Punyamurthy et al., 2012) due to the removal of lignin and hemicellulose during 251 the NaOH treatment (Ronald Aseer et al. 2013). The relationship between treated, and 252 untreated CFBs and their varying percentages is because depending on the fiber weight ratio, 253 alkali-treated fiber composites absorb less water than untreated composites (Reddy et al., 254 2020). 255 Moisture Content 256 Test results for moisture content in Figure 10 show a relationship between how varying fiber 257 percentage and treated fibers affect the moisture content of the specimens. In untreated CFBs, 258 the moisture contents are 11.72%, 12.84%, and 14.53% for CFB UT-1, CFB UT-4, and CFB 259 UT-7, respectively. In treated CFBs, the moisture contents are 10.28%, 12.78%, and 11.42% 260 for CFB T-1, CFB T-4, and CFB T-7, respectively. The researchers observed that as the fiber 261 percentage increases, the moisture content of the sample also increases. Meanwhile, the 262 moisture content of the CFB with 0% banana fiber content is 10.98% which is lower than the 263 moisture content of CFB with 1% untreated fiber while higher than CFB with 1% treated 264 banana fiber. In the specimens observed, untreated banana fibers have more moisture content 265 compared to specimens with no fiber content and treated fibers. This is due to the hydrophilic 266 characteristic of untreated fiber, which causes it to absorb a significant quantity of moisture 267 when submerged in water or exposed to excessively humid conditions (Oun et al., 2022). 11 268 Treated fibers on the other hand less likely to absorb moisture which is similar to the effect of 269 alkali treatment on water absorption of single cellulosic abaca fiber (Punyamurthy et al., 270 2012). 271 Density 272 The test result for density in Figure 11 shows a relationship between how different 273 composites affect their behavior. The addition of fiber has shown a decrease in the density of 274 the composites. Compared to the control having a density of 1884.28 kg/m3, the density for 275 composites with the addition of untreated and treated fiber decreases to 1679.12 kg/m3 and 276 1812.76 kg/m3 respectively. Varying fiber percentages and treated fibers affect the density of 277 the specimens. This implies that in both treated and untreated CFBs, the fiber percentage is 278 inversely proportional to its density. As fiber percentage increases, its density decreases. 279 These results are similar to the study of Daarol et al., in 2023 about the properties of fiber 280 cement board affected by AFPLF (abaca fiber and pineapple leaf fiber). As the fiber 281 percentage increases, there may be a corresponding decrease in the amount of cement in the 282 composite mix. Since cement is denser than most fibers, a higher fiber volume fraction with 283 less cement content can contribute to a lower overall density of the composite. Treatment of 284 the fiber also affects the density of the composite. Density for 1%, 4%, and 7% fiber are 285 1804.80 kg/m3, 1762.84 kg/m3, and 1679.12 kg/m3 respectively for CFB untreated and 286 1846.07 kg/m3, 1837.86 kg/m3, and 1812.76 kg/m3 for CFB treated. This data implies that 287 untreated CFBs are less dense compared to CFBs with treated fibers. Treatment roughens the 288 fiber's surface which enhances adhesion to the cement matrix creating stronger bonds with 289 the cement. This improved adhesion results in a more compact composite and fewer voids 290 leading to higher density. Alkaline treatment also increases the density of fibers by removing 291 noncellulosic components (hemicellulose and lignin), which are less dense (Bekele et al., 292 2022). This causes a decrease in the volume fraction of the fibers making the density of fibers 12 293 increase. Cement boards with fibers are less dense compared to those without fibers. The 294 addition of fibers makes the boards less dense making them a light weight material suitable 295 for construction. 296 Flexural Strength 297 The comparison of flexural strength between untreated and treated samples at varying fiber 298 percentages is shown in Figure 12. At 0% fiber, the treatment had a slight negative effect on 299 strength, with the untreated sample showing 244.93 MPa MOR. Increasing the fiber to 1% 300 resulted in enhanced strength for both untreated and treated samples, with the untreated 301 sample reaching 356.40 MPa and the treated sample averaging 251.60 MPa. However, the 302 untreated sample maintained higher strength, indicating limited effectiveness of the treatment. 303 The untreated sample increased at 4% fiber, reaching 356.40 MPa, while treated samples 304 significantly decreased to 112.66 MPa, showing a negative impact of the treatment. At 7% 305 fiber, the treated sample outperformed the untreated with 248.73 MPa, surpassing the latter at 306 this level. In summary, the treatment's impact varied with fiber content: lower percentages 307 favored untreated samples, moderate fiber content showed a decline in treated sample 308 strength, and at 7%, the treatment showed a beneficial effect. The treatment process 309 especially the duration adapted from a polymer matrix may not apply to cement composites 310 in terms of its flexural strength. Notably, the flexural strength results still met ASTM C1185 311 requirements. Increasing banana fiber content enhances concrete flexural strength (Afraz & 312 Ali, 2021), similar to the positive effect observed with abaca fibers in cement panels (Iqbal et 313 al., 2022). Additionally, Panchore et al. (2018) found that banana fibers significantly 314 improved various engineering properties of concrete, including flexural strength. 315 Furthermore, the treated data by two-way ANOVA shown in Table 4 reflected that there is no 316 significant difference between the interaction of treated and untreated fibers and fiber 317 percentage on the flexural strength of the CFB. 13 318 Tensile Strength 319 Figure 13 compares the tensile strength parallel to the surface of treated and untreated 320 samples at various percentages of fiber (0%, 1%, 4%, and 7%). Tensile strength trends for 321 both treated and untreated samples are similar at for control (0%), averaging 3.1 MPa (449.62 322 psi). Tensile strength increases for both the treated and untreated samples at 1% fiber content, 323 with the treated sample significantly exceeding the untreated one. This indicates that 324 treatment, at low fiber concentrations, is effective. The treated sample's tensile strength 325 significantly increased to 10.45 MPa (1515.64 psi) at a 4% fiber concentration, compared to 326 6.47 MPa (938.39 psi) for the untreated sample. This suggests that treatment at 4% fiber 327 content significantly improved the specimen's performance. When compared to lower fiber 328 content, both the treated and untreated samples' tensile strength decreases at 7% 329 concentration, with the treated sample performing better. According to the results, treatment 330 improves tensile strength, especially at fiber contents of 1% and 4%, with 4% being the 331 optimal. When compared to the 4% fiber content, the treated sample exhibits less 332 improvement in tensile strength, however maintaining greater tensile strength than the 333 untreated sample at 7%. 334 Figure 14 shows the tensile strength comparison between the untreated and treated materials 335 at varying fiber concentrations (0%, 1%, 4%, and 7%) perpendicular to the surface. 336 Specimens containing fiber show better tensile strength than the control (0%). The control 337 specimen has a strength of 1.31 Mpa. The maximum strength of CFB untreated is 1.72 MPa, 338 while the treated sample performs better with maximum strength at 2.66 MPa. In each fiber 339 percentage, treated CFB exceeds the strength of untreated CFB. Both treated and untreated 340 CFBs' maximum strength was obtained at 1% fiber content. At 4% fiber content, the tensile 341 strengths of the untreated and treated specimens are 1.27 MPa and 1.53 MPa respectively. 342 Strength at 4% fiber content of 1.13 MPa and 1.48 MPa is obtained in untreated and treated 14 343 respectively. Even at 7% concentration, the treated sample maintains higher tensile strength 344 at 1.48 MPa compared to the untreated sample at 1.13 MPa, indicating the treatment's 345 effectiveness at higher fiber content. Overall, the data suggests that the treatment significantly 346 improves tensile strength perpendicular to the surface, especially at lower fiber contents (1%), 347 with the most notable enhancement observed at this concentration. It is observed that as fiber 348 percentage increases, there is a decrease in tensile strength perpendicular to the surface. 349 Despite a decrease in strength at higher fiber contents (4% and 7%), the treated samples still 350 outperform the untreated ones, highlighting the treatment's efficacy, particularly at lower 351 fiber content. 352 Results for tensile strength both perpendicular and parallel to the surface suggest that the 353 treatment helps maintain higher tensile strength even at higher concentrations. The reason for 354 this is, that alkaline treatment for bananas removes hemicellulose and other non-cellulosic 355 substances from the fiber surface thus, improving fiber-matrix adhesion and tensile strength 356 (Komal et al., 2018). The effect of banana fiber in this study is similar to the study of 357 Chandramouli, K. et al. in 2019 in the tensile strength of concrete has increased gradually up 358 to 4% addition of banana fiber and has shown a gradual decrease in the tensile strength 359 beyond that percentage. The addition of banana fibers significantly improved many of the 360 engineering properties of the concrete notably tensile strength. Similar to the concrete, the 361 banana fibers dispersed throughout the cement mixture, provide additional support and 362 resistance to tensile forces, making the board less prone to cracking or breaking under tension 363 in the concrete (Afraz & Ali, 2021). It is also observed that there is a decrease in strength for 364 tensile parallel and perpendicular at 7%. This is because, although fibers have helped in 365 strengthening the CFBs, there is a limitation on its content making the addition of 7% fiber 366 reduces its strength. The study of Kongkaew in 2019 suggests that higher fiber content in 367 composite materials may result in void formation, leading to decreased tensile strength and 15 368 impact strength due to the brittleness caused by these voids. As fiber fiber content increases, 369 there may be a difficulty in the dispersion of fiber or balling or clumping during the mixing 370 process. 371 Furthermore, the treated data by two-way ANOVA shown in Table 4 reflected that there is no 372 significant difference between the interaction of treated and untreated fibers and fiber 373 percentage on the tensile strength parallel to the surface of the CFB while there is a 374 significant difference between the interaction of treated and untreated fibers and fiber 375 percentage on the tensile strength perpendicular to the surface of the CFB. It indicates that the 376 combined effect of both the fiber treatment (treated vs. untreated) and the fiber percentage 377 has a notable impact on the tensile strength perpendicular to the surface of the composite 378 material. After conducting a two-way ANOVA (Analysis of Variance) shown in Table 3, t- 379 test is conducted to validate ANOVA analysis and make detailed comparisons between 380 multiple groups or conditions to determine where significant differences lie. Only the 381 inclusion of 1% treated fibers significantly influences the tensile strength perpendicular to the 382 surface of CFB as shown in Table 5. 383 384 FURTHER RESEARCH 385 For a more thorough investigation, it is advisable to consider the following suggestions to 386 enhance the breadth of research findings. Incorporating these recommendations can lead to a 387 more comprehensive and insightful analysis. 388 1. To enhance the accuracy of the results and identify outliers effectively, it is 389 advisable to increase the number of specimens taken for analysis. By expanding 390 the sample size, it is easier to pinpoint and analyze any outliers present in the data. 391 Also, consider investigating the effect of varying time duration for NaOH 392 treatment of banana fibers in CFBs. 16 393 2. To achieve massive production of 3mm fiber cut, it is advisable to use specialized 394 machine for cutting. 395 3. To determine the sustainability of the sample, it is recommended to conduct long- 396 term assessments of the boards to evaluate their performance over time and 397 understand any potential long-term effects. 398 399 CONCLUSIONS 400 Based on the results of this experimental investigation under tidal environment, the following 401 conclusions are drawn: 402 1. Treating banana fibers enhances some of the mechanical properties of CFBs 403 compared to untreated fibers. Additionally, the mechanical properties of CFB 404 improve with increasing banana fiber percentage up to 4%. 405 2. The water absorption and moisture content properties of Cement Fiber Boards 406 (CFB) are influenced by the type of banana fiber used and the percentage of 407 banana fiber content. Treating banana fibers with 5% NaOH tends to reduce water 408 absorption compared to untreated fibers, indicating improved water resistance in 409 CFB. Increasing fiber percentage results higher water absorption and moisture 410 content. 411 3. Adding fibers decreases the CFB’s density, making it an excellent material in 412 construction for being lightweight. The varying percentages’ effect on density is 413 that as fiber content increases, there is a decrease in its density. 414 4. The mechanical properties of CFB improve with increasing banana fiber 415 percentage up to 4%, showing enhanced tensile and flexural strength. However, at 416 7% fiber content, there is a decline in mechanical properties, indicating an optimal 417 fiber percentage for improving the performance of CFB. 17 418 5. Results for flexural strength of all specimens passed the flexural strength 419 requirement of ASTM C1186. These specimens can be applicable for the use of 420 roofing, cladding and walling. Thus, the optimum flexural strength is attained at 421 4% untreated fiber content at 356.40 MPa. 422 6. Optimum tensile strength of this study is at 2.66 MPa (1% ) and 10.45 MPa (4%) 423 treated fibers for perpendicular and parallel to the surface respectively. After the 424 treatment of data using ANOVA, it is found that there is a significant difference of 425 untreated and treated banana fibers and varying fiber percentages only on the 426 tensile strength perpendicular to the surface at 1% treated fiber at 2.66 MPa. 427 7. Increased fiber content in the board creates more air voids, which lowers density, 428 increases moisture content and water absorption, and beyond the optimum fiber 429 content, resulting in decreased strength and workability. Overall, treating banana 430 fibers and optimizing the fiber percentage can significantly enhance the 431 mechanical properties of CFB. CFB T-1 yielded the most promising results out of 432 all the tested samples. 433 434 ACKNOWLEDGMENTS 435 The planning and completion of this work were made possible by the participation, 436 encouragement, and dedication of many people. As a result, the authors would like to thank 437 everyone who has helped them make this a reality, whether directly or indirectly. To their 438 thesis adviser, Dr. Hana Astrid Canseco-Tunacao, the authors would like to express their 439 deepest gratitude for reading, correcting, and guiding them through helpful comments, 440 recommendations, and involvement in the learning process of their undergraduate thesis. The 441 authors would like to thank the Department of Public Works and Highway-Cebu City 442 Engineering Department, as well as the University of San Carlos College of Engineering, for 18 443 assisting them in testing the samples for this thesis work. To the authors’ families, they would 444 like to thank you for your financial support and encouragement to complete this thesis work. 445 446 447 448 449 450 451 452 453 454 455 456 457 458 459 460 461 462 463 464 465 466 467 19 468 NOTATION: 469 A = Cross-sectional Area 470 B = Saturated weight, lb (kg) 471 b = Width of specimen, mm 472 CFB – cement fiber board 473 d = Thickness of specimen, mm 474 F = Final weight of specimen, lb (kg) 475 L = Length of span, mm 476 P = Maximum load, N 477 S = Suspended weight, lb (kg) 478 T – Treated 479 UT – Untreated 480 W = Initial weight of specimen, lb (kg) 481 W = Dry weight of specimen, lb (kg) 482 WD = dry weight of specimen (kg) 483 WS = saturated weight of specimen (kg) 484 485 20 486 REFERENCES 487 Abdalla, J. A., Hawileh, R. A.,A. Bahurudeen, G Jyothsna, A. Sofi, S. Vigneshwaran, 488 & Blessen Skariah Thomas. (2023) A comprehensive review on the use of natural 489 fibers in cement/geopolymer concrete: A step towards sustainability. Case Studies in 490 Construction Materials, 19, e02244–e02244. 491 https://doi.org/10.1016/j.cscm.2023.e02244 492 Afraz, A., & Ali, M. (2021). Effect of Banana Fiber on Flexural Properties of Fiber 493 Reinforced Concrete for Sustainable Construction. The 1st International Conference 494 on Energy, Power and Environment. https://doi.org/10.3390/engproc2021012063 495 ASTM C1185-08 (Reapproved 2012), Standard Test Methods for Sampling and 496 Testing Non-Asbestos Fiber- Cement Flat Sheet, Roofing and Siding Shingles, and 497 Clapboards 498 ASTM C1186-08 (2012): Standard Specification for Flat Fiber-Cement Sheets 499 ASTM C150: Standard Specification for Portland Cement. 500 ASTM C209 - Standard Test Methods for Cellulosic Fiber Insulating Board 501 ASTM C33: Standard Specification for Concrete Aggregates 502 ASTM C270 (Standard Specification for Mortar for Unit Masonry) 503 Bekele, A. E., Lemu, H. G., & Jiru, M. G. (2022). Experimental study of physical, 504 chemical and mechanical properties of enset and sisal fibers. Polymer Testing, 106, 505 107453. https://doi.org/10.1016/j.polymertesting.2021.107453 506 Karimah, A., Ridho, M. R., Munawar, S. S., Adi, D. S., Ismadi, Damayanti, R., 507 Subiyanto, B., Fatriasari, W., & Fudholi, A. (2021). A review on natural fibers for 508 development of eco-friendly bio-composite: characteristics, and utilizations. Journal 509 of Materials Research and Technology, 13, 2442–2458. 510 https://doi.org/10.1016/j.jmrt.2021.06.014 21 511 Chandramouli, K. & Narayanan, Pannirselvam & Pardhu, D.V.V. & Anitha, V.. 512 (2019). Experimental investigation on banana fibre reinforced concrete with 513 conventional concrete. International Journal of Recent Technology and Engineering. 7. 514 874-876. 515 Daarol, M., Pepito, M. J., Polancos, R. J., & Sambong, R. F. (2023). Properties of 516 Fiber Cement Board Affected by AFPLF (Abaca Fiber and Pineapple Leaf Fiber). 517 E3S Web of Conferences, 405, 04004. https://doi.org/10.1051/e3sconf/202340504004 518 Esper, C. D. H., & Canseco, H. A. R. (2020). Influence of Alkali Treatment and Fiber 519 Content on Mechanical Properties of Pineapple Leaf Fiber (PALF)-Reinforced 520 Cement Based Composites via Full Factorial Design. Materials Science Forum, 1005, 521 65–75. https://doi.org/10.4028/www.scientific.net/msf.1005.65 522 Elbehiry, A., Elnawawy, O., Kassem, M., Zaher, A., Uddin, N., & Mostafa, M. (2020). 523 Performance of concrete beams reinforced using banana fiber bars. Case Studies in 524 Construction Materials, 13, e00361. https://doi.org/10.1016/j.cscm.2020.e00361 525 FAO. (2023, March 24). Crops and Livestock Products. Food and Agriculture 526 Organization of the United Nations. https://www.fao.org/faostat/en/#data/QCL 527 Sethunarayanan, R., Chockalingam, S., & Ramanathan, R. (n.d.). Natural Fiber 528 Reinforced Concrete. https://onlinepubs.trb.org/Onlinepubs/trr/1989/1226/1226- 529 008.pdf 530 Khan, M. W., Rahamathbaba, S., Mateen, M., Shankar, D. R., & Hussain, M. M. 531 (2019, February 1). Effect of NaOH treatment on mechanical strength of 532 banana/epoxy laminates. Polymers From Renewable 533 Resources.https://doi.org/10.1177/2041247919863626 534 Kongkaew, P. (2016). MECHANICAL PROPERTIES OF BANANA AND 535 COCONUT FIBERS REINFORCED EPOXY POLYMER MATRIX 22 536 COMPOSITES. https://www.worldresearchlibrary.org/up_proc/pdf/165- 537 145439339466-71.pdf 538 Lilargem Rocha, D., Tambara Júnior, L. U. D., Marvila, M. T., Pereira, E. C., Souza, 539 D., & de Azevedo, A. R. G. (2022). A Review of the Use of Natural Fibers in Cement 540 Composites: Concepts, Applications and Brazilian History. Polymers, 14(10), 2043. 541 https://doi.org/10.3390/polym14102043 542 Oyeyinka, B. O., & Afolayan, A. J. (2019, December 12). Comparative Evaluation of 543 the Nutritive, Mineral, and Antinutritive Composition of Musa sinensis L. (Banana) 544 and Musa paradisiaca L. (Plantain) Fruit Compartments. Plants. 545 https://doi.org/10.3390/plants8120598 546 Oun, A., Manalo, A., Alajarmeh, O., Abousnina, R., & Gerdes, A. (2022). Long-Term 547 Water Absorption of Hybrid Flax Fibre-Reinforced Epoxy Composites with Graphene 548 and Its Influence on Mechanical Properties. Polymers, 14(17), 3679. 549 https://doi.org/10.3390/polym14173679 550 Punyamurthy, Ramadevi & Dhanalakshmi, Dr & Chikkol Venkateshappa, Srinivasa 551 & Bennehalli, Basavaraju. (2012). Effect of alkali treatment on water absorption of 552 single cellulosic abaca fiber. BioResources. 7. 3515-3524. 10.15376/biores.7.3.3515- 553 3524 554 Reddy, B. M., Reddy, R. M., Reddy, B. Chandra. M., Reddy, P. V., Rao, H. R., & 555 Reddy, Y. V. M. (2020). The effect of granite powder on mechanical, structural and 556 water absorption characteristics of alkali treated cordia dichotoma fiber reinforced 557 polyester composite. Polymer Testing, 91, 106782. 558 https://doi.org/10.1016/j.polymertesting.2020.106782) 559 Ronald Aseer, J., K. Sankaranarayanasamy, P. Jayabalan, R. Natarajan, and K. Priya 560 Dasan. 2013. “Morphological, Physical, and Thermal Properties of Chemically 23 561 Treated Banana Fiber.” Journal of Natural Fibers 10 (4): 365–380. 562 https://doi.org/10.1080/15440478.2013.824848. 563 Sivaranjana, P., & Arumugaprabu, V. (2021, January 22). A brief review on 564 mechanical and thermal properties of banana fiber-based hybrid composites. SN 565 Applied Sciences. https://doi.org/10.1007/s42452-021-04216-0 566 Song, H., Liu, J., He, K., & Ahmad, W. (2021). A comprehensive overview of jute 567 fiber reinforced cementitious composites. Case Studies in Construction Materials, 568 15,e00724. https://doi.org/10.1016/j.cscm.2021.e00724 569 Veigas, M. G., Najimi, M., & Shafei, B. (2022). Cementitious composites made with 570 natural fibers: Investigation of uncoated and coated sisal fibers. Case Studies in 571 Construction Materials, 16, e00788. https://doi.org/10.1016/j.cscm.2021.e00788 572 573 574 575 576 577 578 579 580 581 582 583 584 24 585 TABLES AND FIGURES 586 List of Tables: 587 Table 1 - Physical and Mechanical Properties of Natural Fibers 588 Table 2 - Mix Proportion for fiber cement boards (12 specimen per batch) 589 Table 3 – Result of Analysis of Variance (ANOVA) 590 Table 4 – Result of T-test Tensile Perpendicular (TREATMENT) 591 Table 5 - Result of T-test Tensile Perpendicular (TREATED – FIBER CONTENT) 592 593 List of Figures: 594 Fig. 1 - Banana 595 Fig. 2 - Largest banana-producing countries 2022 (in million metric tons) 596 Fig. 3 - Methodology Flowchart 597 Fig. 4 - Breakdown of Number of CFB for 28 Curing Days per Fiber Percentage 598 Fig. 5 – CFB Dimension in mm for Absorption Test 599 Fig. 6 - CFB Dimension in mm for Flexural Test 600 Fig. 7 - CFB Dimension in mm for Tensile Test Parallel to the Surface 601 Fig. 8 - CFB Dimension in mm for Tensile Test Perpendicular to the Surface 602 Fig. 9 – Water Absorption (WA) & Moisture Content (MC) of CFB 603 Fig. 10 – Density of CFB 604 Fig. 11 – Flexural Strength of CFB 605 Fig. 12 – Tensile Strength Parallel to the Surface of CFB 606 Fig. 13 – Sample Failure of Flexural Test UT-7 25 607 Table 1– Physical and Mechanical Properties of Natural Fibers Type of fiber Average Density Tensile Modulus of Elasticity Elongation Specific Specific Toughness diameter (g/cm3) Strength (MPa) (GPa) (%) Tensile Modulus (MPa) of fiber Strength (GPa) (MPa) Abaca 122 0.83-1.3 650-780 29-32 2-4 500 - 939 15.6 21.5 Acacia Tortilis - 0.906 71.63 4.2 1.328 79.06 4.64 - Softwood (kraft - 1.5 1000 40 4.4 - - - pulp) Bamboo - 0.91 262 9.8 2.7 297.9 10.77 - Banana 80-250 1.3 - 1.35 355 - 754 7.7-33.8 5.3 273 - 560.77 25-26 - Coir/husk 121.3, 0.87-1.2 95 - 593 4-6 4-47 158 4.2 10.7 100-450 6 Cotton - 1.5 - 1.54 200 - 800 5.5-13 3-10 125 - 500 3.5 - 8.1 - Jute - 1.3-1.46 393 - 900 10-55 1.5 - 1.8 273.97 - 6.85 - 38 - 616.44 Kenaf 68.5-300 1.31-1.5 930 - 1500 23-53 1.6 - 17.3 361 - 641 19.2 - 36.5 52 Pineapple 20-80 1.32-1.543 654.413 - 1627 34.5-82.51 0.8 - 27 300 - 1100 20.554 95 Ramie 49.6 1.38-1.6 400-1000 44-128 2 - 3.8 220 - 938 20.6 16 Sisal 50-200 0.76-1.58 274 - 855 9-38 2-7 341 - 700 6.3 - 12.1 10 Flax 1.5 1500 27.6 3.2 - - - Hemp 1.47 690 70 4 - - - 608 609 Table 2- Mix Proportion for fiber cement boards (12 specimen per batch) Mix Proportioning by Weight (g) Samples Cement (g) Sand (g) Fiber (g) Water (mL) TOTAL (g) CFB UT - 1 548.59 1097.18 5.49 301.73 1952.99 CFB UT - 2 548.59 1097.18 21.95 301.73 1969.45 CFB UT - 3 548.59 1097.18 38.41 301.73 1985.91 CFB T - 1 548.59 1097.18 5.49 301.73 1952.99 CFB T - 2 548.59 1097.18 21.95 301.73 1969.45 CFB T - 3 548.59 1097.18 38.41 301.73 1985.91 CFB C 548.59 1097.18 0.00 301.73 1947.5 CFB – Cement Fiber Board UT – Untreated T – Treated 1 – 1% fiber 2 – 4% fiber 3 - 7% fiber C - control (0%) 610 611 Table 3 - Result of Analysis of Variance (ANOVA) P-value Tensile Experimental factors Flexural Tensile Parallel Perpendicular Treatment (a) 0.5945 0.1924 0.0001 Fiber content (b) 0.4262 0.6489 0.0006 Interaction (ab) 0.2620 0.5406 0.0256 (p-value ≤ 0.05 = significant difference) 612 613 Table 4 - Result of T-test on Tensile Perpendicular (TREATMENT) 614 26 PROPERTY P(T