Fundamentals of Ceramic Materials 2024-2025 PDF
Document Details
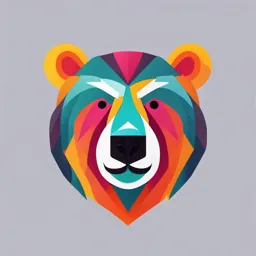
Uploaded by InsightfulHurdyGurdy
2024
Filiz Şahin
Tags
Summary
These notes cover the fundamentals of ceramic materials, specifically focusing on mechanical properties, elastic and plastic deformation. The document discusses stress-strain curves, elastic constants (Young's modulus, Poisson's ratio, etc.), strength, and hardness measurements for ceramics. It also includes information about fracture mechanics.
Full Transcript
FUNDAMENTALS of CERAMIC MATERIALS Prof.Dr. Filiz Şahin 2024-2025 Fall Mechanical Properties of Ceramic Materials 2 Introduction – Elastic Deformation 1. Initial 2. Small load 3. Unload...
FUNDAMENTALS of CERAMIC MATERIALS Prof.Dr. Filiz Şahin 2024-2025 Fall Mechanical Properties of Ceramic Materials 2 Introduction – Elastic Deformation 1. Initial 2. Small load 3. Unload bonds stretch return to initial d F F Linear- elastic Elastic means reversible! Non-Linear- elastic d 3 Introduction – Plastic Deformation 1. Initial 2. Small load 3. Unload bonds stretch planes & planes still shear sheared delastic + plastic dplastic F F Plastic means permanent! linear linear elastic elastic d dplastic 4 Introduction – Stress-Strain Curve Material I: High Young’s modulus (E) High failure stress Low ductility Low toughness No significant plastic deformation Ø Common for many ceramics Material II: Material III: Moderate strength Low E Moderate ductility, Very ductile Deforms plastically prior to failure Low ultimate tensile strength The toughest of the three Ø Common for many elastomers Ø Common for many metals 5 Introduction – Stress-Strain Curve Deformation of … Ceramics: Critical elasticity: ~ 0.01% Plasticity: ~0% Metals: Critical elasticity: ~ 1 - 2 % Plasticity: up to 50 - 100 % PMMA*: Critical elasticity: ~ several % Plasticity: up to several 100 % Stress * Polymethylmethacrylate (acrylic glass) Trade Name „Plexiglas“ 6 Strain Introduction – Elastic Constants § Modulus of elasticity (Young’s modlulus, E) § Poisson’s ratio (ν or μ) § Bulk modulus (stress to strain for hydrostatic compression) § Shear modulus (ratio of shear stress to shear strain) These constants are related directly to bonding forces between atoms, in real ceramics they are affected by microstructure, e.g., porosity and the presence of second phases. 7 Introduction – Modulus of Elasticity (Young’s Modulus) Modulus of Elasticity, E: à slope of the stress-strain curve in elastic region (also known as Young's modulus) Hooke's Law: s=Ee F s E e Linear- 2 factors affect the E F elastic 1. Bond strength simple tension 2. Temperature test 8 Introduction – Modulus of Elasticity (Young’s Modulus) At ambient and intermediate temperatures for short term loading, most ceramics behave elastically with no plastic deformation up to fracture (Brittle fracture) Most ceramic materials undergo plastic deformation at high temperature. Even at room temperature ceramics such as LiF, NaCl, and MgO undergo plastic deformation, especially under sustained loading. These ceramics all have NaCl structure, which has cubic symmetry and thus has many slip systems available for plastic deformation by dislocation movement. The magnitude of the elastic modulus is determined by the strength of the atomic bonds in the material. The stronger the atomic bonding, the greater the stress required to increase the inter atomic spacing, and thus the greater the value of the modulus of elasticity. Ceramics with weak ionic bonding have low E values (E for NaCl: 44.2 GPa) Ceramics with strong covalent bonding have high E values (E for diamond: 1035 GPa) 9 Introduction – Modulus of Elasticity (Young’s Modulus) Stress Silica glass-fiber: tensile deformations Strain lead to an increase in stiffness. This is related to the rotation of the (SiO4)4- tetrahedra, giving an increase in stiffness once the rotation has occurred. 10 T > 1800°C Plastic deformation occurs Also found in many covalent ceramics, such as Si3N4, at high temperatures. The inelasticity is caused by viscous flow of a glassy phase, which is often present in the grain boundaries of these materials 11 Measurement of Modulus of Elasticity Ultrasonic testing A dynamic method for determining elastic constants (Young’s modulus and shear modulus). Dynamic methods are more accurate than static methods 12 Measurement of Modulus of Elasticity 13 Poisson’s Ratio (ν or μ) Relates the longitudinal elastic deformation produced by a simple tensile or compressive stress to the lateral deformation that occurs simultaneously. ν = -εlateral / εlongitudinal For many ceramics, ν is ~ 0.18-0.30 14 Strength Theoretical strength can be defined as the tensile stress required to break atomic bonds and pull a structure apart. The theoretical strength for ceramic materials typically ranges from one-tenth to one-fifth of the elastic modulus. However, the theoretical strength of a ceramic material has not been achieved. This is due to the presence of fabrication flaws and structural flaws in the material, which result in stress concentration and fracture at a load well below the theoretical strength. 15 Strength However, it is evident that the severity of strength reduction is affected by a combination of factors: The shape of a pore The presence of cracks or grain boundary adjacent to a pore The distance between pores and between a pore and the surface The size and shape of an inclusion The differences in elastic moduli and coefficients of thermal expansion between the inclusion and the matrix 16 Strength Measurements Tensile strength is not common for ceramics--applied for metals Bending strength-3 point bending or 4 point bending Compressive Strength- mostly for concrete. Surface preparation of ceramics is important in strength testing that involves tensile stresses. Imperfections such as cracks, pores, large grains, or even scratches may lead to stress concentration and crack growth from these origins. Therefore, well-polished surfaces are required for less scattered data. In compressive stresses however, such imperfections do not play important roles since cracks or crack-like imperfections tend to be closed under these stresses. 17 3-Point Bending Strength Measurement The loading rate is usually between 0.5 mm/min and 1.0 mm/min σ = 3LF/(2bd²) in 3-point testof rectangular specimen L – specimen length; F – loading force; b – specimen width; d – specimen thickness ASTM C1161 18 4-Point Bending Strength Measurement σ = 3Fa/(bd²) in 4-point testof rectangular specimen L – specimen length; F – loading force; b – specimen width; d – specimen thickness; a -distance between the supporting and loading pins; 19 3- and 4-Point Bending Strength Measurement The main advantage of the bend test, other than its lower cost, due to simple sample geometries. The specimens have either a rectangular or cylindrical geometry. The four-point bend test is preferred because an extended region with constant bending moment exists between the inner rollers. 20 Bending Strength 21 Compressive Strength 22 Hardness Hardness is a material's resistance to permanent deformation. Hardness is a function of – the crystal structure, – crystal defects, – bond type, – fractional density, – grain size, – purity of the ceramic. External variables that may affect hardness are; – temperature, – the presence of reactive species, e.g. acids, alkalis, or even water in some cases. 23 Hardness Indentation test is used. Cracking can also occur on indenting à determining fracture toughness 24 Hardness Vickers hardness is a common method for ceramics 25 Hardness Usually the measured value of hardness is load-dependent. Especially at low loads the measured values of microhardness tend to increase. This reflects an effect of a ratio of the impression size to a characteristic microstructural dimension such as grain size, pore size and distribution, inclusions, and the range of residual stresses. The microhardness also depends on the relationship of surface properties vs bulk properties and on environmental interactions. At high loads, such as several kg, the measured hardness of ceramics decrease due to the fracture of the material under the indenter. 26 Stiffness and Hardness 27 Fracture Mechanics Measured fracture strength stress concentrator or stress raiser § Macroscopic internal discontinuities (e.g., voids), sharp corners, 28 notches in large structure –> stress concentrator or stress raiser Fracture Mechanics Cracks always exist under normal conditions at the surface and within the interior of a body of material. 29 Fracture Mechanics 30 Fracture Mechanics The maximum stress at crack tip (σm) Assumption: crack (surface crack with a length of "a") is similar to an elliptical hole through a plate, and is oriented perpendicular to the applied stress (σ0), maximum stress occurs at the crack tip 31 Fracture Mechanics – The maximum stress at crack tip The maximum stress at crack tip (σm) For long microcracks that has a small tip radius of curvature, the factor (a/ρt)1/2 will be very large à σm = many times of σ0 σm /σ0 = stress concentration factor (Kt) A measure of the degree to which an external stress is amplified at the tip of a crack. 32 Fracture Mechanics – Critical stress for crack propagation All brittle materials contain cracks and flaws with a variety of sizes, geometries, and orientations. When the magnitude of a tensile stress at the tip of one of these flaws exceeds the value of this critical stress, a crack forms, propagates, and results in fracture. Critical stress (σc) required for crack propagation in a brittle material: 33 Fracture Mechanics – Fracture Toughness Measure of a material’s resistance to brittle fracture when a crack is present. Unit of Kc: MPa·m1/2 Y: dimensionless parameter or function that depends on both crack and specimen sizes and geometries, as well as the manner of load application (Y =1 or Y = ~1.1) and σc: failure stress § This is the stress intensity factor at which the crack will propagate and lead to fracture. It is also referred to as fracture toughness. § The higher the fracture toughness, the more difficult it is to initiate and propagate a crack. As the crack size increases, the failure stress drops. To achieve a design failure stress, it must be possible to detect and prevent any cracks larger than a certain size. 34 Fracture Mechanics – Fracture Toughness Thickness of specimen is important! § For relatively thin specimens: Kc depends on specimen thickness § When specimen thickness >>> crack dimensions, Kc becomes independent of thickness; under these conditions a condition of plane strain exists. When a load operates on a crack in the manner represented in figure, there is no strain component perpendicular to the front and back faces. Kc value for this thick specimen is known as plane strain fracture toughness or fracture toughness (KIC or K1c ) plate of infinite width having a 35 through-thickness crack Fracture Mechanics - 3 modes of crack surface displacement Mode I, opening or tensile mode I in KIC denotes mode I Most commonly encountered Mode III, tearing mode Mode II, sliding mode Mode 1.If the load applied perpendicular to crack. Mode 2. and 3. when the load applied tangentially to the fracture. 36 Fracture Mechanics – Fracture Toughness (KIc) Kıc for brittle materials 96% of relative density using SPS at 1850 °C. The results showed that HfB2 and ZrB2 diffuses mutually to form (Zr,Hf)B2 solid solution, which enhances the fracture toughness of the material (HZ20S: 8.7 MPa·m0.5) when compared with H20S (5.2 MPa·m0.5) and Z20S (5.7 MPa·m0.5). The reinforcement of CNT (as HZ20S6C) and has led towards achieving the toughest ceramic (10.2 MPa·m0.5) in HfB2- ZrB2 system. Nisar, A.; Balani, K. Phase and Microstructural Correlation of Spark Plasma Sintered HfB2-ZrB2 Based Ultra-High Temperature Ceramic Composites. Coatings 2017, 7, 110. 49 Toughening Mechanisms – Factors contributing the fracture toughness of composite a) Volume fraction of reinforcement à b) Strength of the matrix/reinforcement interface. In fiber-reinforced composites a strong interface can lead to transfer of the stress from the matrix to the fibers; weak interface can lead to debonding and crack deflection à c) Young’s modulus of matrix and reinforcement. If a matrix is reinforced with high modulus, high strength fibers then more of the stress can be carried by the fibers. 50 Toughening Mechanisms – Transformation Toughening Phase transformation in ZrO2. Transformation toughening can lead to an improvement in both toughness and strength (preferred toughening mechanism) Manufacturing of partially stabilized zirconia Add about 10% MgO Sinter in the cubic phase to 1800 C Lower temperature (quench to room temp.) and heat treat (age- above 1400 C) to nucleate small precipitates of t-phase These are growing below the critical size for t-m transformation Cool to room temperature Remaining c-phase has no time to 51 transform Toughening Mechanisms – Transformation Toughening PSZ is a transformation-toughened material; Microcrack and Induced-stress may be two explanations for the toughening in partially stabilized zirconia. The Microcrack explanation depends upon difference in the thermal expansion between the cubic phase particle and monoclinic (or tetragonal)-phase particles in the PSZ. CTE for the monoclinic form is 6.5-6/° C up to 1200° C, 10.5-6/° C for cubic form is. This deference creates microcracks that dissipate the energy of propagating cracks 52 Toughening Mechanisms – Transformation Toughening After sintering at 1800°C an Mg-PSZ Microstructures annealing stage at 1400°C is introduced: -After 4-5 hours tetragonal precipitates, grow by conventional diffusion processes as coherent spheroids along {001} cube planes -Below a well defined critical size of about 200 nm the t-particles remain tetragonal down to room temperature!!!! - Optimum microstructures contains about 25% - 30% by volume of tetragonal phase in cubic matrix. Toughening Mechanisms – Transformation Toughening 1. The stresses concentrated at the crack tip transform the surrounding tetragonal ZrO 2 inclusions to the monoclinic crack polymorph. The transformation absorbs fracture energy and slows down crack propagation. tetragonal ZrO2 inclusion “ transformed to monoclinic structure stress orientation around the crack tip transformation zone Lense-shaped tetragonal inclusions in a matrix (black) of cubic zirconia (A. 200 nm Heuer). Toughening Mechanisms – Transformation Toughening 2. Microcracking around the transformed inclusions: The volume stresses resulting from the tetragona- monoclinic transformation delocalize also the stresses from the crack tip volume of the tetragonal zirconia inclusion crack volume after transformation to monoclinic stresses due to the volume increase microfracture due to the volume stresses Penetration depth 3. Crack deflection due to volume stresses: The deflection of cracks increases the crack surface.The stress releave per unit penetration is, therefore, larger then for an inclusion free zirconia. crack Toughening Mechanisms – Transformation Toughening 100nm Initially tetragonal zirconia inclusion in a cubic zirconia matrix, which are completely transformed to the monoclinic structure. The bands within the Monoclinic inclusions are twin lamellae. Toughening Mechanisms – Transformation Toughening § Zirconia-toughened alumina (ZTA), which contains 10–20 vol% of fine ZrO2, particles. § At high temperatures ZrO2 is tetragonal (t) and at low temperatures it is monoclinic (m). On cooling, t à m transformation may occur in ZrO2. § This transformation (not time dependent, very rapid) is accompanied by an increase in volume of about 3%. This volume change produces stresses in the Al2O3 matrix around the transformed particle leading to microcracking. § These microcracks increase the toughness of the ceramic by their ability to deflect and branch a propagating crack. Control of the extent of the microcracking determines the increase in toughness. If microcracking becomes extensive, strength will decrease! 57 Toughening Mechanisms – Transformation Toughening Several conditions are required for transformation toughening to occur. First, the matrix and zirconia should not react to form a new phase. Second, the dispersed zirconia phase should not be soluble in the matrix and vice versa. In addition, the following parameters must be optimized to maximize toughening: 1. the particle size of the zirconia, 2. the stabilizing phase concentration, 3. the particle size distribution, and 4. the particle-matrix thermal expansion mismatch. Unstabilized particles that are, larger than a critical size will transform spontaneously during cooling whereas particles that are too small will not transform, even under stress. The critical particle size for ZTA is 0.6 µm. (particle size of ZrO2 is 1.25 µm) 58 Toughening Mechanisms – The Effect of Different Toughening Mechanisms 59 References 60 Glass and Glass-Ceramics Introduction – Definition of Glass § Glass is an amorphous solid material (no arrangement on a scale larger than a few times the size of atom groups). https://www.sciencedirect.com/science/article/pii/B9781845699314000076 https://www.sas.upenn.edu/~milester/courses/chem101/LSChem101/LSPages/LSfigures174.ht ml Introduction – Definition of Glass § The term glass classically refers to any noncrystalline solid, which is formed by cooling from the melt. § Some glasses do not have to be formed by rapid melt cooling. ü For instance, sol-gel glasses are prepared by using organic-precursors or solutions prepared at low temperature. The solution is either poured into a mold then allowed to gel or is diluted and applied to a substrate by spinning, dipping, spraying or electrophoresis. § It possesses no sharp melting point and definite chemical formula. Glass – Amorphous Solid § Surface crystallization can occur § No volume crystallization XRD of lead-silicate glass. Introduction – Definition of Glass § Traditional glasses are made of inorganic materials like silica, sand, sodium and calcium carbonates, feldspars, borates, and phosphates. These inorganic materials form metallic oxides as they are melted together. § The glass composition is not limited to inorganic materials. There are many examples of organic glasses (Organic glasses consist of carbon-carbon chains which are so entangled that rapid cooling of the melt prevents reorientation into crystalline regions). Introduction – Definition of Glass A metastable solid with no long-range atomic order (short-range atomic order) § The material has a less stable state when it is in the form of glass and it reaches a more stable state by crystallization. Ø Glasses are metastable with respect to their stable crystalline phase Ø Atoms can rearrange to for more stable state given enough time and thermal energy Properties of Glass Amorphous (i.e. SRO) Brittle Transparent / Translucent Can absorb, transmit and reflect light Good electrical insulator Unaffected by air, water, acid or chemical reagents (but HF is an exception) High compressive strength Properties of Glass Mechanically Strong- Glass has great inherent strength and is weakened only by surface imperfections. Hard Surface- Glass resists scratches and abrasions Chemical corrosion resistance- Glass is affected by a few chemicals. It resists most industrial and food acids. Heat Absorbent- Glass retains heat, rather than conducts it. Glass absorbs heat better than a metal. Optical Properties- Glass reflects, bends, transmits and absorbs light with great accuracy. Volume vs Temperature § Volume of the material decreases as if the melt is being cooled with a steady cooling rate along the ab line. § If the cooling is slow enough and there is nuclei in the melt then the liquid crystallizes at temperature Tf, with a sudden change in volume given with bc. § As the cooling goes on, the crystalline material follows the cd line. § If the cooling rate is high enough, crystallization does not occur at Tf and the volume of the supercooled liquid decreases along be. Glass Transition Temperature (Tg) Tg: Glass transition temperature. At Tg, the volume-temperature curve continues almost parallel to the curve of the crystalline material and demonstrates a change in gradient. Hence the material can be referred as glass only below Tg. Viscosity of the material at Tg is very high ~ 1012 Pa·s (In the glass- transition interval, viscosity increases from about 108 to 1012 Pa·s) Volume vs Temperature § A material is a supercooled liquid in the temperature range between Tg and Tf. Its volume will decrease along the vertical arrow until it comes to the be line, if the glass is held at temperature just below Tg. § In the glass transition region, other properties of the material also change which are time dependent. This effect is named as stabilization. § Supercooled liquid cannot reach a more stable state without crystallization. Hence, there are no time-dependent changes above Tg. § The material has a less stable state when it is in the form of glass and it reaches a more stable state by crystallization. Hence, properties of glasses depend on the cooling rate to a certain degree especially in the temperature range near Tg due to stabilization effects. Structural Theories of Glass Formation 1) Early structural theories § Tammann (1925), investigated the formation and constitution of glasses and regarded them as strongly undercooled liquids. § Tammann studied the thermodynamics of glass structures. 2) Goldschmidt’s theory-radius ratio criterion § Goldschmidt derived empirical rules about glass formation. § Goldschmidt stated that the ratio of atomic radii of the cations and anions controls the formation of glass. § According to Goldschmidt theory, glass formation is only possible if the CN = 4 for all the glass forming oxides. § This criterion was first agreed for SiO2, P2O5 and later it was understood that the theory was also true for GeO2 and BeF2. § Theory lacks the appreciation of the bonding type and assumes that glass-forming oxides are assumed to be purely ionic due to the radius ratio of the ions and CN but later it is understood that most of the glass forming oxides such as SiO2 have covalent character. CN: coordination number Structural Theories of Glass Formation 3) Zachariasen’s random network theory § The random network theory proposed by W. H. Zachariasen for the structure of oxide glasses is very important. § The random network theory characterizes “the glass network as an infinitely large unit cell containing an infinite number of atoms, which does not have any periodicity”. § The theory and the requirement for a material to form glass infers that the structures should be disordered and open structures are more likely to give rise to such disorder, because the coordination polyhedral need not share edges and faces. Edge and face sharing induce crystalline order. (Zachariasen, 1932) Structural Theories of Glass Formation 3) Zachariasen’s random network theory Zachariasen formulated a set of rules for glass formation: 1. An oxygen atom is linked to not more than two glass-forming atoms, 2. The CN of the glass-forming atoms, which are cations, is small: 3 or 4, 3. The oxygen polyhedra share corners with each other, not edges or faces, 4. The polyhedra are linked in a three-dimensional network (at least 3 corners are shared) Same type of oxygen polyhedra of low CN exists in both crystalline and glassy states since they have similar energies. As the bond angle of A-O-A varies in the network structure, crystalline structure loses its periodicity. Long-range columbic interactions force edge and face sharing of the oxygen polyhedra in highly ionic materials and hence these compounds are not good glass formers such as MgO, Al2O3 and TiO2. Only applies to most (not all) oxide glass (Zachariasen, 1932) Highlights the importance of network topology Structural Theories of Glass Formation 3) Zachariasen’s random network theory Zachariasen categorized the cations in a glass structure according to their role in the glass network 1) Network-formers: Si4+, B3+, P5+, Ge4+, As3+, Be2+, where CN is 3 or 4. 2) Network-modifiers: Na+ , K+ , Ca2+, Ba2+, where CN ≥ 6. 3) Intermediates: reinforce (CN=4) or loosen the network further (CN is 6 to 8). Ø SiO2, B2O3, P2O5 GeO2, As2S3 and BeF2 are network formers. Structural Theories of Glass Formation 3) Zachariasen’s random network theory Ø Short-range order is preserved Ø Long-range order is disrupted by changing bond angle (mainly) and bond length Ø Structure lacks symmetry and is usually isotropic The glass network can be defined as a corner sharing Crystalline Glass oxygen polyhedra, which does Zachariasen’s Random Network not have periodicity. Theory (1932) Structural Theories of Glass Formation Network-modifiers: Na+ , K+ , Ca2+, Ba2+, where CN ≥ 6: § As Na2O is added into the SiO2 structure, it breaks the Si-O-Si linkages by cutting the oxygen bridges and forms Si-O- terminations à structure is depolymerized or modified. § The oxygen bonds in the network, Si-O-Si linkages, are known as bridging oxygens. § The oxygen bonds, in the Si-O- linkages are known as non-bridging oxygens. SiO2 à network former Na2O à network modifier Structural Theories of Glass Formation Intermediates: reinforce (CN=4) or loosen the network further (CN is 6 to 8). § Al2O3 does not form glass. § In aluminosilicate crystals the CN of Al =4 and CN of O =2. Hence, aluminosilicate crystals obey the Zachariasen’s rules and form glasses. These oxides are known as intermediates or conditional glass formers. § Intermediate oxides do not form glass by themselves but act like glass formers if they are combined with others. § Aluminosilicate, aluminoborate and aluminophosphate glasses are examples for intermediate oxides. Structural Theories of Glass Formation 4) Dietzel and field strength Dietzel classified elements according to their field strength (Fs). This considers the forces (attraction / repulsion) between cations and anions in the glass. 5) Kinetic theory of glass formation FUNDAMENTALS OF CERAMIC MATERIALS Prof.Dr. Filiz Şahin 2024-2025 Fall Glass Raw Materials – Base materials (glass makers, melts, stabilizers) and – Auxiliary materials (fining agents, dyes, discoloration agents, opal glass and melting accelerators). The raw materials for making glass are all oxides. The composition of any sample of glass can be given in terms of the percent of each oxide used to make it. Glass Raw Materials The basic materials for glass production are: – Quartz (Silicon dioxide (𝑺𝒊𝑶𝟐 )) – Soda (Sodium Carbonate (𝑵𝒂𝟐 𝑪𝑶𝟑 ) (using in soft glass)) – Limestone (Calcium Carbonate (𝑪𝒂𝑪𝑶𝟑 )) – Potash (Potassium Carbonate (𝑲𝟐 𝑪𝑶𝟑 ) (using in hard glass)) – Dolomite (Magnasium Calcium Carbonate (𝑴𝒈𝑪𝒂(𝑪𝑶𝟑 )𝟐 )) – Crushed glass forms 25-30% of the whole mixture and has to be of the right size; the crushed glass pieces must not be too large and also too small, since the latter make the clarification process more difficult Auxiliary Materials Auxiliary materials are added to base materials: – Materials for glass discoloring and clarification of glass mixture (Manganese dioxide), – Materials for coloring are metal oxides, – Materials for opaque glass texture (Titanium and Zirconium oxides) Composition of Glass When sand is mixed with metal oxides, melted at high temperatures, and then cooled to a rigid condition without crystallization, the product is called glass. By adding soda (𝑵𝒂𝟐 𝑪𝑶𝟑 ) to the sand, its melting point and viscosity are both lowered, making it much easier to work it. Lime (CaO) is added to the sand and soda mixture so that the " soda-lime " glass will not dissolve in water. Components of Glass Because glass is used in so many different ways, there is no chemical composition for each glass sample (composition range). There are so many different glass compositions but only 3 categories of substances in all glass. These are: – Network Formers – Network Formation – Network Modifiers (Fluxes, Softeners) – Intermediate Oxides (Stabilizers – Provide Chemical Resistance) Components of Glass The network former makes up the bulk of the glass. Silica (𝑆𝑖𝑂# ) in the form of sand is the most common type Other possible formers include 𝐵# 𝑂$ and 𝑃# 𝑂%. The network modifiers (fluxes) change the temperature at which the formers melt during the manufacturing of glass. 𝑁𝑎# 𝐶𝑂$ and 𝐾# 𝐶𝑂$ The stabilizers (or intermediate oxides) strengthen the glass and make it resistant to water. – 𝐶𝑎𝐶𝑂$ is the most frequently used Network Formers, Modifiers and Intermediates Glass network formers – Form the interconnected backbone glass network Glass network modifiers – Present as ions to alter the glass network – Compensated by non-bridging oxygen (NBO) in oxide glasses – Usually reduce glass network connectivity Intermediates – Can behave as network formers or modifiers depending on glass composition – Improve chemical resistance (especially Al2O3) – Behave as stabilizing agent (e.g. TiO2, ZrO2, CeO2) Network Formers, Modifiers and Intermediates § 3D glass network predominantly consisting of corner-sharing 𝑆𝑖𝑂& tetrahedral interconnected by bridging oxygen (BO) § High network connectivity: high softening point, low diffusion coefficient, small coefficient of thermal expansion (CTE) ü Each alkali ion creates one non bridging oxygen ü Reduced network connectivity viscosity decreases (compared to silica at the same T), diffusion coefficient Si: Glass Former and CTE increases Na: Network Modifier ü Increased ionic conductivity, reduced chemical resistance Network Formers, Modifiers and Intermediates Glass former: high valence state, covalent bonding with oxygen Modifier: low valence state, ionic bonding with oxygen Alkali - Alkaline Earth Silicate Glass Each alkaline earth ion creates two NBOs Increased network connectivity compared to alkali silicates stabilized glass network (Na2O), improved chemical resistance Approximate composition of commercial soda lime glass (window glass) 16𝑁𝑎2𝑂·10𝐶𝑎𝑂·74𝑆𝑖𝑂2 11 Borate Glass 𝐵2𝑂3:the glass former consisting of corner sharing. 𝐵𝑂3 triangles connected by bridge oxygen 12 Network Formers, Modifiers and Intermediates Example: Window glass composition Example: Borosilicate glass composition Glass Manufacturing Melting Forming and Shaping Annealing Finishing Melting Raw materials in proper proportions are mixed It is finely powdered and intimate mixture called ʹ batch ʹ is fused in furnace at high temperature of 1800 C this charge melts and fuses into a viscous fluid 𝐶𝑎𝐶𝑂3+𝑆𝑖𝑂2→𝐶𝑎𝑆𝑖𝑂3+𝐶𝑂2↑ 𝑁𝑎2𝐶𝑂3+𝑆𝑖𝑂2→𝑁𝑎2𝑆𝑖𝑂3+𝐶𝑂2↑ After removal of 𝐶𝑂2 decolorizes like 𝑀𝑛𝑂2 are added to remove traces of ferrous compounds and carbon Heating is continued till clear molten mass is free from bubbles is obtained and it is then cooled to about 800 C 15 Forming and Shaping The Viscous mass obtained from melting is poured into molds to get rid of different types of articles of desired shape by either blowing or pressing between the roller 16 Annealing Glass articles are the allowed to cool gradually at room temperature by passing through different chambers with descending temperatures This reduces the internal strain in the glass. 17 Finishing Finishing is the last step in glass manufacturing It involves following steps ü Cleaning ü Grinding ü Polishing ü Cutting ü Sand Blasting 18 19 Characterization GLASS-CERAMICS: PROCESSING, PROPERTIES and APPLICATIONS Glass-Ceramic § Enhancing mechanical properties of glasses with crystallization § Controlled microstructure, crystalline phases (+ residual glassy phase) Controlled Heat Treatment nuclei crystal glass formation growth ceramic Nucleating Agents v Nucleating agents promotes heterogeneous nucleation, which could be controlled much more easily comparing with homogenous crystallization surface crystallization mechanism. v Nucleating agents increase the number of heterogeneous nucleation sites. v Nucleating agents are composed of v either metal elements dispersed as colloids (Au, Ag, Pt,Pd, etc.) v or simple oxides (TiO2, ZrO2, P2O5, Ta2O5, etc.) v Agents are added to glass composition as 1-8 mol% for oxides and less than 1 mol% for colloids for the efficient volume crystallization with material that has enhanced properties v Processing and thermal, optical, chemical, biological, mechanical, electrical and magnetic properties could be controlled by considering composition, morphology, amount and orientation of crystals in the glass-ceramics. Electrical and Processing Thermal Optical Chemical Biological Mechanical Magnetic Method Controllable Translucency or Resorbability Biocompatibility Machinability Isolation Limited and expansion opacity High chemical Bioactivity High strength and Ion conductivity controllable depending on Pigmentation durability toughness Superconductivity shrinkage temperature Photo-induction Ferromagnetism No porosity in High temperature Opalescence, monolithic G/C stability fluorescence Military High thermal stability High radar wave transparence Low CTE Optical Electrical High ion High transparence conductivity Chemical Zero porosity stability Cost-effective Good formability Application areas Construction Medical High strentgh High strength Aesthetic Aesthetic Low cost Bioactive q Kitchenware Aesthetic Low CTE Glass-Ceramic Production Designing of Glass Ceramics 1. Choice of the parent glass composition for desired crystalline phase. 2. Synthesis of the glass via a melting process (followed by quenching) and shaping. 3. Controlled crystallization of the glass. (microstructure) https://wellcomecollection.org/works/w935sf9w v The composition determines; § The nature of the future crystalline phase(s), § Nucleation and growth § Thermodynamics and the kinetics of the system § Properties Glass-Ceramic Production Fabrication from glass batch; §Melting of glass §Casting and cooling of molten glass §Nucleation and grain growth to convert G/C Fabrication from glass powder; §Sintering of the glass powder by holding during heating §Cooling of the bulk Glass-Ceramics in Dentistry: A Review - Scientific Figure on ResearchGate. Available from: https://www.researchgate.net/figure/The-two- manufacturing-processes-of-glass-ceramics-a-The-classic_fig4_339500415 [accessed 11 Sep, 2022] Glass–Ceramic Glazes for Ceramic Tiles: A Review - Scientific Figure on ResearchGate. Available from: https://www.researchgate.net/figure/Evolution-of-the-microstructure-from-glass-to-glass-ceramic-through-volume_fig1_225719990 [accessed 11 Sep, 2022] https://www.ivoclar.com/en/p/all/all-ceramics/ips-emax-system-technicians/ips-emax-cad/ Glass-Ceramic – LAS (Lithium Aluminosilicate) v Most common glass ceramic LAS (Li2O-Al2O3-SiO2) v Transparent v Heat – resistant v Low thermal expansion of coefficient (CTE) v It could be colored with the addition of nucleating agents like TiO2, ZrO2 LAS glass-ceramics are used in cooktop panels for kitchen stove and in fireplace glass doors Glass-Ceramic – MAS (Magnesium Aluminosilicate) v Most important crystal phase in MAS is cordierite (Mg2Al4Si5O18) v High mechanical strength v Excellent dielectric properties v Good thermal stability v Thermal shock resistance § The development of MAS glass–ceramic was motivated by the need arose for a ceramic missile nosecone for a missile to be guided by an internal antenna. Glass-Ceramic - MACOR® § Machinable glass ceramics with nucleated fluoromica crystals in glass vAerospace industry: More than 200 special parts of the U.S. space shuttle orbiter are made of this glass-ceramic. These parts contain rings at all hinge points, windows, and doors. v Medical equipment: Machinability of the material and its inert character are particularly significant in the fabrication of specialized medical equipment. vUltrahigh vacuum applications: Excellent insulators and pore-free material which is used to manufacture equipment for vacuum technology. vWelding: It is used in welding equipment which has excellent nonwetting properties with regard to oxyacetylene. vNuclear-related experiments: Irradiation resistant material. https://m.turkish.industrial-ceramicparts.com/photo/pt33610095- macor_machinable_glass_ceramic.jpg Glass-Ceramic - DICOR® § Machinable glass ceramics which are used for dental applications § Translucency and chemical durability are improved comparing to MACOR® § Includes tetrasilisic mica: KMg2,5AlSi4O10F2 § Good strength (~150 MPa) is associated with the development of anisotropic at relatively high temperatures (> 1000°C). § Ceria addition is made to catch fluorescency of natural teeth. HÖLAND, W., & RHEINBERGER, V. (2008). Dental glass-ceramics. Bioceramics and Their Clinical Applications, 548–568. doi:10.1533/9781845694227.2.548 Two usage areas in dentistry; § Dental crowns or inlays are produced with lost-wax technique. § Machining of dental restorations by DICOR® MGC Glass-Ceramic - DICOR® A schematic (a) and an example (b) demonstrating interlocking effect in glass–ceramics. The interlocking microstructure of the mica glass-ceramic allows the material to be machined. Cracks propagate in a zig path, instead of propagating in a direct path, which effectively consumes the energy of cracks and slows crack propogation. Fu, L., Engqvist, H., & Xia, W. (2020). Glass–Ceramics in Dentistry: A Review. Materials, 13(5), 1049. doi:10.3390/ma13051049 Glass-Ceramic – BIOVERIT I and BIOVERIT II § Biocompatible and machinable depending on the high mica content § Machinability (BIOVERIT III) § in orthopedic surgery, § especially different types of head and neck surgery, § middle ear implants Middle-ear implants of BIOVERIT II What are Refractory Materials? Refractories are non-metallic inorganic materials capable of withstanding high temperatures and not degrading in a furnace environment when in contact with corrosive liquids, gases and solids. Refractory insulators are used in high-temperature applications – to provide proper processing environment – to reduce heat losses and – to save fuel 44 45 Properties of Refractory Materials Withstand high temperatures under high load (high creep resistance) High volume stability. Withstand sudden changes in temperature. Low coefficient of thermal expansion. High thermal shock resistance Withstand the action of molten metal slag, glass, hot gases, etc. (high corrosion resistance) Withstand the abrasive/wear/erosive forces. Able to conserve heat. Thermal conductivity (low or high depending on properties of thermal shock and insulation) Density and porosity (depending on dense or insulating character requirements) 46 FIGURE 1.1 Industry wise global refractory consumption (in percent) 47 Manufacturing and Properties Crushing of Refractories about 25 mm Shaped Refractories Grinding 200 mesh Refractory raw materials are normally available as hard lumpy materials, which are crushed first in primary crusher like jaw Screening crusher (if the size of the lumps is above 50 mm) and then in the secondary crusher like hammer mill. The crushed materials are screened to several size fractions like 3–5 Batch Weighting mm, 1–3 mm, 0–1 mm, and fines. The different size fractions are mixed together along with a binder Mixing and pressed in a mold of desired size in high capacity press to form the brick. Shaping The brick is then dried to remove the moisture inside and fired at high temperature (1150–1750 °C) to impart the strength and desired properties to the refractory. Drying Firing 48 (1150–1750 °C) Physical Properties of Refractories; 1) Refractoriness 2) Strength of refractories under load 3) Dimensional stability 4) Porosity 5) Thermal spalling 6) Thermal expansion 7) Thermal conductivity 49 Physical Properties of Refractories 1) Refractoriness Ability of a material to withstand the heat, without appreciable deformation or softening under particular servise conditions. In general, measured as the softening or melting temperature of the material. Most of the refractory materials are mixture of metallic oxides, they do not have a sharp melting point. Pyrometric Cones Test (Seger Cones Test) The softening temperature of the refractory materials are determined by using Pyrometric Cones Test. Expressed in terms Pyrometric Cone Equivalents (PCE) Softening Temp. of Mat. to be used as Refractory ≫ Operating Temp. 50 Pyrometric Cones Test (Seger Cone Test) Refractoriness is determined by comparing the behaviour of the heat on cone of material to be tested with that of a series of Seger Cones of standart dimensions. Pyrometric cone is a refractory specimen of standart dimension (38 mm hight abd 19 mm triangular base) and composition Seger cones melt or fuse at definite temperature when heated under standart conditions of 10 C/min. ISO 528:1983--; EN 993-12: The temp. at which the fusion or softening of the test cones occures is indicated by its apex touching the base. 51 Pyrometric Cone Equivalent (PCE) Number PCE Number: Value representing the number of standard cones which also fuses with the test cone for a given refractory. 52 2) Strength of Refractories Under Load (RUL) It is used to determine the temperature at which a standard dimensioned specimen of a refractory undergoes 10 % deformation with constant load of 3.5 or 1.75 kg/cm2 ( standart heating rate 10 C/min.) The RUL test gives only an index of refractory quality rather than a figure which can be used in a refractory design. TS EN ISO 1893 53 TS EN ISO 1893 Ta; temperature at which 0.6 % def. occurs (3 mm deflection) Tc ; temperature at which 40 % def. occurs (20 mm deflection) Usage temp. of refractory< Ta Safe Ref. having narrow range between Ta-Tc 54 3)Dimensional Stability Resistance of a material to any volume change which may be occur on its exposure to high temperature, over a prolonged time. Dimensional change can be permanent or reversible Irriversible change may result either in the contraction or expansion of a refractory 55 56 4) Porosity All refractories contain pores, either due to manufacturing methods or deliberately made (by incorporating saw-dust or cork during manufacture). Porosity is the ratio of its pore’s volume to bulk volume. TS EN 993-1 57 58 5) Thermal Spalling Property breaking, cracking or peeling of refractory material under high temperature. Thermal spalling mainly due to 1.Rapid change in temperature This causes uneven expansion and contraction with the mass of a refractory, leads to development of uneven stresses and strain. 2.Slag penetration This causes variation in the coefficient of expansion, leads to spalling. Thermal spalling can be decreased by a)Using high porosity, low CTE and good thermal conductivety b)Avoiding sudden temperature change c)By modifiying furnace design. 59 6)Thermal expansion Expansion of a refractory material when exposed to heat for a longer duration. Refractory expansion has an impact on the capacity of the furnace lifetime. Reapeted expansion and contraction of refractory materials due to thermal impact causes wear, tear, breakdown… Refractory materials should have least possible thermal expansion. TS EN 993-19 60 7) Thermal conductivity A good heat conductivity of the refractory material is desirable for effective heat transmission in furnace construction. The densest and least porous brick have the highest thermal conductivity, owing to the absence of air-voids. In porous bricks, entrapped air in pores acts as a non heat conducting materials. Thermal conductivity depends on Temperature Chemical and mineralogical composition of the brick Porosity, pore size Th.cond. at 1000C (W/m.K) Brick firing temperature TS EN 993-15 61 Classification of Refractories Refractories are generally classified based on different parameters, e.g.: (a) Basicity of oxides (b) Form (c) Manufacturing process (d) Method of application (e) Special chemistry (f) Insulating property 62 Refractories By Basicity 1. Acidic refractories are those refractories which readily react with bases at high temperatures. The most common examples of acidic refractories are fire clay and silica. 2. Basic refractories Complimentary to the idea of acidic refractories are basic refractories. These often react with acids at high temperatures. Magnesite and dolomite. 3. Neutral refractories Neutral oxides are prized for their lack of reactivity with acids and bases, and are often considered superior refractories for their wide utility and performance over a broad number of applications. Neutral refractories are used across many applications, as they are tolerant to both acidic and basic atmospheres and slags Table: Classification of refractories based on basicity. 63 By Insulating Property of Refractory Most refractory linings are composite in nature and made of the combination of - dense refractories, in front, to contain the high temperature and to withstand the harsh operating condition and - insulating refractories, at back, to contain the heat and protect the energy loss from the system. The main features of insulating refractories are the high porosity and low bulk density which leads to low thermal conductivity and low mechanical strength of the insulating refractory. Insulating refractories are made out of aluminosilicate range of materials because of their inherent lower thermal conductivity compared to many other materials. The insulation product can be designed for continuous use at a very high temperature (1600 °C). 64 Besides normal insulating bricks and castables, there are other types of insulating refractories available made of ceramic fiber. The advantages of ceramic fiber lining are: 1. Ceramic fibers have very low bulk density. 2. Ceramic fibers are virtually completely resistant to thermal shock. Ceramic fiber lined furnaces can be heated up very fast and can be cooled also very fast. 3. Ceramic fiber can withstand thermo-mechanical stresses better than insulation bricks or castables. 4. Ceramic fiber lining is easier and cheaper to install. 65 Silica brick made from naturally occurring sources of silica and bonded by adding 3.0–3.5% CaO to promote liquid phase sintering. Semisilica brick. A silica brick containing 18–25% Al2O3. Fireclay brick. Made from kaolinite (Al2O3.2SiO2.2H2O) with 25–45% Al2O3. High-alumina brick. Alumina content in the range 45–100 wt.%. Dolomite brick. Made from dolomite (CaCO3.MgCO3). Magnesia brick. Contains mainly MgO (typically >90% MgO). Chrome brick. Made from naturally occurring chrome ore. Contains 34% Al2O3 and 30% Cr2O3. Often MgO is added to produce chrome-magnesia brick. Zircon refractory brick. Zircon is ZrO2.SiO2. Bricks may contain 4% CaO. 66 Diagram of a blast furnace indicating the type of refractories used in each region. 67 Silica Refractories Silica refractories contain a minimum of 95% SiO2. The common impurities are CaO, Fe2O3, and Al2O3. The main raw material used, is quartzite. The combined Al2O3 and TiO2 should be as low as possible, <2% Alkali <0.3% and for high-quality products it should be below 0.1%. Various grades of silica brick have found extensive use in the iron and steel melting furnaces and the glass industry. 68 Properties of Silicate Refractories; High resistance to thermal shock( above 600 C) and high thermomechanical resistance up to 1600 C. It does not begin to soften under high loads until its fusion point is approached. (alumina silicate materials, which begin to fuse and creep at temperatures considerably lower than their fusion points. ) High flux and slag resistance, volume stability and high spalling resistance 69 Quartz shows polymorphic transformation to different crystalline forms when heat treated at different temperatures with some being reversible and some irreversible in nature. Volume changes occur in silica associate with polymorphic transformation. The horizontal arrows in figure indicate the transformations which occur slowly, and the vertical arrows show the transformations that occur fast. The suitable quartzite for manufacturing of silica refractories must not degrade fast on heating!!! 70 The degeneration can be measured by testing the sp. gravity of the quartzite after firing at 1460 °C from ambient temperature and holding for 1 h at peak temperature. Degeneration behavior of quartzit. The milk of lime (slurry of Ca(OH)2 in water) is used as binder which provides 1–2.5% CaO, in the composition, that acts as a mineralizer and speeds up the polymorphic transformations in the horizontal direction. The addition of Fe2O3 also promotes the conversion of quartz to desirable phase. 71 CaO reacts with SiO2 above 600 °C and forms β-2CaO.SiO2(C2S) and 3CaO.SiO2 (C3S), which play a significant role in the development of mechanical strength in silica refractory. Tridymite is the most desirable phase in silica refractories although all the crystalline forms of silica exist in silica refractory. Residual or free quartz is the most undesirable phase in a silica refractory. The main problem associated with the existence of free quartz is its conversion to tridymite and cristobalite in service which is accompanied by 14% volume expansion and causes crack to the refractory. The determination of specific gravity also gives a good indication about the presence of free quartz in the refractory body. A silica brick without free quartz should have a sp. gravity below 2.34. Silica refractories are prepared from grounded quartzit mixing with CaO for mineralizing and sulphite solution as binder. After shaping with hydraulic press, bricks are fired 1450-1500ºC for 200-330 hours. 72 Aluminosilicate Refractories The main chemical constituents of this category of refractories are alumina (Al2O3) and silica (SiO2). If the Al2O3% is less than 50%, it is normally categorized as fireclay refractory, and refractory with more than 50% Al2O3 is called high alumina refractory, Fireclay The main raw material used for fireclay refractories is calcined fireclay or chamotte, along with plastic fireclay. Fire-clay brick comprise about 75% of the production of refractories on a volume basis. Fireclay refractories have alumina and silica as main constituents. The other oxides which remain present as impurities are Fe2O3, TiO2, CaO, MgO, K2O, and Na2O. All these oxides are acting as flux and bring down the softening temperature of the refractory Typical composition consists of SiO22) Densities ~3.50 g/cc or more Large periclase crystal sizes (1000 microns minimum) 83 Due to its relatively high chemical stability, strength, resistance to abrasion, and excellent corrosion resistance, refractory-grade fused magnesia is used in high wear areas in steelmaking, like the molten metal and slag contact areas. The important properties to characterize the magnesia raw material are as follows: 1. Bulk density and grain porosity 2. The impurities and their distribution 3. CaO/SiO2 ratio 4. Boron content 5. Periclase crystal size 84 85 Phase diagram of magnesia with lime 86 (CaO) and iron oxide (Fe2O3) 87 MAIN APPLICATION AREAS OF MAGNESIA Highly pure magnesia refractories (MgO ∼95%–97%, CaO ∼2%–1.5%, and SiO2 ∼1%– 0.5%) are useful for the upper part of the glass tank furnace regenerator checker work. Resistance to alkali and sulfur attack is of prime importance in that region. The next-level purity class magnesia refractories (MgO ~95%, CaO ~2%, and SiO2 ~2%) are used in a lime kiln, where lime stone is calcined, and high basic environments prevail. Magnesia refractory with 92% MgO and impurities about 2% CaO and ∼4% SiO2 are used for the hot metal mixer in the iron and steel industries. Backup of the lining of converters, ladles, and electric arc furnaces of steel industries are done by using relatively less pure magnesia refractories (MgO ∼87%, CaO ∼2%, and SiO2 ∼6%). Relatively inferior magnesia refractories containing about 50% MgO are used for the reheating furnace hearth applications. 88 ZIRCON AND ZIRCONIA REFRACTORIES Zircon (ZrSiO4) is the silicate of zirconium, containing 67% ZrO2 and 33% SiO2. It is used as a refractory material directly and also used as a raw material for zirconia (ZrO2). The main reason for these materials to become a refractory is the presence of zirconia in them, which is high melting, chemically inert, and imparts special mechanical properties in the refractories. Zircon has a wide range of applications as a ceramic and refractory material, for example, As refractory, it is mainly used for the construction of glass tank furnaces and nozzles for iron and steel industries. As molds and cores in precision, investment casting. As protective coatings on steel molding tools. As an opacifier in the glaze for ceramic and whiteware industries due to its high refractive index. As the principal precursor for the preparation of metallic zirconium and its compounds, like zirconia. 89 The wide range of applications of zircon is due to its excellent thermophysical properties such as low thermal expansion, good thermal stability, high corrosion resistance against glass melts, slag, and liquid metals and alloys. Zircon is one of the most chemically stable compounds, and mineral acids other than HF cannot attack zircon. Very aggressive reaction conditions are required to break down the strong bonding between the zirconium and silicon parts of the compound. The main feature of the zircon refractories is their acidic slag resistance, excellent thermal shock resistance, good mechanical strength with wear and erosion resistances. 90 Dissociation of Zircon Zircon (ZrSiO4) decomposes by a solid-state reaction, and chemically pure zircon decomposes in solid state at 1676 °C to form a mixture of tetragonal zirconia and cristobalite. ZrSiO4 t-ZrO2 + SiO2 (Cristobalite) But the presence of impurities reduces the decomposition temperature, which may even start at 1285°C. The more the impurities present in zircon, the lower the onset temperature of dissociation. To reduce the degree of zircon degradation, impurities, like iron, titanium, aluminum, and alkali must be minimum. 91 Zircon refractories are used as ladle nozzles for steel pouring, tundish metering nozzles, in furnaces for melting aluminum, as compounds and coatings. Zircon refractories with at least 63% zirconia and maximum 20% apparent porosity are used in glass tank furnace. It is also an ideal mold and chill sand due to its low thermal expansion rate, high thermal conductivity, and non-wettability with molten metal. Zircon is also used in core and mould washes to improve surface finish. 92 Preparation of Zircon Based Refractories Zircon-based refractories are prepared by using beneficiated zircon aggregate or sand, fine- milled zircon, and a temporary binder. It is then pressed into desired shape and size, and finally the shapes are fired. The firing temperature must be lower than the decomposition temperature of zircon. 93 ZIRCONIA REFRACTORIES Zirconia is mainly obtained by chemical processing of zircon or by carbothermal reduction (fusion) of zircon in an electric arc furnace. Zirconia has a natural source, called baddeleyite, commonly found in igneous rocks containing felspar, zircon, etc. Baddeleyite is chemically homogeneous, Main impurities ; hafnium (Hf), titanium (Ti), and iron (Fe). Zirconia is an important refractory material due to its excellent high-temperature properties and chemical inertness. But high cost and cracking tendency (due to polymorphic transformation) have restricted its wide application!!! At ambient condition, zirconia has a monoclinic structure, which instantaneously changes to tetragonal form during heating around 1180°C and from the tetragonal to the cubic form around 2370°C. This polymorphic transformation causes a huge volume expansion during cooling and cracking and failure of the material. Hence for any high-temperature application, zirconia has to be stabilized, with CaO/MgO/CeO2/Y2O3. 94 Zirconia refractories have refractoriness above 2000°C and also have excellent chemical resistance to the action of melts, alkalis, and most of the acids. But due to the high cost, they are only used for very specific critical applications. The main application areas are the metering nozzle in tundish (for continuous casting of steel) inserts in the bore area in the slide gate plates (in steel ladles). In combination with carbon, it is used as a band in the subentry nozzle (SEN) to resist the corrosive attack of mold powders during continuous casting, etc. Zirconia is mostly used as a component in a refractory composition mainly to improve mechanical, corrosion, and thermal shock properties. It is also used as crucibles for melting platinum, palladium, and other metals and quartz glass, and also in the construction of nuclear reactors. Lightweight zirconia products, fibers, and granular powders are suitable for high-temperature thermal insulation. It is also used as heating elements at temperatures up to 2200°C in furnaces with resistive and induction heating. 95 Fused Cast Al2O3–ZrO2–SiO2 (AZS) Refractories Fused cast Al2O3–ZrO2–SiO2 refractories, abbreviated as AZS, are important for their corrosion resistance and wear properties mainly due to its compositions and considerable ZrO2 content in them. They are the most widely used materials both in glass contact areas and superstructure of glass melting furnaces due to their very high resistances against glass and alkali vapor. These refractories are further divided as per ZrO2 content. According to ASTM C 1547-02, these refractories are classified by the amount of monoclinic zirconia (ZrO2) present, as determined by chemical analysis or quantitative image analysis. 96 Heindrich R., Gupta A.,”Fused Cast AZS Glassy Phase Exudation: Intrinsic or Pathologic Property?” RHI Bulletin , 2, 2011, pp.24-28 The refractory consists of two major interlocked crystalline phases (i.e., corundum and zirconia) and one glassy/vitreous phase that fills the interspace of the crystalline structure. Therefore, fused cast AZS has no open porosity, which is one reason for the high corrosion resistance to molten glass. The glassy phase can, above its transition temperature, relax mechanical stress present in fused cast refractories that could result in block cracking. The composition of this viscous alumina silicate melt phase is approximately: 70% SiO2, 20% Al2O3, 5% Na2O, and 2% ZrO2 with some wt.% of other oxides. Whilst the weight percent of glassy phase in an AZS block amounts to about 22%, the volume equivalent is approximately 33%. 97 FUNDAMENTALS OF CERAMIC MATERIALS Prof.Dr. Filiz Şahin 2024-2025 Fall Powder Processing Ceramic Raw Materials The nature of the raw material has a major effect on the final properties of a ceramic component. Purity, particle size distribution, reactivity, polymorphic form, availability, and cost must all be considered and carefully controlled. 1. Traditional ceramic raw materials; Derived from common, naturally occurring raw materials such as clay minerals and quartz sand. Used for traditional ceramics such as wall and floor tiles, sanitary ware, white ware, refractories, bricks…. 2. Advanced ceramic raw materials; Mostly derived from synthetic(not naturally occurred) powders obtained by special synthesis processes. High purity materials and precise methods of production must be employed to ensure that the desired properties of these advanced materials are achieved in the final product. Used for special purpose such as space shuttle tile, engine components, artificial bones and teeth, computers and other electronic components, and cutting tools,. 3 1.Traditional Ceramics Raw Materials Ceramics have been produced for centuries. The earliest ceramic articles were made from naturally occurring raw materials. Early civilizations found that clay minerals became plastic when water was added and could be molded into shapes. The shape could then be dried in the sun and hardened in a high-temperature fire. Many of the raw materials used by the ancient civilizations are still utilized today and form the basis of a sizable segment of the ceramic industry. These ceramic products are often referred to as traditional ceramics. 4 Ceramic Tile Production Process 5 6 7 8 Traditional Ceramics Raw Materials Clay Kaolin Quartz Felspar Wollastanite Talc 9 CLAY MINERALS They form the basis of pottery and building bricks. They are layer materials and a subgroup of the sheet layer silicates. The clay minerals are hydrated aluminum silicates based on (Si 2O5)n sheets. They contain chemically bound water. Clays occure by the weathering of aluminosilicate rocks and sedimentation. 10 In terms of formation, kaolins are formed as a result of the alteration of feldspars in granitic or volcanic rocks into the mineral kaolinite. Primary Formation Secondary Formation Formation Formation through resulting from the the transportation of decomposition of decomposed feldspathic rocks in kaolinite by nature. floodwaters, erosion, etc Higher purity, Due to impurities whiter raw color and firing in the structure, color, darker raw and coarse-grained firing colors, Less plasticity. fine-grained. 11 CLAY MINERALS They have a broad range of physical characteristics, chemical compositions, and structures. Common impurities include compounds (usually oxides) of Ba, Ca, Na, K and Fe, and also some organic matter. Average particle size 2 µm 4 µm 12 CLAY The plate like morphology of clay important; 1. Gives easy cleavage which leads to a fine particle size and a narrow particle size distribution. 2. Allows the particles to easily move over one another. TEM image of single hexagonal plates of Planar and hexagonal plates kaolinite 13 Functions of Clay in Ceramic Body 1. Develop plasticity when mixed with water (hydroplasticity); the plasticity of clay suspensions is basic to many of the forming processes commonly used to fabricate ceramic bodies. 2. Clays melt over a temperature range (depending on composition) thus, a dense and strong ceramic piece can be produced without losing their shape or without complete melting. Desired shape can be maintained. The formed piece green body is dried to remove some of the moisture, and then fired at an elevated temperature to improve its mechanical strength 14 CLAY MINERALS Most of the clay based products fall within 2 broad classifications; 1. Structural clay products (building bricks, tiles, etc applications in which structural integrity is important). 2. Whitewares (become white after the high temperature firing Porcelain, pottery, tableware, sanitary ware etc.) 15 CLAY MINERALS Plasticity: When mixed with limited amounts of water, clays become plastic, and are able to be molded and formed easily. Example: Flour mixed with water = Easy form (plastic), Sand mixed with water = not plasticity Water have to add to clay to get plasticity, nothings give plasticity to clay except water. Cohesion: This property proves the clay to keep its form when it dried. Since sand doesn’t have this property, it changed its form when it dried. 16 CLAY MINERALS Color: Since clays contain some mixed oxides in their body, they are naturally colored. If the clay is pure, its color is white and is called as kaolin. Their color can be yellow, pink, reddish, blue-gray, green or black. Their colors give some information about their compositions; · If the clay contains limonite, its color is dark. · If it contains iron peroxide, its color is red. · If it contains manganese oxide, its color is black. · Organic materials change the clay color to violet. However its color can change after firing due to change in color of oxides at high temperatures. Shrinkage: The dimensions of clay after forming with water reduce during drying. This volume shrinkage continues during the firing step of ceramic material processing. This shrinkage is related with plasticity of the clay. 17 CLAY MINERALS There are 6 types of commercial clays. Their composition, plasticity, color firing characteristics are different. 18 CLAY -Kaolinite Al2(Si2O5)(OH)4 The most common clay mineral. The bonding within this two layered sheet is strong and Electron micrograph of kaolinite crystals. They intermediate ionic covalent, are in the form of hexagonal plates, some of adjacent sheets are only loosely which are stacked on top of one another (x21k) bound to one another by weak 19 van der Waals forces CLAY -Kaolinite Al2(Si2O5)(OH)4 The general structure of the kaolinite group is composed of silicate sheets (Si2O5) bonded to aluminum oxide/hydroxide layers (Al2(OH)4) called gibbsite layers. The silicate and gibbsite layers are tightly bonded together with only weak bonding existing between the s-g paired layers It also known as China clay. It contains coarse grains (10-0.1µm). Fired color is white. Uses: In ceramics, as a filler for paint, rubber and plastics and the largest use is in the paper industry that uses kaolinite to produce a glossy paper such as is used in most magazines. In ceramic industry, kaolinite mostly used for sanitary ware, porcelain and insulator materials. For tiles processing it uses max.20 % of kaolinite. The most famous kaolinite reserves are situated in southwest of England, the Ukrain China. In Turkey; Balıkesir(M.Kemal Paşa), Çanakkale (Biga, Çan), Eskişehir (Bigadiç), Uşak, Bursa, İstanbul 20 CLAY -Kaolinite Al2(Si2O5)(OH)4 Chemical formula of kaolinite; Al2O3.2SiO2.2H2O Chemical composition of kaolinite; Al2O3 46.54 % SiO2 39.50 % H2O 12.96 % When kaolinite heated upto 200°C, hygroscopic water removes. At 500-600°C;crystal water removes and it transforms metakaolinite as follows; 3(Al2O3.2SiO2.2H2O) 3(Al2O3.2SiO2) + 6H2O At 1000°C metakaolinite transforms to mullite and silicate (Crystobalite); 3(Al2O3.2SiO2) 3Al2O3.2SiO2 + 4SiO2 Mullite forms at 1000°C from metakaolinite, is in the nidle form crystall structure rather than layers and causes very high hardness, chemical resistance, high strength and electrically insulator structure. 21 CLAY -Kaolinite Al2(Si2O5)(OH)4 Natural impurities (i e MgO, CaO, Na2O K2O in kaolinite act as mineralizers, which promote the crystallization of different mineral phases and enhance strength at 1000°C 22 CLAY –other Clay Minerals ü Halloysite; Al2(Si2O5)(OH)4·2H2O ü Pyrophyllite; Al2(Si2O5)2(OH)2 ü Montmorillonite; Al1.67Na0.33Mg0.33(Si2O5)2(OH)2 ü Mica; Al2K(Si1.5Al0.5O5)2(OH)2 ü Illite; Al2-xMgxK1-x-y (Si1.5y Al0.5+y O5)2 (OH)2 These minerals have different stacking of the silica and alumina layers as well as, incorporating metal hydrates of Na, K, Mg, Al or Fe between the silica and alumina layers 23 24 In Turkey there are 2 different clay rich areas. These are; Bilecik-Söğüt Istanbul-Şile-Kemerburgaz 1.500.000 ton clay produces in a year and whole clay is consumed in Turkish ceramic production industry. Main clay producers are; Matel A.Ş., Kalemaden A.Ş., Toprak A.Ş., Sörhaz A.Ş., -in Istanbul Söğüt A.Ş., Essan A.Ş., etc.-in Söğüt 25 Quartz Quartz is the most common mineral found on the surface of the Earth. A significant component of many igneous, metamorphic and sedimentary rocks, this natural form of silicon dioxide is found in an impressive range of varieties and colors. Silica (Si02) is a major ingredient in glass, glazes, enamels, refractories, abrasives, and white ware. Its major sources are in the polymorphic form quartz, which is the primary constituent of sand, sandstone, and quartzite. 26 Silica does not have plasticity, improves strength at high temperature in ceramic body. Quartz; reduces drying shrinkage of ceramic structure helps to regulate plasticity allow gas outlet during firing without deformation. Quartz is available in different minerals in the nature. Quartz sand: The most common minerals of quartz. It generally contains iron compounds. Quartz sand without iron use in the ceramic industry. Quartzit: It is pure quartz stone. It is available as large rocks. It is commonly used in the ceramic industry. Flint stone: It is used as milling media in mills. Diatomite: It has porous structure and it is amorphous. It is used for high temperature furnace bricks. 27 28 The silica has many different polymorphic forms. Increasing the temperature, forms are stable; – α-quartz – β-quartz – β-trydimite – β -crystobalite The transformation phases between α and β is displacive type The transformations between quartz, tridimite and crystobalite are reconstructive. 29 Feldspar In the manufacture of ceramics, feldspar is the second most important ingredient after clay. Feldspar is the name of a group of rock-forming minerals which make up as much as 60% of the Earth's crust. Feldspar minerals range in composition from KAlSi3O8 (Orthoclase) to NaAISi3O8 (Albite) to CaAl2Si2O8 (Anorthite). Feldspar does not have a strict melting point, since it melts gradually over a range of temperatures. This greatly facilitates the melting of quartz and clays and, through appropriate mixing, allows modulations of this important step of ceramic making. Feldspars are used as fluxing agents to form a glassy phase at low temperatures and as a source of alkalies and alumina in glazes. They improve the strength, toughness, and durability of the ceramic body. Feldspar is a source of Al2O3 which improves the mechanical properties of glass such as its scratch resistance and its ability to withstand thermal shock. 30 Melting Range of Feldspar Na-Feldspar :1150-1225°C K-Feldspar :1200-1250°C Ca-Feldspar :1500-1550°C Chemical Composition, (%) 31 32 Wollastonite Wollastonite forms from the interaction of limestones, that contain calcite, CaCO3, with the silica, SiO2, in hot magmas( high temperature and pressure). It forms by the following formula: CaCO3 + SiO2 ----> CaSiO3 + CO2 It contains 48.3 % CaO and 51.7 % SiO2. It is an important constituent in refractory ceramics (those ceramics that are resistant to heat) such as refractory tile and as a filler for paints. Some of the properties that make wollastonite so useful are its high brightness and whiteness, low moisture and oil absorption, and low volatile content. Wollastonite is used primarily in ceramics, friction products (brakes and clutches), metal making, paint filler, and plastics. It improves strength of ceramic products and reduces firing time of tiles. 33 Wollastonite Source of water insoluble calcium is wollastonite (CaO·SiO2). Wollastonite is found in either a pure form or in association with garnet or calcite and dolomite. In glazes, wollastonite may be used as a substitute for calcite, which reduces the volatiles and increases the gloss and texture of the glaze Wollastonite deposits are known for their low iron content which gives a glaze with an excellent fired color In enamels, wollastonite acts as a natural frit to reduce gas evolution It is used in ceramic insulating bodies and as an auxiliary flux in electrical insulators 34 Talc Talc is a mineral composed of hydrated magnesium silicate with the chemical formula H2Mg3(SiO3)4 or 3MgO.4SiO2.H2O. Natural raw material for producing tile, dinnerware, and electronic components Reasonable sintered strengths can be obtained when talc is sintered at 1000°C. 35 Talc In loose form, it is the widely used substance known as talcum powder. It has a perfect basal cleavage, and the folia are non-elastic, although slightly flexible. It is soft, with a hardness of 1 (Talc is the softest of the Mohs' scale of mineral hardness). It has a specific gravity of 2.5–2.8, a clear or dusty luster, and is translucent to opaque. Its colour ranges from white to grey or green and it has a distinctly greasy feel. Its streak is white. 36 Talc Talc is primarily formed via hydration and carbonation of serpentine, via the following reaction; Serpentine + Carbon Dioxide → Talc + Magnesite + Water 2Mg3Si2O5(OH)4 + 3CO2 → Mg3Si4O10(OH)2 + 3MgCO3 + 3H2O Talc can also be formed via a reaction between dolomite and silica, which is typical of skarnification of dolomites via silica-flooding in contact metamorphic aureoles; Dolomite + Silica + Water → Talc + Calcite + Carbon Dioxide CaMg(CO3)2 + 4SiO2 + H2O → Mg3Si4O10(OH)2 + 3CaCO3 + 3CO2 Talc can also be formed from magnesian chlorite and quartz in blueschist and eclogite metamorphism via the following metamorphic reaction: Chlorite + Quartz → Kyanite + Talc + H2O In this reaction, the ratio of talc and kyanite is dependent on aluminium content with more aluminous rocks favoring production of kyanite. 37 Usage of Talc A coarse grayish-green high-talc rock is soapstone or steatite and has been used for stoves, sinks, electrical switchboards, etc. Talc finds use as a cosmetic (talcum powder), as a lubricant, as a filler in paper manufacture. Talc is used in baby powder, an astringent powder used for preventing rashes on the area covered by a diaper (see diaper rash). In the ceramic industry, due to its low volume expansion, it is used in bathroom and kitchen ceramics and even in electrical stoves plates. 38 TRADITIONAL RAW MATERIALS Clay Plasticity à formability Must be clean and pure enough for Feldspar (Flux) products Reduction for vitrification Sand temperature Increasing strength Additives may be added for improving particular properties 39 The increase of clay makes easy molding processes The increase of feldspar lowering the temperature of sintering and introduces in a liquid phase sintering The increase in silica limit shrinkages and deformations in sintering. 40 2.Advanced Ceramics Raw Materials During the past 50 years scientists and engineers have acquired a much better understanding of ceramic materials and their processing and have found that naturally occurring minerals could be refined or new compositions synthesized to achieve unique properties. These refined or new ceramics are often referred to as modern ceramics or advanced ceramics. They typically are of highly controlled composition and structure and have been engineered to fill the needs of applications too demanding for traditional ceramics. 41 General Comparison The wide variety of application possibilities for advanced ceramic materials arise from their specific properties which in many respects cannot be achieved by other materials. To highlight some positive properties: low density, high hardness, high mechanical strength, dimensional stability (specific stiffness), resistance to wear, resistance to corrosion (resistance to chemical attack), weathering resistance, high working temperature, low or high thermal conductivity, good electrical insulation and dielectric and ferroelectric properties 42 In accordance with their chemical composition the advanced ceramic materials can be divided into main groups SILICATE-CERAMICS technical porcelain-The natural starting materials of technical porcelain are quartz, feldspar and kaolin. steatite -major component: soapstone, additives: clay and flux, Mg 3 Si4 O10 (OH)2 cordierite -These magnesium silicates occur during the sintering of soapstone with added clay, kaolin, corundium and mullite. Mg2Al4Si5O18 mullite-ceramic -3 Al2O3.2 SiO2 OXIDE CERAMICS aluminium oxide-Al2O3 magnesium oxide-MgO zirconium oxide-ZrO2 aluminium titanate-Al2TiO5 or Al2O3.TiO2 piezo ceramic-lead zirconate to lead titanate NONOXIDE CERAMICS CARBIDES NITRIDES silicon carbide-SiC silicon nitride-Si3N4 tungsten carbide-WC aluminium nitride-AlN boron carbide-B4C boron nitride-BN carbide-TiC SIALON Titanium nitride-TiN BORIDES ELEMENTEL Titanium Borides- TiB2 Boron Zirc