Basis Of Telescopes PDF
Document Details
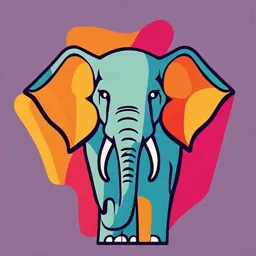
Uploaded by RetractableParrot
İstanbul University
Tags
Summary
This document provides an in-depth analysis of the basis of telescopes, encompassing various topics such as observational instruments, methods, telescope optics, and atmospheric effects. It covers topics like chromatic aberration, spherical aberration, telescope scale, pixel scale, and focal ratio. The document is structured as a set of slides, each presenting a detailed component of telescope and optical systems.
Full Transcript
Type equation here. ÖNEMLİ UYARI: Bu sunum İ.Ü. İstanbul Fen Fakültesi Lisans öğrencilerinin eğitimlerine ücretsiz katkı sağlamak amaçlı hazırlanmış olup, bilimsel kaynak olarak gösterilemez, ücretlendirilemez, izinsiz kaydedilemez, kullanılamaz, çoğaltılamaz ve ticari bir ürün haline dönüştürülemez...
Type equation here. ÖNEMLİ UYARI: Bu sunum İ.Ü. İstanbul Fen Fakültesi Lisans öğrencilerinin eğitimlerine ücretsiz katkı sağlamak amaçlı hazırlanmış olup, bilimsel kaynak olarak gösterilemez, ücretlendirilemez, izinsiz kaydedilemez, kullanılamaz, çoğaltılamaz ve ticari bir ürün haline dönüştürülemez. İSTAN B U L ÜNİVERSİTESİ OBSERVATIONAL INSTRUMENTS & METHODS CLASS CODE: ASUB3229 (STUDENTS ENROLLED AFTER 2017-2018 EDUCATION SEASON) CLASS CODE: ASUB4195 (STUDENTS ENROLLED BEFORE 2017-2018 EDUCATION SEASON) BASIS OF TELESCOPES Telescope scale: For a telescope of focal length 𝒇 Lens Focal Plane BASIS OF TELESCOPES Pixel scale: A measurement of how much of the sky is covered by one pixel. 𝝎=𝝁×𝑺 𝑺; Telescope Scale (“/mm) 𝝁; pixel size (mm) 𝝎; pixel scale (“) BASIS OF TELESCOPES Focal Ratio: A lens or mirror's focal length divided by its aperture. E.g. If diameter of the telescope is 100cm and the focal length is 800cm, F=8 It is shown as f/8 Brightness of a source by telescope is proportional with F2 So same source with the same exposure time, the view by a telescope with F=f/6 is brighter than the one by a telescope with f/10. For the same brightness f/6 telescope needs less exposure time than f/10. BASIS OF TELESCOPES The angular resolving power (or resolution) of a telescope is the smallest angle between close objects that can be seen clearly to be separate. Intensity BASIS OF TELESCOPES The angular resolving power (or resolution) of a telescope is the smallest angle between close objects that can be seen clearly to be separate. BASIS OF TELESCOPES The angular resolving power (or resolution) of a telescope is the smallest angle between close objects that can be seen clearly to be separate. Rayleigh Criterion: Two point objects are just resolvable if they are separated by the angle; BASIS OF TELESCOPES The angular resolution of a telescope in practice is limited by; 1. Rayleigh limit. The theoretical angular resolution. 2. Atmospheric seeing 3. Detectors pixel scale 4. Optical Aberrations (will be discussed later) BASIS OF TELESCOPES light-gathering power: The ability of a telescope to collect light. This is one of the most important features of a telescope with angular resolution. Proportional to the area of mirror; e.g. Twice the mirror diameter multiple the power by four. BASIS OF TELESCOPES Ocular lens (eyepiece): A type of lens that is attached to a variety of optical devices such as telescopes and microscopes. The eyepiece is placed near the focal point of the objective to magnify this image. The amount of magnification depends on the focal length of the eyepiece. BASIS OF TELESCOPES Ocular lens (eyepiece): A type of lens that is attached to a variety of optical devices such as telescopes and microscopes. The eyepiece is placed near the focal point of the objective to magnify this image. The amount of magnification depends on the focal length of the eyepiece. Angular magnification BASIS OF TELESCOPES Telescopic images in the absence of an atmosphere. Plane wavefronts diffract upon encountering the circular aperture, and focus as an Airy disk: a bright central spot surrounded by rings of decreasing brightness. BASIS OF TELESCOPES Astronomical seeing: image formation by a telescope in a turbulent atmosphere. (a) In a short exposure, wavefront distortions caused by variations in refractive index in the atmosphere produce interfering Airy patterns. In (b) turbulent motion in the atmosphere during a long exposure moves the individual maxima around the image plane to produce a large seeing disk. BASIS OF TELESCOPES Atmospheric refraction We can approximate the Earth’s atmosphere as a series of plane-parallel plates, and the surface as an infinite plane. We imagine that the atmosphere consists of a very large number of thin layers, so in the limit, the effect of refraction is to curve the path of the incident ray. In this limit, the plane-parallel model gives BASIS OF TELESCOPES Atmospheric refraction We can approximate the Earth’s atmosphere as a series of plane-parallel plates, and the surface as an infinite plane. We imagine that the atmosphere consists of a very large number of thin layers, so in the limit, the effect of refraction is to curve the path of the incident ray. In this limit, the plane-parallel model gives where n is the index of refraction at the surface. The quantity (𝒏 − 𝟏) 𝒙 𝟏𝟎𝟔 is called the refractivity. The refractivity of air is R0 in radians times 106. BASIS OF TELESCOPES Aberration - Aberration Types 1. Monochromatic Aberration: Effetcs both refracting and reflecting telescopes. Independent of wavelength. a) Spherical Aberration b) Coma c) Astigmatism d) Curvature of field e) Distortion 2. Chromatic Aberrations: Due to the refracting indice depends on the wavelength. Seen in refracting telescopes. BASIS OF TELESCOPES Chromatic aberration Since n(λ) for optical glasses decreases with wavelength in the visible, then the focal length of a convex lens will be longer for red wavelengths than for blue. Different colors in an image will focus at different spots. This inability to obtain perfect focus is called chromatic aberration. Atmospheric refraction as a function of wavelength. Data are for dry air at 0oC and standard pressure. Actual refractivity will depend on local temperature, humidity and pressure. BASIS OF TELESCOPES Chromatic aberration Since n(λ) for optical glasses decreases with wavelength in the visible, then the focal length of a convex lens will be longer for red wavelengths than for blue. Different colors in an image will focus at different spots. This inability to obtain perfect focus is called chromatic aberration. BASIS OF TELESCOPES Chromatic aberration To correct chromatic aberration in a lens, the usual strategy is to cement together two lenses made of different glasses, a positive (convex) lens with low chromatic dispersion, and a negative lens with lower absolute power, but higher chromatic dispersion. If powers are chosen to be inversely proportional to dispersions, then the combination will have a finite focal length but reduced dispersion over a significant part of the spectrum An achromat is a lens whose focal length is the same at two different wavelengths. An apochromat, can bring three different wavelengths to a common focus, but only through use of expensive materials. A superapochromat, can bring four different wavelengths to a common focus. BASIS OF TELESCOPES Monochromatic Aberrations – wavefront aberrations An aberration is an imperfection in telescope design that degrades an image. Chromatic aberration is present when rays of differing wavelength fail to reach a common focus. Unfortunately, some aberrations are present even in monochromatic light A ”perfect” telescope; should transform an incident plane wavefront into a converging spherical wavefront whose center is at the focus predicted by the paraxial approximation – the Gaussian focus. For point sources off-axis, the perfect telescope should produce spherical wavefronts converging somewhere on the Gaussian image plane, also as predicted by paraxial theory. Straight lines in the sky should produce straight lines in the image plane. This mapping of the points and lines on the sky to points and lines in the image plane is called a collinear transformation. If an optical system fails to produce this collinear transformation it is said to exhibit aberrations. BASIS OF TELESCOPES Monochromatic Aberrations – Spherical Aberrations Focus is not at the Gaussian focus. Two types; Longitudinal Spherical Aberration Transverse Spherical Aberration BASIS OF TELESCOPES Monochromatic Aberrations – Spherical Aberrations Focus is not at the Gaussian focus. Two types; Longitudinal Spherical Aberration Transverse Spherical Aberration BASIS OF TELESCOPES Monochromatic Aberrations – Spherical Aberrations Focus is not at the Gaussian focus. Two types; Longitudinal Spherical Aberration Transverse Spherical Aberration Angular Spherical Aberration BASIS OF TELESCOPES Monochromatic Aberrations – Spherical Aberrations Focus is not at the Gaussian focus. Two types; Longitudinal Spherical Aberration Transverse Spherical Aberration Angular Spherical Aberration λ = 550 𝑛𝑚 D ≈ 0.007𝐹3 D = 10𝑐𝑚, 𝐹 ≈ 11 D = 1𝑚, 𝐹 ≈ 24 BASIS OF TELESCOPES Monochromatic Aberrations – Off-Axis Aberrations Coma, Astigmatism Curvature of field Distortion Of the four off-axis aberrations, only the first two, coma and astigmatism, actually degrade the image resolution, while the other two only alter the image position. BASIS OF TELESCOPES Monochromatic Aberrations – Off-Axis Aberrations Coma; Coma is the wavefront aberration that depends on ρ𝟑 𝒃𝒄𝒐𝒔𝜙, which means, like spherical aberration, it is particularly a problem of large apertures. BASIS OF TELESCOPES Monochromatic Aberrations – Off-Axis Aberrations Coma; Coma is the wavefront aberration that depends on ρ𝟑 𝒃𝒄𝒐𝒔𝜙, which means, like spherical aberration, it is particularly a problem of large apertures. BASIS OF TELESCOPES Monochromatic Aberrations – Off-Axis Aberrations Coma; Coma is the wavefront aberration that depends on ρ𝟑 𝒃𝒄𝒐𝒔𝜙, which means, like spherical aberration, it is particularly a problem of large apertures. An optical system with neither SA nor coma is called aplanatic. No single element aplanatic telescope is possible, either in a refractor or reflector. As with SA, large focal ratios will reduce coma, but impose penalties in image brightness and telescope length. Otherwise, minimizing coma in refracting systems requires a system of lenses and, fortunately, achromatic doublet or triplet designs that minimize SA also reduce coma. Aplanatic reflecting telescope designs require two mirrors. BASIS OF TELESCOPES Monochromatic Aberrations – Off-Axis Aberrations Astigmatism; Astigmatism is an aberration whose wavefront distortion depends on the term ρ𝟑 𝒃𝟐 𝒄𝒐𝒔𝟐𝜙Therefore, it increases more rapidly than coma for off-axis images. BASIS OF TELESCOPES Monochromatic Aberrations – Off-Axis Aberrations Astigmatism; Astigmatism is an aberration whose wavefront distortion depends on the term ρ𝟑 𝒃𝟐 𝒄𝒐𝒔𝟐𝜙Therefore, it increases more rapidly than coma for off-axis images. All rays pass through two line segments. One line, called the sagittal focus, is in the meridional plane, and extends on either side of the Gaussian image point. The other line segment, called the meridional focus, is in the sagittal plane, centered on the focus point of the tangential rays. BASIS OF TELESCOPES Monochromatic Aberrations – Off-Axis Aberrations Astigmatism; Astigmatism is an aberration whose wavefront distortion depends on the term ρ𝟑 𝒃𝟐 𝒄𝒐𝒔𝟐𝜙Therefore, it increases more rapidly than coma for off-axis images. All rays pass through two line segments. One line, called the sagittal focus, is in the meridional plane, and extends on either side of the Gaussian image point. The other line segment, called the meridional focus, is in the sagittal plane, centered on the focus point of the tangential rays. All uncorrected refractors and all practical two-mirror reflectors suffer from astigmatism. In many cases, the astigmatism is small enough to be ignored. In other cases, a corrector lens or plate removes the astigmatism. If a telescope design is free from astigmatism, coma, and SA, it is called an anastigmatic aplanat. For large anastigmatic telescopes, the correction plate is usually located near the focal plane. For small telescopes, a popular design that can be made anastigmatic is the Schmidt–Cassegrain, a two-mirror telescope with a corrector plate located at the aperture BASIS OF TELESCOPES Monochromatic Aberrations – Off-Axis Aberrations Field curvature; In the absence of other aberrations, optic theory predicts that off-axis images will fall on a spherical surface known as the Petzval surface, not on the plane predicted in paraxial theory. The radius of the Petzval sphere depends on the curvatures and refractive indices of the mirrors and lenses in the optical system. Detectors tend to be flat. A flat detector placed tangent to the Petzval surface, will necessarily record most of its images out of focus. For a small detector, this defocus will not exceed the seeing disk or diffraction limit and therefore not present a problem. A large enough detector, however, will produce blurred mages because of field curvature. BASIS OF TELESCOPES Monochromatic Aberrations – Off-Axis Aberrations Field curvature; In the absence of other aberrations, optic theory predicts that off-axis images will fall on a spherical surface known as the Petzval surface, not on the plane predicted in paraxial theory. The radius of the Petzval sphere depends on the curvatures and refractive indices of the mirrors and lenses in the optical system. One solution is to bend the detector to match the Petzval surface. This has been done for many years with glass photographic plates. Large solid-state detectors like charge-coupled devices (CCDs) are mechanically quite fragile, so bending them is not an option. In many telescopes, a corrector plate or lens, which may also help remove other residual aberrations, serves to flatten the field. BASIS OF TELESCOPES Monochromatic Aberrations – Off-Axis Aberrations Distortion; Distortion relocates images in the focal plane so that the colinearity requirement is violated – straight lines on the sky become curved lines in the focal plane. (a) Pincushion distortion of an object that has a square outline centered on the optical axis. (b) Barrel distortion of the same object. BASIS OF TELESCOPES Monochromatic Aberrations BASIS OF TELESCOPES Monochromatic Aberrations Qualitative appearance of images of a point source in optical systems with a single aberration present. Discrete rays strike the entrance aperture in a pattern spaced around multiple. Concentric circles. Actual sizes of the aberrations will depend upon details of the system. In the diagram, ‘‘focus’’ means the best compromise on-axis focus, which may differ from the Gaussian focus. TELESCOPES Telescope mounts and drives Fixed mounts Fixed altitude mounts Transit mounts Altazimuth mounts Equatorial mounts TELESCOPES Telescope mounts and drives Fixed mounts Fixed telescope mounts are entirely fixed in one position, such as Zenith telescopes that point only straight up Fixed altitude mounts Transit mounts Altazimuth mounts Equatorial mounts TELESCOPES Telescope mounts and drives Fixed mounts Fixed altitude mounts Fixed-altitude mounts usually have the primary optics fixed at an altitude angle while rotating horizontally (in azimuth). They can cover the whole sky but only observe objects for the short time when that object passes a specific altitude and azimuth. Transit mounts Altazimuth mounts Equatorial mounts TELESCOPES Telescope mounts and drives Fixed mounts Fixed altitude mounts Transit mounts Transit mounts are single axis mounts fixed in azimuth while rotating in altitude, usually oriented on a north-south axis. Altazimuth mounts Equatorial mounts TELESCOPES Telescope mounts and drives Fixed mounts Fixed altitude mounts Transit mounts Altazimuth mounts Altazimuth, altitude-azimuth, or alt-az mounts allow telescopes to be moved in altitude (up and down), or azimuth (side to side), as separate motions. Equatorial mounts TELESCOPES Telescope mounts and drives Fixed mounts Fixed altitude mounts Transit mounts Altazimuth mounts Equatorial mounts The equatorial mount has north-south "polar axis" tilted to be parallel to Earth's polar axis that allows the telescope to swing in an east-west arc, with a second axis perpendicular to that to allow the telescope to swing in a north-south arc. Slewing or mechanically driving the mount's polar axis in a counter direction to the Earth's rotation allows the telescope to accurately follow the motion of the night sky. TELESCOPES Telescope mounts and drives German mount & Fork Mount TELESCOPES Telescope mounts and drives German mount & Fork Mount TELESCOPES Telescope mounts and drives Fixed mounts Fixed altitude mounts Transit mounts Altazimuth mounts Equatorial mounts TELESCOPES Telescope mounts and drives Fixed mounts Fixed telescope mounts are entirely fixed in one position, such as Zenith telescopes that point only straight up Fixed altitude mounts Transit mounts Altazimuth mounts Equatorial mounts TELESCOPES Telescope mounts and drives Fixed mounts Fixed altitude mounts Fixed-altitude mounts usually have the primary optics fixed at an altitude angle while rotating horizontally (in azimuth). They can cover the whole sky but only observe objects for the short time when that object passes a specific altitude and azimuth. Transit mounts Altazimuth mounts Equatorial mounts TELESCOPES Telescope mounts and drives Fixed mounts Fixed altitude mounts Transit mounts Transit mounts are single axis mounts fixed in azimuth while rotating in altitude, usually oriented on a north-south axis. Altazimuth mounts Equatorial mounts TELESCOPES Telescope mounts and drives Fixed mounts Fixed altitude mounts Transit mounts Altazimuth mounts Altazimuth, altitude-azimuth, or alt-az mounts allow telescopes to be moved in altitude (up and down), or azimuth (side to side), as separate motions. Equatorial mounts TELESCOPES Telescope mounts and drives Fixed mounts Fixed altitude mounts Transit mounts Altazimuth mounts Equatorial mounts The equatorial mount has north-south "polar axis" tilted to be parallel to Earth's polar axis that allows the telescope to swing in an east-west arc, with a second axis perpendicular to that to allow the telescope to swing in a north-south arc. Slewing or mechanically driving the mount's polar axis in a counter direction to the Earth's rotation allows the telescope to accurately follow the motion of the night sky. TELESCOPES Telescope mounts and drives TELESCOPES Telescope mounts and drives German mount & Fork Mount TELESCOPES Telescope mounts and drives German mount & Fork Mount TELESCOPES Telescope mounts and drives Telescope mounts in space: To adjust and stabilize the orientation of a telescope in space: Methods: Small rockets Rockets require a supply of propellant. For example, bursts of a compressed gas escape from a nozzle to produce a force on the telescope. Spinning reaction wheels. Reaction wheels require an on-board motor – when the rotation speed of the wheel is changed, the telescope begins to rotate in the opposite direction. TELESCOPES Telescope mounts and drives Telescope mounts in space: To adjust and stabilize the orientation of a telescope in space: Methods: Small rockets Rockets require a supply of propellant. For example, bursts of a compressed gas escape from a nozzle to produce a force on the telescope. Spinning reaction wheels. Reaction wheels require an on-board motor – when the rotation speed of the wheel is changed, the telescope begins to rotate in the opposite direction. TELESCOPES Telescope mounts and drives Telescope mounts in space: To adjust and stabilize the orientation of a telescope in space: Methods: Small rockets Rockets require a supply of propellant. For example, bursts of a compressed gas escape from a nozzle to produce a force on the telescope. Spinning reaction wheels. Reaction wheels require an on-board motor – when the rotation speed of the wheel is changed, the telescope begins to rotate in the opposite direction. TELESCOPES Reflecting telescope optics Prime Focus & Newtonian Telescope The prime focus configuration has the advantage of both simplicity and minimum light loss (there is only one reflection), but has some limitations. Newtonian design provides a more convenient access to the focus. TELESCOPES Reflecting telescope optics Cassegrain & Gregorian reflectors (a) Cassegrain mirror configurations. (b) Gregorian mirror configurations. Tube of the Cassegrain will be much shorter than a Newtonian of the same focal length. TELESCOPES Reflecting telescope optics Cassegrain & Gregorian reflectors 𝛽 is positive if the focus is behind the primary, and that 𝑚 and 𝑘 are both positive for a Cassegrain and negative for a Gregorian. A telescope designer may choose only two of these parameters, with the third given by TELESCOPES Reflecting telescope optics Cassegrain & Gregorian reflectors The overall focal length, f, is positive for a Cassegrain and negative for a Gregorian. TELESCOPES Reflecting telescope optics Aplanatic two-mirror telescopes The classical versions of the Cassegrain and Gregorian just described begin with the assumption that the primary must be a paraboloid. The resulting telescopes suffer from coma and astigmatism. One can, in fact, choose any conic constant for the primary, and find a secondary shape that preserves freedom from SA. To choose any conic constant for the primary, and find a secondary shape that preserves freedom from SA. The proper choice K1 and K2, in fact, will remove both SA and coma, producing an aplanatic reflecting telescope. TELESCOPES Reflecting telescope optics Aplanatic two-mirror telescopes The aplanatic Cassegrain is called a Ritchey–Chretien or R–C, and consists of a hyperbolic primary and hyperbolic secondary. The aplanatic Gregorian has no special name and utilizes an ellipsoidal primary and secondary. The required conic constant; Remember; TELESCOPES Reflecting telescope optics Nasmyth and coude foci (a) The Nasmyth configuration. Light from the secondary mirror (2) is redirected by a tertiary flat (3) along the hollow altitude axis and reaches a focus above the Nasmyth platform (4). The platform rotates around the azimuth axis (5) as the telescope points and tracks. (b) A coude configuration. Light from the secondary is redirected by flat 3 to a series of flats (4–5– 6) that bring the beam to the polar axis. Similar arrangements can direct the beam along the azimuth axis of an altazimuth. TELESCOPES Reflecting telescope optics Schmidt telescopes All telescope designs discussed so far give good images over relatively small fields of view – diameters of slightly less than one degree of arc for an RC, and perhaps up to three degrees with a corrector lens, depending on the focal ratio. The Schmidt telescope produces good images over a much larger field – six to eight degrees. TELESCOPES Reflecting telescope optics Schmidt telescopes The Schmidt telescope exploits the symmetry of a spherical mirror to avoid off-axis aberrations. It consists of three elements: a spherical primary mirror an aperture stop located at the center of curvature of the primary a refracting corrector plate designed to remove spherical aberration. TELESCOPES Reflecting telescope optics Schmidt telescopes The aperture stop insures there can be no distinction between on-axis and off-axis sources: wave fronts from different directions will illuminate slightly different parts of the mirror, but since it is a spherical mirror, all will experience an identical change in wavefront shape upon reflection. Because of the aperture stop, the chief ray from every source always passes through point C, the center of curvature. This means that points B and V in the figure always coincide, and there can be therefore no third-order coma or astigmatism. TELESCOPES Reflecting telescope optics Schmidt telescopes The aperture stop of an imaging system is the optical element that limits angle of rays passing through the system from a source on the optical axis. For an off-axis object, the chief ray is the ray that passes through the center of the aperture stop, and the marginal rays are those that pass through the edge of the aperture stop. The size of the aperture stop determines the light-gathering capability of an instrument, and thus the brightness of its images. TELESCOPES Reflecting telescope optics Schmidt telescopes (a) shows the arrangement of aperture stop, corrector plate, primary mirror, and focal surface; (b) shows how the aperture stop located at the center of curvature results in identical optics for beams from different directions. The corrector plate, located in the plane of the aperture stop, is designed to remove SA. TELESCOPES Reflecting telescope optics Schmidt telescopes a) shows a plate whose power decreases radially, starting with zero power at center; b) shows a plate with positive power at center, decreasing to zero at 0.866 fractional radius minimizes the chromatic aberration introduced by the corrector plate. It is possible to further minimize chromatic aberration by using a twoelement achromatic corrector. Unlike the spherical mirror, the corrector plate does have an optical axis, and introduces some off-axis aberrations, which are of concern in systems with very fast focal ratios (f/2). The refracting corrector plate limits apertures to modest values, and the focal surface is inaccessible to a human observer, so the instrument is often called a Schmidt camera. c) shows the actual cross-section of a corrector with shape (b) for an f/3 system. TELESCOPES Reflecting telescope optics Schmidt - Cassegrain telescope; A catadioptric telescope is one that combines full-aperture refracting (dioptric) and reflecting (catoptric) elements. usually at a focal ratio near f/10. Such systems generally have a spherical primary and elliptical secondary, with the aperture stop and corrector placed near the focus of the primary (in Figure 6.8, α ≈ 𝟎 and 𝒎 = 𝒇/𝒇𝟏 ≈ 𝟓). They usually have D=DP and produce fields of view of around 1o. TELESCOPES Reflecting telescope optics - Large mirrors An example; Hale 5m teleskop has weight of 14 tons. The mirror-maker pours a pool of molten glass into a cylindrical mold with a diameterto-thickness ratio of about 6:1 Large modern telescopes have used three different types of mirror: Honeycombed monolithic mirrors are an extension of the classical design, but with reduced mass and stiffness. Fabrication of these mirrors is greatly facilitated by a technique known as spin casting. The idea here is to rotate the glass mold at a constant rate, so that the centrifugal effect forms the surface of the molten glass into a paraboloid. Segmented mirrors are mosaics of several easily fabricated smaller mirrors arranged to produce a single large aperture. 10-meter Keck telescopes on Mauna Kea, for example, each consist of 91 hexagonal segments. Each 1.8-meter segment is held in place by an active control system that constantly adjusts mirror positions to compensate for misalignments. TELESCOPES Reflecting telescope optics - Large mirrors Thin meniscus mirrors have a diameter-to-thickness ratio of something like 40:1. They are usually spun-cast in a bowl-shaped mold. Unlike the honeycombed monolith or the individual segments of a mosaic, these mirrors have no ability to retain their shapes unless supported by an active cell. TELESCOPES Adaptive optics - Atmospheric wavefront distortion Distortion of wavefronts by a turbulent atmosphere. The figure shows plane wavefronts incident on turbulent layers, and the distortion that results. Solid contours indicate lower temperatures; dotted contours indicate higher temperatures. The distortion in (b) is much greater than in (a). TELESCOPES Adaptive optics - Atmospheric wavefront distortion Adaptive optics (AO) aims at removing wavefront distortions by inserting one or more adjustable optical elements into the path between source and detector. In practice, the adjustable elements are usually reflective, and usually located near the telescope focal plane. The shape of the reflecting surface is adjusted to exactly cancel distortions generated by atmospheric turbulence. TELESCOPES Adaptive optics - Atmospheric wavefront distortion Adaptive optics (AO) aims at removing wavefront distortions by inserting one or more adjustable optical elements into the path between source and detector. In practice, the adjustable elements are usually reflective, and usually located near the telescope focal plane. The shape of the reflecting surface is adjusted to exactly cancel distortions generated by atmospheric turbulence. TELESCOPES Adaptive optics - Atmospheric wavefront distortion Adaptive optics (AO) aims at removing wavefront distortions by inserting one or more adjustable optical elements into the path between source and detector. In practice, the adjustable elements are usually reflective, and usually located near the telescope focal plane. The shape of the reflecting surface is adjusted to exactly cancel distortions generated by atmospheric turbulence. TELESCOPES Adaptive optics - Atmospheric wavefront distortion Adaptive optics (AO) aims at removing wavefront distortions by inserting one or more adjustable optical elements into the path between source and detector. In practice, the adjustable elements are usually reflective, and usually located near the telescope focal plane. The shape of the reflecting surface is adjusted to exactly cancel distortions generated by atmospheric turbulence. TELESCOPES Adaptive optics - Atmospheric wavefront distortion Adaptive optics (AO) aims at removing wavefront distortions by inserting one or more adjustable optical elements into the path between source and detector. In practice, the adjustable elements are usually reflective, and usually located near the telescope focal plane. The shape of the reflecting surface is adjusted to exactly cancel distortions generated by atmospheric turbulence. wavefront reconstruction ÖNEMLİ HATIRLATMA: Bu sunum İ.Ü. İstanbul Fen Fakültesi Lisans öğrencilerinin eğitimlerine ücretsiz katkı sağlamak amaçlı hazırlanmış olup, bilimsel kaynak olarak gösterilemez, ücretlendirilemez, izinsiz kaydedilemez, kullanılamaz, çoğaltılamaz ve ticari bir ürün haline dönüştürülemez. İLGİNİZ ve SABRINIZ İÇİN TEŞEKKÜRLER… Bu sunum İstanbul Tıp Fakültesi öğrencilerinin eğitimlerine ücretsiz katkı sağlamak amaçlı hazırlanmış olup, bilimsel kaynak olarak gösterilemez, ücretlendirilemez, izinsiz kaydedilemez, kullanılamaz, çoğaltılamaz ve ticari bir ürün haline dönüştürülemez.