Eye Lecture PDF
Document Details
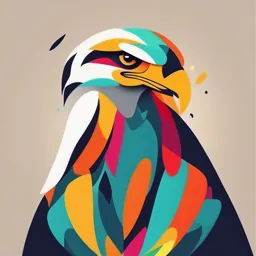
Uploaded by RoomierCanyon
Boston University
Béla Suki
Tags
Summary
This lecture explores structure-function relationships in the human eye, focusing on the cornea and sclera. The lecture covers evolution, mechanisms of the eye, and related questions. It also includes key historical figures in the field, such as Charles Darwin.
Full Transcript
Structure-function relations in the eye: the cornea and the sclera Béla Suki Department of Biomedical Engineering Boston University Boston, MA Introduction Many interesting phenomena are associated with visi...
Structure-function relations in the eye: the cornea and the sclera Béla Suki Department of Biomedical Engineering Boston University Boston, MA Introduction Many interesting phenomena are associated with vision. These often involve physics, physiology, neuroscience and psychology. For example, there is a very interesting adaptation of vision in the dark. What do you see from the corner of your eye? How is the light processed in your eye? How does the brain process visual information? How do you see colors and how do you distinguish shapes? How does your eye ball survive a punch? Is mechanics important for the eye? Who created the eye? “The human eye is an exquisitely complicated organ. It acts like a camera to collect and focus light and converts it into an electrical signal that the brain translates into images. But instead of photographic film, it has a highly specialized retina that detects light and processes the signals using dozens of different kinds of neurons. So intricate is the eye that its origin has long been a cause célèbre among creationists and intelligent design proponents, who hold it up as a prime example of what they term irreducible complexity—a system that cannot function in the absence of any of its components and that therefore cannot have evolved naturally from a more primitive form.” T.D. Lamb, Scientific American, July 2011. Darwin on the evolution of the eye Charles Darwin (1859) explained: “If numerous gradations from a perfect and complex eye to one very imperfect and simple, each grade being useful to its possessor, can be shown to exist. and if any variation or modification in the organ be ever useful to an animal under changing conditions of life, then the difficulty of believing that a perfect and complex eye could be formed by natural selection, though insuperable by our imagination, can hardly be considered real”. Evolutionary view of the eye ‘Nothing in biology makes sense except in the light of evolution’ (Dobzhansky, 1973). This insight is fundamental if we are “trying to comprehend the nature of our rod and cone photoreceptors, and the organization of our retina. Unless we understand how these cells and structures arose, through hundreds of millions of years of evolution, we have little prospect of making sense of their morphological and molecular structure, or being able to answer the recurring conundrum ‘Why does the retina do it this way?’. In addition to providing a rationale for the arrangement of our retina, a study of the evolution of our eye and its cones and rods is immensely satisfying, in offering potential answers to questions such as ‘How and when did our eyes originate?’ and ‘Why should we and all other vertebrates possess eyes so different from those of (for example) insects?’. “ (Lamb, 2013). The structure of the eye Eyes are organs that detect light and convert it into electro-chemical impulses in neurons. In higher organisms the eye is a complex optical system which collects light from the surrounding environment, regulates its intensity through a diaphragm, focuses it through an adjustable assembly of lenses to form an image, converts this image into a set of electrical signals, and transmits these signals to the brain through complex neural pathways that connect the eye via the optic nerve to the visual cortex and other areas of the brain. Eyes with resolving power have come in ten fundamentally different forms, and 96% of animal species possess a complex optical system. Description Cornea: This is the front transparent surface of the eye. The cornea functions as a lens and together with the eye lens it will focus the image onto the retina. It has a thickness of 0.5 to 1 mm and is 12 mm in diameter approximately. Conjunctiva: This is a mucous membrane that lines the inner surface of the eyelids and the visible part of the eye-ball. The conjunctiva contains small sebaceous glands producing the mucus of the lacrimal film. Pupil or Iris: The iris, containing pigments, determines the color of the eye. This thin membrane works as a diaphragm and regulates the light entry in the eye. In full light the pupil contracts thus diminishing the amount of light falling onto the retina; inversely, in darkness the pupil expands in order to absorb as much light as possible. Eye Lens: The lens is located immediately behind the iris. The lens, together with the cornea, is focusing the light onto the retina and consequently is creating a sharp image. The lens has the capability to change shape and thus to bend the light rays to greater or lesser extent. This enables us to see sharply in the distance or up close. The lens is at rest when one looks in the distance. When reading, the lens will slightly change shape and will focus on the image nearby. This mechanism is called accommodation. From the age of 45 onwards this accommodation will slowly grow more difficult due to the ageing of the lens. This ageing causes the lens to be less flexible and deformable. This is the reason why most people may need reading glasses at the age of 45. Retina: The retina is the visual organ. It forms the inner lining of the eye. The image is captured by the eye and projected onto the retina and it is then transmitted to the brain via the optic nerve. The center of the retina (macula) is the most sensitive part and captures details. The peripheral retina takes care of the peripheral field of vision which allows us to see objects sideward. Macula: This is the center of the retina. The light rays are focused onto this part. This area of the retina contains the highest concentration of photo receptors and consequently is key to the perception of details. The fovea (the center of the macula) is the most important part of the retina. Vitreous Body: The vitreous body fills the space between the lens and the retina. It is a jelly-like substance contained by a thin membrane. It is composed of 98% water and 2% macro molecules which give the substance its jelly-like aspect. Over the years the vitreous body will loose its elasticity and will gradually shrink. This is a normal ageing process. Moreover, in time the vitreous body will become less transparent and the fibers will gradually grow more visible. Little black spots will then appear into the patient's field of vision. If the vitreous body shrinks any further its rear part will detach from the retina. Sclera: Thanks to the sclera the eye is white and ball shaped. It is made of strong connective tissue, due to which the eye is very robust. Processing of light Light enters from the top and passes through the neural structure to the photoreceptors, which are the rods and cones. Just behind the rods and cones is the retinal pigment epithelium (RPE). Its major function is to supply the metabolic needs of the photoreceptors. The rods respond to dim light, whereas the cones contribute to vision in bright light and in color. Light transduction The initial step in the translation of light from a source into an electric signal propagating to the visual cortex takes place in the photoreceptors in a process known as transduction. This consists of the cis-trans isomerization of the carotenoid chromophore, which leads to a transient change in the membrane potential of the cell. The result consists of a graded response, seen as a hyperpolarization of the photoreceptor, and an electrotonic current linking the outer and inner segments. A photoreceptor is capable of transducing the energy of a single photon into a pulsed reduction of axial current of about 1 pA lasting about 1 s. The photoreceptor serves as a photomultiplier with an energy gain of some 100 times. Molecular mechanism of light transduction (A) Schematic anatomy of representative vertebrate rod. (B) Major proteins and mechanisms in vertebrate rod transduction. Abbreviations: hn, light; Rh*, activated form of the photopigment rhodopsin; GTP, guanosine triphosphate; GDP, guanosine diphosphate; cGMP, guanosine 30,50-cyclic monophosphate; GMP, guanosine monophosphate; PDE, guanosine nucleotide phosphodiesterase; RK, rhodopsin kinase; RGS complex, group of three proteins including RGS9 which accelerate the hydrolysis of GTP by the alpha subunit of transducin; and Pi , inorganic phosphate. (Fain et al, Current Biology, 2009). Cell to cell communication The photoreceptors synapse with a horizontal cell and bipolar cell in what is known as the triad. The signal transmitted via the horizontal cell results in the inhibition of neighboring receptor cells and, hence, an enhancement in contrast. The bipolar cell responds electrotonically with either a hyperpolarization or depolarization. The bipolar cells synapse with ganglion cells. This synaptic connection, however, is modulated by the amacrine cells. These cells provide negative feedback and thus allow regulation of the sensitivity of transmission from the bipolar to ganglion cells to suitable levels, depending on the immediate past light levels. At the ganglion cell the prior (slow) graded signals are converted into an action pulse that can now be conveyed by nerve conduction to the brain. The magnitude of the slow potential is used by the ganglion cell to establish the firing rate, a process sometimes described as converting from amplitude modulation to pulse-frequency modulation. Automatic mechanisms The iris adjusts its size automatically according to the intensity of light due to the muscles attached to it. In a dark place, the iris widens to take in as much light as possible. When light increases, it shrinks to limit the light entering the eye. This automatic adjustment system works as follows: As light comes to the eye, nerve impulses travel to the brain with information about the brightness of light. The brain immediately sends back a signal to the muscles to adjust the iris. Another eye mechanism working parallel to this structure is the lens. The lens focuses the light coming to the eye onto the retina at the back of the eye. This is done via the muscles around the lens allowing light rays coming to the eye from different angles and distances to always be focused on the retina. All these systems are smaller than the mechanical devices designed by the use of the latest technology in order to imitate the eye. Even the most advance artificial imaging system in the world remains simple compared to the eye especially if we consider how the image capture is enhanced by brain processing. Nevertheless, the eye is not a perfect organ, it shows signs of tinkering by evolution. Evolution of the camera eye Euglena Nautilus hagfish Lamprey Mammals Evolution of the eye and embryonic development T.D. Lamb, Scientific American, July 2011. The eye is not perfect These defects are by no means inevitable features of a camera style eye because octopuses and squid independently evolved camera-style eyes that do not suffer these deficiencies. Indeed, if engineers were to build an eye with the flaws of our own, they would probably be fired. Considering the vertebrate eye in an evolutionary framework reveals these seemingly absurd shortcomings as consequences of an ancient sequence of steps, each of which provided benefit to our long-ago vertebrate ancestors even before they could see. The design of our eye is not intelligent—but it makes perfect sense when viewed in the bright light of evolution. T.D. Lamb, Scientific American, July 2011. The human and the octopus eye Vertebrates and octopuses developed the camera eye independently. In the vertebrate version the nerve fibers pass in front of the retina, and there is a blind spot where the nerves pass through the retina. In the vertebrate example, 4 represents the blind spot, which is notably absent from the octopus eye. In vertebrates, 1 represents the retina and 2 is the nerve fibers, including the optic nerve (3), whereas in the octopus eye, 1 and 2 represent the nerve fibers and retina respectively. The above structural difference may be accounted for by the origins of eyes; in cephalopods they develop as an invagination of the head surface whereas in vertebrates they originate as an extension of the brain. Macroscopic structure of the cornea Meek, KM, The cornea and sclera, In Collagen: Structure and Mechanics, Springer, 2008 The cornea is the clear anterior section of the eye ball and forms 15% of the outer layer of the eye the remaining 85% being the sclera. The cornea is continuous with the white sclera. The radius of curvature R is greater on the front surface. The cornea also has a higher refractive index than the air and the aqueous humor, a gel-like viscous fluid, behind it. From front to back the cornea has 5 layers: 1) the epithelium (ep) containing about 5 layers of cells, 2) the Bowman’s membrane (b) which is an acellular region with collagen I, III, V and VII, 3) the stroma (s) is the main connective tissue taking up 90% of the cornea, 4) the Descemet’s membrane (d), a modified basement membrane, and 5) the endothelium (e) which is a single layer of cells that helps hydrating the corneal tissue. There is a fine superficial tear film sitting on the epithelial layer. Main functions of the cornea and sclera The cornea and the sclera provide a tough outer shell of the eye ball. Their major function is to protect the content of the eye from infections. The cornea is essentially a connective tissue and hence it has mechanical function. It has to be able to withstand mechanical injury from outside and contain the ocular pressure from inside. This is achieved by collagen fibrils embedded in a gel-like ground substance. The cornea also has to be precisely curved. The cornea must also have a smooth surface. It is coated by a 4-7 mm thick tear film that transports metabolic products and prevents it from drying. Optically, the tear film provides a very smooth surface together with the precise curvature of the cornea they form a functional lens. About two thirds of the focusing occurs at the air-tear film interface. The cornea is where light enters the eye and hence it must be transparent to visible light. These mechanical and optical functions are simultaneously met by a efficient organization of collagen fibrils in the cornea. Lamellar structure in the stroma In the stroma, collagen fibrils form structures called lamellae. Lamellae run parallel to the surface of the cornea but directed in various angles forming a plywood-like structure. They are typically 2 mm thick and up to 0.2 mm wide as shown in the EM image. Sometimes the lamellae split (see black arrowhead). The stroma contains cells called keratocytes which are flattened fibroblasts that maintain the collagen structure and respond to injury by repair. Meek, KM, The cornea and sclera, In Collagen: Structure and Mechanics, Springer, 2008 3-dimensional reconstruction The image was obtained by second-harmonic TL imaging. A) shows a cross-sectional slice through 3D data set showing the outer 1/3 of the cornea with IL transverse oriented collagen bundles (TL). The middle 1/3 section has intervowen lamellae (IL) and the inner 1/3 shows orthogonally oriented OL lamellae (OL). B) 3D reconstruction of cross-section of cornea showing multiple transverse collagen (arrow). The inset depicts a transverse lamellae just above the Bowman’s membrane (asterisk) underlying the epithelial layer. Bar 50 mm, bar in inset 20 mm. Meek, KM, The cornea and sclera, In Collagen: Structure and Mechanics, Springer, 2008 Nanoscopic structure The lamellae are composed of narrow, uniform diameter collagen fibrils that run parallel to the lamellae that confers radial strength to the cornea. No fibril runs through the full thickness of the stroma. The fibril diameters are tightly regulated around 31 nm and they increase with age to 34 nm. Fibril diameters do not vary with depth but suddenly increase to 40 nm at about 4 mm from the center of the cornea. The center to center distance between fibrils is constant 57 nm in the 4 mm center and rises to 62 nm outside the center. The TE image shows the cross-section of 5 lamellae in human cornea. Fibrils in adjacent lamellae make large angles with one another. The interfibrillar space is filled with PGs (arrow). Bar is 100 nm. Meek, KM, The cornea and sclera, In Collagen: Structure and Mechanics, Springer, 2008 Cross-linking Fibrils are mostly made of type I collagen and a small amount of type V (nucleator) and have an axial stagger similar to other tissues. The fibrils are stabilized by various covalent cross-links such as deH-HLNL, deH-HHMD and HHL. The schematic drawings show the cross-linking and packing of collagen. A collagen molecule is represented by a cylinder with 4 regions (1-4) and an extra 38 nm long segment at the C terminus. The HHL cross-link is represented by a T and the deH-HHMD cross-link by an H. The top shows 3 collagen molecules with both cross-links and the bottom shows a cross-section at the C terminus. Type V collagen is the black circle. This arrangement is similar to that in the skin but the fibrils are more hydrated than skin or tendon or the sclera. The molecular spacing is 1.8 nm and this hydration reduces light scattering by fibrils. Meek, KM, The cornea and sclera, In Collagen: Structure and Mechanics, Springer, 2008 Composite fibril structure Proposed model of the type I-type V composit fibril. The N terminus of type V extends through an intermolecular hole to the surface of the fibril. Type I is light and type V is dark shaded. The bottom shows the type I as transparent so that type V can be seen inside the fibril. The extension of type V produces a steric hindrance to fibril-fibril distance as well as limits fibril diameter. Meek, KM, The cornea and sclera, In Collagen: Structure and Mechanics, Springer, 2008 Composition of the stroma The table shows the approximate composition of the stroma with the keratocyes containing about 11% of water. The collagen is mostly type I (58%) and type V (15%), type VI (24%) and a bit of types XII and XIV. Type VI forms 100 nm periodic filaments and due to its interaction with type I, it has been suggested as a bridging structure and hence contributing to mechanical stability. Types XII and XIV are FACIT collagens and mostly found on the surface on type I fibrils. The interfibrillar space is filled up with PGs that contain GAGs and hence a lot of water. The GAGs are keratan sulphate, chondroitin sulphate and dermatan sulphate. They associate with decorin and biglycan. Corneal shape For a thin walled membrane, we can apply LaPLace’s equation: Dp = T(1/R1 + 1/R2) Where the left hand side is the pressure drop through the membrane carrying a tension T and R1 and R2 are the principal radii of curvature. The stress inside the membrane can be averaged through the thickness h to give tension: T = h. Thus, the average stress in the stroma is s = Dp R/(2h) Where Dp is referred to as intraocular pressure, 1.5-3 kPa, and R is the average radius of curvature. This gives an average stress of 60 kPa. This is what the fibrils need to be able to support in all direction which is why the lamellae have varying directions. But this is also what maintains the shape and hence focus of the cornea. The top drawing shows that the curvature of the cornea is greater than that of the sclera and hence at the interface there is a change in curvature and hence stress in the cornea which is why the collagen fibril diameters change. The bottom drawing shows that the optical zone of the cornea occupies the middle 2/3 of the cornea. Meek, KM, The cornea and sclera, In Collagen: Structure and Mechanics, Springer, 2008 X-ray scattering to determine structure Using X-ray scattering, the orientation of parallel fibrils can be determined. The position of the scattering maxima is characterized by the scattering angle 2q, which depends on the lateral center to center spacing of the cylinders (either collagen molecules or fibrils) and the rotation angle j which depends on the orientation of the cylinders. Thus, both can be determined. Meek, KM, The cornea and sclera, In Collagen: Structure and Mechanics, Springer, 2008 X-ray scattering of the cornea Wide angle X-ray scattering pattern from the center of the human cornea. The equatorial reflection corresponds to an intermolecular spacing of 1.6 nm (arrow) and has 4 maxima that arise from excess collagen in the vertical and horizontal directions. Meek, KM, The cornea and sclera, In Collagen: Structure and Mechanics, Springer, 2008 The orientation map The preferred orientation throughout the cornea and part of the sclera averaged through the thickness and mapped onto the human eye. The colors represent scaling down to fit the same grid. There is an orthogonal orientation in the middle of the eye, the central 8 mm optical zone. It has been suggested that these orthogonal lamellae help take up the mechanical load of the extraocular muscles. Note the tangential-like bigger (green) lamellae orientations. This is where the curvature changes and the fibril diameters increase. Meek, KM, The cornea and sclera, In Collagen: Structure and Mechanics, Springer, 2008 Biomechanics of the cornea Corneal mechanical behavior is heavily influenced by stromal collagen architecture. Since collagen fibrils are strongest axially, lamellar anisotropy may be expected to confer direction dependency on the corneal elastic modulus. From a clinical point of view, the stress-bearing ability of the cornea is likely to be important in determining the topography of the anterior corneal surface and its response to disease, refractive surgery, and changes in intraocular pressure (IOP). However, to what degree, if any, collagen fibril organization influences the precise shape that the cornea adopts in response to a given IOP is an open question. Retinopathy, globe enlarged (RGE) is an autosomal recessive disease of chickens arising from an in-frame 3-bp deletion in the cone β-transducin gene and is characterized clinically by progressive retinal degeneration and blindness by 1 month posthatch. The study by Boote et al. (Invest Ophthalmol Vis Sci. 2011) was designed to compare the behavior of normal and RGE chicken corneas under varying simulated IOP, using corneal inflation. In addition, second harmonic generation (SHG) multiphoton microscopy was used to compare the in-plane appearance of stromal lamellae. Biomechanical measuring apparatus (A) Schematic showing main components of the corneal inflation rig. (B) Photograph of apparatus. The average values of thickness (±SD) were 334 ± 34 μm (normals) and 309 ± 15 μm (RGE). Specimens were tested on a custom-developed corneal inflation rig designed to assess corneal deformation under simulated IOP. It pressurizes the posterior cornea, while the deformation of the anterior surface is simultaneously measured. The pressure chamber was filled with saline at 37°C and connected to a reservoir whose height was computer controlled to set the applied pressure. A 1 μm accuracy laser displacement sensor aligned normal to the corneal apex, and two digital cameras positioned in the corneal plane measured the initial corneal radius of curvature. Stiffness can be obtained using thin shell deformation analysis. Boote et al. Invest Ophthalmol Vis Sci. 2011 Pressure-radius curves Comparison of inflation behavior between normal and RGE chicken corneas, showing for each group pressure-apical rise data for the (A) highest/lowest stiffness corneas and (B) the average of all corneas. Error bars, SD. From pressure- rise data RGE corneas appeared considerably different. Is the RGE cornea softer? Boote et al. Invest Ophthalmol Vis Sci. 2011 Stress-strain curves and stiffness Left: Average stress-strain behavior of normal and RGE chicken corneas. Error bars, SD. Once tissue thickness and geometry are accounted for, RGE corneas displayed significantly increased material stiffness compared with normal controls. Right: Tangent modulus (E), the gradient of a tangent line drawn at a given point on the stress-strain curve, plotted as a function of applied stress for normal and RGE chicken corneas under the fourth cycle of posterior pressure loading. Error bars, SD. A 30–130% increase in E, commonly considered a direct measure of tissue stiffness, is observed in RGE corneas compared with controls. Boote et al. Invest Ophthalmol Vis Sci. 2011 Structure comparison In-plane SHG collagen signals from the normal and RGE chicken cornea at stromal depths of 225 μm (posterior) and 50 μm (anterior). Bar, 50 μm. An orthogonal collagen straight latticework is evident in the posterior stroma (A, C, E, G). These structures appear wavier in the anterior stroma, while still retaining their lattice-like arrangement with 90° cross-angles (B, D). The characteristic fanlike structures in the peripheral anterior stroma of the normal cornea (F) are notably absent in RGE (H). Boote et al. Invest Ophthalmol Vis Sci. 2011 Aging cornea Relationship between corneal Young’s modulus (E) and age. Filled circles: measurements from unpaired corneas and one randomly selected measurement from paired specimens. Open circles: measurements obtained from fellow eyes. The line of best fit for first eyes (solid line) has the equation E=0.0031*age + 0.21; R2=0.74. Dashed lines : 95% confidence limits; dotted lines : measurement prediction boundaries. Cartwright et al. IOVS, 2011 Why is the cornea transparent? The transparency of the ocular media of the eye was originally ascribed to the homogeneous nature of their constituent elements and the absence of opaque structures, especially blood vessels. Measurement of refractive index has been attempted in isolated components of the cornea. Values of 1.547 were found for dry collagen and 1.342 for pressure- extracted corneal fluid derived from the interstitium. The question has arisen as to just how transparency is attained in a heterogeneous medium of fibers embedded in a matrix of different refractive index. Transmission of visible light through the cornea Experimental values for the fraction of light transmitted through normal and edematous rabbit corneas as a function of wavelength. The ratio of the thickness of the edematous corneas to normal thickness values and the number of corneas used for each curve are given in the key. (From Farrell RA, et al. J Physiol233:589, 1973.) Light scattering Maurice’s theory In 1957, Maurice assumed that there was a sharp difference in refractive index between collagen fibrils and ground substance, based on measurements of birefringence, and indicated that if individual collagen fibrils scattered light independently of one another, the stroma would be opaque. His theory asserted that, since the cornea is transparent, a phase relationship must exist between electric fields emanating from individual fibers, resulting in destructive interference of scattered wavelets which limited the intensity of light scattering. He believed that this distribution of fibrils was the only arrangement that could ensure transparency on a simple theoretical basis and was in accordance with the striking electron micrographic appearances. The theory assumed a high degree of uniformity in size of corneal fibrils and a high degree of regularity in their arrangement as a lattice. However, the fibers of Bowman's zone are neither parallel nor disposed in a lattice and yet it scatters less light than the rest of the corneal stroma. It may be concluded that a lattice arrangement of collagen fibrils is not a necessary condition for corneal transparency. Experiments have indicated that fibril diameters could vary considerably and that a regular crystalline structure is not necessary to enable transparency provided that the fibril diameters remain a small proportion of the wavelength of light. Current understanding of transparency Current understanding of corneal transparency favors a short-range ordering of fibril structure such that fluctuations in refractive index fall well within the minimum dimensions of the wavelength of light thus enabling a high degree of transparency to the visible portion of electromagnetic radiation. The highly aligned fibril structure probably has more to do with tectonic function by allowing the greatest number of fibrils per unit volume for optimal strength and in helping to maintain regularity of the surface curvature of the cornea. Direct Summation of Fields (DFS) In 1986, Freund et al. (Appl Opt 1986; 25: 2739–2746) published a method to compute light scattering from the cornea. The technique can be used to predict transmission by an arbitrary short-range order distribution of different-sized fibrils. A full account of the approach, called the direct summation of fields (DSF) method, is found in the original papers. It is a statistical technique in which the scattering from each individual fibril is computed, then the effects of interference are included and summed for the whole tissue using a method called ensemble averaging. In this method, transmission is computed as a function of wavelength, ignoring the lamellar structure of the stroma and also the presence of stromal cells. With these assumptions in mind, however, the DSF method has been tested and found to give reliable results in a number of situations. Experiment vs theory Differential scattering cross section per fibril per unit length (Eq. 19): The transmittance is: T=exp{-Nσx} where N is the number density of fibrils with cross section σ and x is the path length of light, q is the wave number vector. Sensitivity analysis of light transmission Theoretical effects of varying individual parameters as predicted by the summation of scattered fields model. The predicted transmission of light is plotted against wavelength. The number by each curve represents the values of the parameters being varied, in units of nm (a), mm-2 x 10-2 (b), and mm (c). Transmission is reduced if 1) order in the spatial arrangement of the fibrils is destroyed; 2) fibril diameters increase; 3) fibril number density increases; 4) there is an increased refractive index imbalance between the hydrated fibrils and the extrafibrillar matrix; 5) corneal thickness increases. Secondary waves Secondary waves from a single (a) and pair (b) of collagen fibrils. The right hand side insets are a magnified view of the fibril arrangement contained in the rectangle in the main panels. The bar, 1 μm, is in common to (a) and (b). Secondary waves The effect of increased fibril diameters on light Secondary waves from a collagen fibril distribution presenting short-range order. Primary incoming light is travelling from left transmission. Secondary waves from the same collagen fibril distribution shown left. As before, primary incoming to right (yellow arrow), with a wavelength of 500 nm. Collagen fibrils in transverse sections are represented by brown circles. light is travelling from left to right (yellow arrow), with a wavelength of 500 nm. Collagen fibrils in transverse All fibrils have the same diameter of about 31 nm, and no sections are represented by brown circles. 20% of the fibrils collagen fibrils can be closer than 62 nm. Only the intensity of were selected at random and their diameter was doubled the secondary radiation arising from the fibrils is shown in to 62 nm. The intensity of the secondary radiation arising blue. No backwards secondary radiation can be seen in the from the fibrils is shown in blue. Backwards secondary figure. Bar 500 nm. radiation is evident in the figure (white arrows). Bar 500 nm. Secondary waves The effect of fibril voids on light transmission. Secondary Secondary waves from a collagen fibril distribution presenting short-range order. Primary incoming light is travelling from left waves from the same collagen fibril distribution shown left. As before, primary incoming light is travelling from left to to right (yellow arrow), with a wavelength of 500 nm. Collagen fibrils in transverse sections are represented by brown circles. right (yellow arrow), with a wavelength of 500 nm. Collagen fibrils in transverse sections are represented by brown All fibrils have the same diameter of about 31 nm, and no circles. Regions devoid of fibrils are now present (lakes). collagen fibrils can be closer than 62 nm. Only the intensity of The intensity of the secondary radiation arising from the the secondary radiation arising from the fibrils is shown in fibrils is shown in blue. Even in this case, backwards blue. No backwards secondary radiation can be seen in the secondary radiation is evident in the figure (white arrow). figure. Bar 500 nm. Bar 500 nm. The avascular nature of the cornea (Cursiefen et al. PNAS, 2006) Vascular endothelial growth factor receptor 3 (VEGFR3) is an angiogenic receptor when expressed on vascular endothelial cells. However, when expressed on epithelial cells, VEGF3 has opposite effects. Indeed, antiangiogenic effect of corneal epithelium critically depends on epithelial VEGFR3. Direct inhibition of epithelial VEGFR3 using ex vivo treatment with neutralizing antibodies diminishes the epithelium’s ability to dampen angiogenesis. B and C) The neovascular response to an inflammatory stimulus is significantly increased after ex vivo treatment with a neutralizing anti-VEGFR3 antibody. Representative segments from CD31-stained corneal flat mounts (C) are compared with corneas receiving epithelial transplants that received ex vivo treatment with control IgG (B). (D) Morphometry. It is thus thought that the lack of vasculature is needed for transparency. This may also have implications for cancer research. Light scattering in swollen cornea A number of pathological or surgical conditions can lead to increased light scattering and cloudy cornea. Pathologically, the cornea can swell when the endothelial cells fail to pump excess fluid out into the aqueous chamber. As fluid enters the cornea, GAGs take up water and inflate the tissue. The left graph shows X-ray scattering-based intermolecular spacing (D) and interfibrillar spaces (+) as a function of hydration H defined as H=weight/dry weight-1. Intermolecular spacing stops increasing above H=1. In edematous cornea, “lakes” are formed that disrupt the ordered fibril structure and spacing (right) such that the irregularities in refractive index can be comparable to half the wavelength which increases light scattering and vision becomes impaired. Meek, KM, The cornea and sclera, In Collagen: Structure and Mechanics, Springer, 2008 Corneal ectasia Ectasia means stretching of an organ beyond its normal limits. In the cornea, progressive non-inflammatory thinning can occur either pathologically or postoperatively. The most common form is keratoconus in which the central portion of the cornea is thin and weak and allows the protrusion of the eye ball. There is a loss of collagen due to enzymatic degradation. A shearing occurs between lamellae and PGs and this weakens the tissue. Corneal edema can also lead to this condition. Too much hydration can lead to a loss of PGs with subsequent reduction in stiffness. Meek, KM, The cornea and sclera, In Collagen: Structure and Mechanics, Springer, 2008 LASIK Laser refractive surgery is now a common method to restore vision in mild to moderate shortsightedness. Laser in situ keratomileusis (LASIK) is a popular treatment with over 30 million treated worldwide. LASIK involves creating a corneal flap and then removing by laser ablation a layer of collagen from the mid-stroma. This flattening increases the focal length of the cornea. The flap is then repositioned without stitches. But this procedure can reduce the stiffness of the cornea and might lead to post surgical ectasia. Meek, KM, The cornea and sclera, In Collagen: Structure and Mechanics, Springer, 2008 Tissue engineering the cornea Attempts have been made to create artificial tissues with similar properties to the native cornea. However, it is very difficult to recreate the subtle properties of the stroma and hence most constructs are not enough strong or not quite transparent. Usually, 3 layers are added only (see figure). Layers with oriented collagen are stacked while changing directions to mimic lamellae. The collagen gels are populated with proper cell types hoping that they produce good cornea. To obtain proper strength, cross-linking is needed. But PGs also play an important role. Adding PGs help with transparency too probably by controlling fibril diameter and spacing. New and promising approaches are to use either artificial ECM populated by stem cells or introducing such cells in decellularized corneal ECM. Meek, KM, The cornea and sclera, In Collagen: Structure and Mechanics, Springer, 2008 Cell-based engineering of cornea Morphology of human corneal cells cultured as monolayers on a plastic substrate. A: Corneal epithelial cells co- cultured with an irradiated 3T3 feeder layer. B: Stromal fibroblasts. C: Corneal endothelial cells. Bar, 100 µm. Macroscopic aspect of the bioengineered cornea. A: Dots written in arial 12 pt font can easily be seen through the bioengineered cornea. B: The bioengineered cornea possesses a slight haze. Fibroblasts cultured in medium containing serum and ascorbic acid secreted their own extracellular matrix and formed sheets that were superposed to reconstruct a stromal tissue. Endothelial and epithelial cells were seeded on each side of the reconstructed stroma. This study demonstrates the feasibility of producing a complete tissue-engineered human cornea, similar to native corneas, using untransformed fibroblasts, epithelial and endothelial cells, without the need for exogenous biomaterial. Proulx et al. Mol Vis. 2010. Summary Despite its apparent complexity, the eye is not designed, it has evolved! Collagen type I is responsible for the gross anatomy as well as mechanical and optical properties of the cornea and the sclera. The cornea contains more organized collagen and it is more hydrated than the sclera. Collagen type V and PGs are likely responsible for the differences in ordered structures between cornea and sclera. The specific structure of the lamellae serves to provide proper stiffness for protection and shape as well as for proper optical properties and transparency. More studies are required for better understanding how the nanostructure of collagen-PGs assembly determine the mechanical and optical properties of the cornea which might help developing viable tissue engineering approaches.