Oral Microbiome Exercise 29 PDF
Document Details
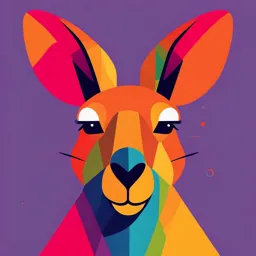
Uploaded by SupportiveAlbuquerque
BCMB 401
Tags
Summary
This document provides an introduction to the oral microbiome, discussing its diversity, composition, and coevolution with humans. It details how the microbiome is acquired, the role biofilms play, and the influence of environmental factors. The document also explores the negative consequences of changes within the oral microbiome.
Full Transcript
INTRODUCTION-------------- The human mouth contains the second most diverse population of microbes in the human body; only the gastrointestinal tract contains a more diverse population. Collectively, over 700 individual species have been identified within the soft tissues of the oral cavity and on...
INTRODUCTION-------------- The human mouth contains the second most diverse population of microbes in the human body; only the gastrointestinal tract contains a more diverse population. Collectively, over 700 individual species have been identified within the soft tissues of the oral cavity and on the surfaces of the teeth; of these, 54% are validly named culturable species, 14% are cu\tur- able but unnamed species, and the remaining 32% are presently unculturable and their presence has only been detected via metagenome analysis. The oral microbiome has coevolved with humans over millions of years and it generally maintains a mutualistic relationship with its human hosts. The oral microbiome receives nutrients, moisture, and a niche, and humans are somewhat protected by microbial antagonism from exogenous species colonization. The acquisition of the oral microbiome commences with childbirth. Studies have indicated that the acquisition and diversity of the oral microbiome heavily depends on how a child arrives into the world-either by natural childbirth or by Cesarean section. Babies delivered vaginally are colonized by bacteria similar to those found in the mother's vagina, while those delivered by Cesarean section become colonized by organisms typically found on the mother's skin. In addition, differences in the composition of the oral microbiome have been documented between breast-fed infants and formula-fed infants. The eruption of baby teeth provides the first hard surface for microbial colonization within a child's mouth and the com- position of the oral microbiome shifts following this event. Likewise, when adult teeth begin to replace baby teeth, the composition changes once again. It is important to remember that the human mouth is not a homogeneous environment and many local microenvironments exist, including highly acidic zones, aerobic zones, anaerobic zones, and zones of high nutrient availability. Finally, the composition of an individual's oral microbiome is influenced by hormonal factors, tobacco use, consumption of refined sugars, consumption of acidic drinks, stress, and of course, an individual's dedication to oral care. Members of the oral microbiome are assembled into biofilm communities that contain both harmless as well as pathogenic members. The bacteria begin to adhere via adhesins to enamel surfaces coated by a proteinaceous film known as the acquired enamel pellicle. The organisms also co-adhere to each other and promote colonization and biofilm formation on the teeth. Formation of the biofilm begins via the process of quorum sensing using both two-component-type systems found in gram-positive bacteria (where small oligopeptides serve as the signal molecule) and the luxS-encoded autoinducer -2 (Al-2) systems found in gram-negative as well as gram-positive bacteria. The biofilm matrix, which contains extra- cellular polymeric substances (EPS), is a source of carbon and energy for the developing population. Other sources of nutrients include host proteins, glycoproteins found in saliva, and gingival crevicular fluid (GCF). Order of colonization of the biofilm community is dependent on the metabolic capabilities of organisms and the nutrients they require for growth. It is not uncommon to find that the products of metabolism from primary feeders become the source of nutrients for the secondary feeders, and sometimes groups of microbes break down a nutrient more efficiently than individual species do. In this way, members of the bio- film avoid direct competition with one another and maintain a stable equilibrium within the biofilm matrix. One consequence of quorum sensing regulation by two-component-systems utilizing small oligopeptides is that these peptides often promote genetic competence. The environment within a biofilm provides an ideal environment for horizontal gene transfer (HGT), including transformation (which requires competent cells), transduction, and conjugation, to occur. Scientists have also determined that DNA can be transferred in gram-negative bacteria by membrane vesicles. The biofilm environment contains a vast metagenome from which organisms can acquire genes and which, in turn, affects their ability to adapt to changing environments. For example, antibiotic-resistance genes (ARGs) are p populous within the oral cavity and acquisition of these genes by antibiotic-susceptible organisms provides an enormous survival advantage for these species. Negative changes in the oral microbiome lead to changes in the oral ecosystem, which in turn lead to oral dysbiosis (a microbial imbalance or maladaptation) in the form of dental cavies and periodontitis. Dental caries has long been associated with an increased consumption of dietary sugar, but how is the oral ecosystem actually impacted? In healthy individuals, certain bacteria in the oral biofilms ferment glucose to yield lactic acid as a primary waste product. Other members of the biofilm community then metabolize lactic acid (a strong acid) as a carbon and energy source, with the resultant waste products being only weak acids. Thus, lactic acid does not accumulate within the biofilm, the pH of the biofilm is only weakly acidic, and fewer carious lesions (cavities) cause decay of tooth enamel. Conversely, in dysbiosis, repeated conditions of lactic acid accumulation within the biofilm alter the composition of the biofilm community and select for strongly saccharolytic, acidogenic, and acid-tolerating bacteria. According to the ecological plaque hypothesis, this results in a loss of microbial diversity and a reduction in the activities beneficial to enamel health within the biofilm. A similar situation occurs in periodontal disease. In those with poor oral hygiene, the accumulation of microbes around the gingival margin induces an inflammatory response from the host immune system. In healthy individuals, the inflammatory response is appropriate, proportional, and resolves without intervention. In a dysbiotic situation, however, some individuals experience an exaggerated, chronic inflammatory response that is ineffective at best and, in worst-case scenarios, can lead to tooth loss. In these patients, the initial inflammatory response leads to an increase in the flow of GCF. GCF is an extravascular, serum like fluid secreted from the underlying connective tissue. It is composed of products of tissue breakdown and components of the immune response, and its production increases during inflammation. GCF delivers beneficial components of the host innate and adaptive immune responses, but it also delivers substrates for proteolytic bacteria living within the biofilm. Chief among these substrates is hemin, which is an essential nutrient required for growth by potentially pathogenic species. Once again, selection for certain species leads to a change in the competitiveness within the oral biofilm community and results in changes in the com- position of the biofilm itself. Additionally, periodontal disease is thought to be one trigger of bacteremia which facilitates spread of bacteria throughout the body from the oral mucosa. Diseases such as cardiovascular disease, rheumatoid arthritis, stroke, inflammatory bowel disease, respiratory tract infections, appendicitis, pneumonia, and diabetes may trace their beginnings to the systemic spread of bacteria from periodontal disease. Today's experiment will examine the diversity and composition of oral biofilms on the teeth and toothbrushes of students in Biology 229. Samples of the oral microbiome will be collected, diluted, and then plated on nonselective as well as selective and differential media to identify various groups and genera of biofilm communities. Tryptic soy agar with 5% sheep blood, often referred to as blood agar, is a general, all- purpose nonselective medium that is used for cultivation of a variety of microbes. The addition of sheep blood provides a source of hemin, but also allows for the detection of the presence of hemolysins based on their effect on red blood cells. This medium is the base for Columbia CNA with 5% sheep blood agar used in Exercise 18, but does not contain the selective com- pounds colistin and naladixic acid. Columbia CNA with s 0 A>Sheep Blood Agar, mannitol salt agar (MSA), and MacConkey agar are all types of selective and differential media. These media were used in Exercise 18, so please refer back to Exercise 18 for a description of the properties of these media. Sabouraud’’s dextrose agar (SDA) was discussed in Exercise 8 and is a medium used for the cultivation of fungi. In this experiment, SDA is used to isolate yeasts from dental biofilms. Pseudocel agar (also known as Cetrimide agar) is a selective medium used for the isolation and identification of Pseudomonas aeruginosa. The medium contains 0.03% cetyltrimethyl- ammonium bromide (cetrimide), a quaternary ammonium cationic detergent that allows for selective inhibition of organisms other than P. aeruginosa by damaging or destroying cytoplasmic membranes in most bacteria. Cetrimide also enhances the production of pyocyanin, a blue-green, water-soluble, secondary metabolite produced by P. aerugiosa. Thus, colonies of P. aeruginosa will appear green to blue-green on Pseudocel agar, which makes this medium a valuable tool in the identification of P. aeruginosa within biofilm communities. Wilkins-Chalgren agar is designed to support the growth of most clinically relevant anaerobic bacteria. This medium is recommended for the isolation of anaerobes from clinical specimens and is a nonselective medium that supplies essential vitamins, other growth factors, arginine, pyruvate, dextrose, hemin and vitamin K3 to enhance the growth of numerous anaerobic species. Incubation of Wilkins-Chalgren Agar under anaerobic conditions allows for the selective growth of strict anaerobes and facultative anaerobes only. Incubation times of Wilkins-Chalgren Agar plates may need to be extended to encourage the growth of anaerobic species. Mitis Salivarius agar is a selective and differential medium used to isolate Streptococcus mitis, S. salivarius, and enterococci from specimens of mixed species and for the differentiation of the viridans streptococci. Peptones and tryptose supply amino acids and nitrogenous com- pounds for bacterial growth, and sucrose and dextrose are sources of carbon and energy. Crystal violet, 1% potassium tellurite, and trypan blue are included as selective agents that inhibit the growth of most gram-negative bacilli as well as gram-positive organisms other than the streptococci and enterococci. Potassium tellurite is reduced by Enterococcus spp., causing the colonies to appear black. S. salivarius utilizes sucrose and forms large colonies with a gum-drop appearance. On the other hand, the enterococci and S. mitis do not hydrolyze sucrose and form smaller colonies on the medium. Trypan blue is absorbed by colonies of the streptococci and causes these colonies to appear blue.