EXCI 360 Neural Control PDF
Document Details
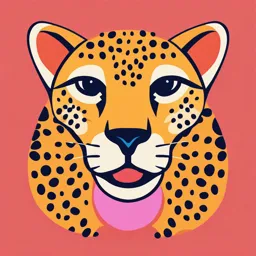
Uploaded by SuperSense9723
Concordia University
Tags
Summary
This document covers the neural and hormonal control of human systems, including the organization of the nervous system at spinal cord, subcortical, and cortical levels. It discusses neuron structures, types of neurons, and glial cells. The notes also examine membrane potentials, synapses, and neurotransmitters.
Full Transcript
EXCI 360 - Neural and Hormonal Control of Human Systems Lecture 1 – The Nervous System Organization of the Nervous System Central Nervous System o Encephalon: Brain, Brainstem, Cerebellum o Spinal Cord Peripheral Nervous System o Peripheral Nerves (sen...
EXCI 360 - Neural and Hormonal Control of Human Systems Lecture 1 – The Nervous System Organization of the Nervous System Central Nervous System o Encephalon: Brain, Brainstem, Cerebellum o Spinal Cord Peripheral Nervous System o Peripheral Nerves (sensory and motor) Autonomic Nervous System o Controls all the autonomic functions of the body ▪ Heart rate, breathing, digestion, urination ▪ Shares fibre tracts with both CNS and PNS o Sympathetic NS ▪ Increases the activity of autonomic functions ▪ Fight or flight o Parasympathetic NS ▪ Decreases the activity of the autonomic functions Functional Organization Sensory Division – Receiver o Receives sensory information → Tactile, Proprioception (muscle), Visual, Auditory, Olfactory, Gustatory, and Vestibular o Reports information about the state of the body and its environment Integrative Division – Processor o Integration of sensory input and motor output to cause desired responses o Provides higher order brain function ▪ Perception, decision making, thinking, attention, language, emotion, and memory Motor Division – Effector o Responds adequately to order of other divisions o Controls bodily activities 3 Major Levels to the Central Nervous System Spinal Cord Level An intersection that conducts sensory and motor signals from the PNS to CNS or CNS to PNS The spinal cord is the centre of processing basic motor and sensory information: o Reflexes can work without the need of other levels o Circuit for motor reflexes: ▪ Stretch Reflex ▪ Golgi Tendon Reflex ▪ Withdrawal Reflex o Circuits for reflexes that control internal organ function ▪ Blood vessels, gastrointestinal, urination Subcortical Level (Lower Brain) Controls Subconscious Body activity o Equilibrium, movement modulation, arterial pressure, respiration, heart beats, feeding reflexes, body temperature, wakefulness, sleep, and hormonal regulation Contains: o Brainstem (medulla and pons) o Mesencephalon o Thalamus o Hypothalamus o Basal Ganglia o Cerebellum Cortical Level (higher brain, Telencephalon, Cerebral Cortex) Superficial layer of the grey matter (2 – 4mm) Cortex rarely functions alone, always in association with lower centers (subcortical and spinal cord) Place of the most complex and sophisticated functions of the nervous system: o Information processing o Memory formation o Decision making o Thoughts and emotions Cortical Level: Cerebral Cortex Divided into 2 hemispheres: Left and Right Each Hemisphere is divided into 4 Lobes: o Frontal Lobe ▪ Area of motor functions (precentral gyrus) ▪ Area of higher mental functions (executive function, attention) o Parietal Lobe ▪ Area of somatosensory functions (postcentral gyrus) ▪ Area of higher mental functions (giving sense to sensory information) o Temporal Lobe ▪ Area of auditory functions ▪ Area of memory formation and storage o Occipital Lobe ▪ Area of visual functions Somatosensory Axis of the Nervous System Somatosensory System o Transmission of somatic (body) information’s from receptors in the periphery of the body (skin, muscle, bone) to the CNS ▪ PNS → CNS Somatic Information is transmitted to: o Spinal cord o Brainstem o Cerebellum o Thalamus o Cerebral Cortex (somatic areas) Motor Axis of the Nervous System Motor System o Responds adequately to the order of integrative and sensory divisions Controls the body motor activities o Skeletal (striated) muscles o Smooth muscles (internal organs, heart, stomach) Neuron Structures Neuron → basic functional unit of the nervous system More than 100 billion neurons in the nervous system Electrical Cell (generates and transmits electricity) Neurogenesis (formation of new neurons) possible but slow Components Cell Body (soma) o Main body of the neuron (nucleus location) o Signal processing Dendrites o Projections of the soma o Signal input Axon o Extends from the soma to the synaptic terminals o Signal outputs (sends signal to other neurons) Types of Neurons - Anatomy Multipolar Neurons o Multiple processes (several dendrites and one main axon) on different sides of the soma o Most common cell type (motoneuron, purkinje cell) Bipolar Neurons o Two processes (one main dendrite and one main axon) on different sides o Found in the retina, inner ear, and olfactory system Unipolar Neurons o One process (dendrite and axon) on the same side o Photoreceptors in the Retina (rods and cones) Pseudo – unipolar neurons o One process (dendrite and axon develop on the same process but on opposite sides o Sensory neurons of the spinal cord Types of Neurons – Functions Afferent Neurons o Send signals to spinal cord and brain o Sensory input = sensory neurons Efferent Neurons o Send electrical signals from the brain and spinal cord to the periphery o Motor output = motor neurons Interneurons o Local circuitry located between sensory and motor neurons (integration) Neurons are interconnected o Each neuron can be connected to up to 1000 neurons = neural network o Synapse → is the point of communication between two neurons Glial Cells They do not participate directly in electrical signaling or synaptic transmission Supportive functions: o Help define synaptic contacts o Maintain signalling abilities of neurons o Defend neurons Types of Glial Cells o Astrocytes ▪ Maintain a good chemical environment for neuronal signalling ▪ Secrete substances that influence the formation of new synaptic connections o Oligodendrocytes ▪ Produce myelin around the axon in the CNS to maintain signaling abilities of the neuron o Schwann Cells ▪ Produces myelin around axon in the PNS to maintain signaling abilities of the neuron o Microglia ▪ Immune function ▪ Consumes cellular debris, bacteria, and dead cells ▪ Secretes signaling molecules that can modulate local inflammation and influence cell survival and death Lecture 2 – Membrane Potentials, Synapses and Neurotransmitters Electrical Potentials of Neurons Specific feature of a neuron (muscles too) → Generate Electricity Neurons have high density of ion channels on their plasma membrane that allow them to control the flow of different ions and generate action potentials (potential difference) Neurons generate electrical potentials (signals) to transmit information throughout the brain and body Four Types of Membrane Potential Resting Membrane Potential (Vm) o At a resting state (no stimulus) o Results from a polarization of the plasma membrane (potential difference) o Always negative ( -90 mv to – 70 mv) Action Potential o Results from transient changes in the membrane potential of a stimulated neuron o Electrical signal that travels along the axons o Long range transmission of information within the nervous system Receptor Potential o Results from transient changes in the membrane potential of a receptor of sensory neurons by external stimuli. (receptor is stimulated) Synaptic Potential o Results from at the communication of signals between neurons at the synaptic contact o Recorded at the postsynaptic neuron by the stimulation of the presynaptic neuron Resting Membrane Potentials At rest the neuron is polarized (the inside (intracellular) of the membrane is more negative than the outside) The recorded potential is between -90 and -70 mv. o This is the resting membrane potential (voltage) Vm is caused by a difference in the concentration of ions outside the cell and inside the cell At the resting state, all voltage gated sodium (Na+) channels and most voltage gated potassium (K+) channels are closed At the Resting State: the Na+/K+ pump transports Na+ ions out of the cell and K+ ions into the cell, keeping the inside of the cell more negative (intracellular fluid is filled with negatively charged proteins) Action Potential (Spike, Impulse, Firing) The action potential is a very rapid shift in the membrane potential from “- “to “+” values and return back to its initial resting potential level “-“ Three Phases: o Depolarization Phase (“- “to “+”) o Repolarization Phase (back to “- “) o Hyperpolarization Phase (below Vm) These phases of the AP are caused by the activation of 2 special types of ion channels on the nerve membrane: o Voltage Gated Sodium (Na+) Channels o Voltage Gated Potassium (K+) Channels Depolarization Phase of AP Initial increase in the membrane potential can be caused by a mechanical, electrical, or chemical stimulation If the membrane potential rises, some of the voltage gated sodium channels will open The Na+ will start to flow inside of the cell (making it more positive inside the cell) The potential rises further to reach the threshold level (65 mV) This causes more sodium channels to open This creates a positive feedback cycle The potential than reaches the overshoot level (above 0 mV) Repolarization Phase of AP Return of the membrane potential toward the resting level (back to negative) This phase is caused by the activation of the voltage gated potassium channels and the deactivation of the voltage gated sodium channels K+ channels are activated when the membrane potential increases above 0mV K+ gates open slowly at the same time as the Na+ gates close o K+ ions flow outside of the cell o Na+ ions are blocked to flow inside o Inside of the cell is more negative o Membrane potential returns towards resting state of -90 mV Hyperpolarization Phase of AP K+ channels remain open for a few seconds after the repolarization state is complete o Excess K+ flows out of the cell (inside is more negative) o The membrane potential becomes more negative than the resting state Back to Resting Stage K+ channels gate close and membrane potential comes back to resting stage (-90 mV) Threshold of AP The threshold of an AP is the level of the membrane potential at which the Na+ channels start to open one another in a positive feedback cycle Preceded by a sub threshold potential (no AP yet) This occurs when the number of Na+ ions entering the neuron becomes greater than the number K+ leaving the neuron o – 55 mv (for a neuron with a Vm = -70 mv) o – 65 mv (for a neuron with a Vm = -90 mv) “All or None” Principle AP is said to be all, or none signal, since either is occurring fully, or it does not occur at all. o No ½ AP, ¼ AP only 1AP If the threshold level is reached the Action Potential occurs The amplitude (voltage) of an AP is NOT dependent of the intensity of the stimulus that it evokes o Distance of peak has nothing to do with the intensity of the stimulus The frequency of firing (number of AP) is dependent on the intensity of the stimulus o More we stimulate the greater number of AP are generated Propagation of Action Potential Unmyelinated Nerve Fibers (adjacent propagation) The Action Potential travels along the neuron’s axons and fibers AP excited adjacent portions of the membrane resulting in propagation of the AP Na+ ions flow to adjacent area: o Open more voltage gated Na+ channels o Increase voltage of adjacent area to reach threshold level o Initiate a new AP in the adjacent area This adjacent propagation if AP occurs in unmyelinated axons Conduction velocity/speed is slow = 0.25 m/s Myelinated Nerve Fibers (saltatory conduction) Axon surrounded by myelin sheath (fatty white substance) The myelin forms wrapping layers around the axon Myelin is produced as an extension of Glial Cells o Schwann cells in PNS o Oligodendrocytes cells in CNS About once every 1-3mm, myelin sheath is interrupted by a Node of Ranvier Ions cannot flow significantly through thick myelin sheath, which insulates nerve fiber Saltatory Conduction in Myelinated Nerve Fibers Action potential can only occur at the Node of Ranvier (where the voltage gated channels are located) and is conducted from node to node Saltatory = jump o Fast conduction: conduction velocity increases 5 to 50 folds → 100 m/s compared to unmyelinated fibers 0.25 m/s o Saltatory conduction conserves energy for axon (little metabolism required to activate ions channels) Conduction Velocity Principles of Action Potential Propagation All or none principal (1) o If conditions are right: ▪ “Full” AP propagation o If conditions not right ▪ “Stop” AP propagation Initial point of generation of AP (2) o Action potential does not begin near the soma or dendrite rather the initial segment of the axon known as the Axon Hillock (where AP begins) Direction of the propagation (3) o Action potential travels from the Axon Hillock towards the Synaptic Terminal of the axon (cannot go in the reverse direction) Functions of Action Potential Transmitting Information o Transfer all sensory information from the PNS to CNS o Transfer all motor information for the CNS to PNS o Transfer information between different parts of the CNS Encoding Information (neuronal language) o Encoding information in the for of AP o The frequency of AP defines the code Rapid Transmission over Distance o Speed of transmission (conduction velocity) depends on the fiber size and if it is myelinated or not Multiple Sclerosis: Blockage of AP Conduction Multiple Sclerosis is an immune deficiency disease that causes the demyelinated of the neuronal fibers in the central nervous system (CNS) Symptoms: o Progressive muscle weakness o Loss of sensation o Loss of vision o Death Demyelination causes the blockage in the conduction (propagation) of AP o “all or none” principle Synaptic Transmission Neuronal Communication Information is transmitted within the neuron by an action potential (electrical signal) Information is communicated between neurons by synapse (point of communication between two neurons) Synapse Synapse is the contact point between two neurons: o Presynaptic neuron → sending neuron o Postsynaptic neuron → receiving neuron The space between both neurons is known as the synaptic cleft (200 – 300 angstrom) Types of Synapses Synapses in the human brain fall into two classes o Electrical synapses (electrical signal) o Chemical Synapse (chemical signal) These two classes of synapse can be distinguished based on their structures and the mechanism they use to transmit signals from the presynaptic to the postsynaptic element Electrical Synapses Electrical synapses permit direct passive flow of electrical current from one neuron to another Electrical current flow through the intracellular continuities called connexons (channel bridges). These connexons are grouped in small areas called gap junctions. Transmission is very fast because the current flow across connexons is virtually instantaneous Transmission is bidirectional allowing electrical synapse to synchronize electrical activity among the population of neurons (pre to post or post to pre) Electrical synapses are minority (in the brain) and are mostly inhibitory synapse Chemical Synapses At the chemical synapse there are no connexons (no intracellular continuity) between the two neurons. (only synaptic cleft = empty space) At the presynaptic terminal, there are synaptic vesicles containing chemical substances known as neurotransmitters Synaptic transmission is initiated when an AP invades the presynaptic terminal and causes the release of the neurotransmitters into the synaptic cleft The binding of neurotransmitters into the postsynaptic receptors causes the activation of the postsynaptic receptors and thus a change in the membrane potential Synaptic Transmission – Neurotransmitter (Ligand) Neurotransmitter is a chemical substance that is synthetized and packaged in the presynaptic terminal and released into the synaptic cleft by the arrival of a nerve impulse (AP). By binding to its specific receptor, the neurotransmitter causes the transfer of the impulse to the postsynaptic neuron. Types of neurotransmitters: Based on the action on the postsynaptic neuron: o Excitatory: Excite (depolarize) postsynaptic membrane (i.e., more likely to generate AP): o Glutamate (Glu), synthesized by Glutamatergic neurons o Dopamine (DA), synthesized by Dopaminergic neurons o Acetylcholine (ACh), synthesized by Cholinergic neurons o Serotonin (5HT), synthesized by Serotonergic neurons o Norepinephrine (NE), synthesized by Norepinephregic neurons o Inhibitory: Inhibit (hyperpolarize) postsynaptic membrane (i.e., less likely to generate AP): o GABA, synthesized by GABAergic neurons o Glycine, synthesized by Glycinergic neurons Each neurotransmitter has his specific postsynaptic receptor (e.g., key and lock). Types of Neurotransmitters Based on size and speed of action of the molecule o Small Molecule; Rapidly Acting Transmitters ▪ Glutamate (Glu) ▪ Acetylcholine (ACh) ▪ GABA, Glycine ▪ Serotonin (5HT) ▪ Dopamine (DA) ▪ Nitric oxide ▪ Norepinephrine (NE) o Large Molecules; Slow Acting Transmitters; Neuropeptides ▪ Hypothalamic-releasing hormones ▪ Pituitary peptides ▪ Peptides that act on gut and brain ▪ Peptides from other tissues Synaptic Transmission – Action of Neurotransmitter on Postsynaptic Neuron Two types of postsynaptic receptors: o Ionotropic receptors o Metabotropic receptors Both receptors contain two components o A binding component which binds the neurotransmitter o An active component: activated by the binding of the neurotransmitters ▪ Ionotropic receptors: active component is an ion channel ▪ Metabotropic receptors: active component is a second messenger activator (G-protein) Synaptic Transmission – Ionotropic Receptors In the ionotropic receptors, the ion channel (active component) is an integrated part of the receptor. Once the neurotransmitter binds into the receptor, the ion channel is activated. Two types of Ion Channels o Cation Channels: allow cations (+ ions) (Na+, Ca2+, K+) to pass. These channels excite (depolarize) the postsynaptic neuron. Make inside the cell positively charged (glutamate receptor) o Anion Channels: allow anions (-ions) (Cl-) to pass. These channels inhibit (hyperpolarize) the postsynaptic neuron. Make inside the cell negatively charged (GABA receptors) Ionotropic receptors open and closes rapidly providing a very rapid control of postsynaptic neurons: fast synaptic transmission. Synaptic Transmission – Metabotropic Receptors In the, the active component is not an integrated part of the receptor. It is a protein structure “second messenger activator” that causes prolonged changes in the neurons (minute to months) by activating substances inside the postsynaptic neuron, slow synaptic transmission. The most common type of second messenger activator uses G – proteins as an active component: o The G-protein is a protein complex (α, β, γ sub-units) attached to the interior portion of the binding component. o The binding of the neurotransmitter on the receptor activates the G – protein, which initiates a cascade of events leading to alternation in the cellular activity. The binding of the neurotransmitter on the receptor activates the G-protein, Upon the activation of the G-protein complex, the α sub-unit detaches from the complex and activates multiple functions inside the cell (e.g., opens ion channels, activates membrane enzymes, activates intracellular enzymes, activates gene transcription). Slow synaptic transmission causes prolonged changes in the neurons (up to months). Electrical Events in the Postsynaptic Membrane The binding of the neurotransmitter on the post-synaptic receptor (ionotropic receptors) opens ions channels and increases the permeability of ions, Causes the postsynaptic membrane potential to shift from the resting state (Vm). The new potential is called postsynaptic potential (PSP). Excitatory postsynaptic potential (EPSP): The membrane potential moves towards less negative values (> Vm, depolarization). Increased permeability to Na+ and/or Ca2+ (more positivity inside). Caused by the activation of excitatory receptors. EPSP favorites the generation of AP (depolarized membrane) Inhibitory postsynaptic potential (IPSP): The membrane potential moves towards more negative values (< Vm, hyperpolarization). Increased permeability to Cl- and/or K+ (more Negativity inside). Caused by the activation of inhibitory receptors. IPSP blocks the generation of AP (hyperpolarized membrane). Characteristics of postsynaptic potentials: Sub-threshold potentials (below threshold of AP) Do not obey to the All-or-none principle (i.e. more stimulus, higher the amplitude). EPSP favorites the generation of AP (signal transmission) IPSP blocks the generation of AP (no signal transmission) Summation of different PSPs (Spatial & Temporal). Summation of Postsynaptic Potentials – Spatial Summation Spatial Summation: when different presynaptic terminals (E1 & E2) stimulate the same postsynaptic neuron, their respective EPSPs summate (i.e., superpose). The membrane potential may reach the threshold to generate an action potential. If the postsynaptic neuron receives an: o Excitatory stimulus (E1) o Inhibitory stimulus (I) ▪ The 2 stimuli cancel each other (i.e. (+1) + (-1) = 0). Summation of Postsynaptic Potentials – Temporal Summation Temporal Summation: when a postsynaptic neuron receives, in a very short period of time, successive stimulations, form the same presynaptic terminal (e.g., E1) o The 2nd EPSP will generate before the recovery of the 1st one, thus both EPSPs can summate. Rapid successive discharges from the same presynaptic terminal can summate to reach the threshold for firing AP Synaptic Plasticity Synaptic plasticity is the ability of a synapse to change (strengthen or weaken) over time, in response to increase or decrease in its activity. Synaptic plasticity results from: o Change in the quantity of neurotransmitters released, o Change in the number of postsynaptic receptors, o Change in the response of the postsynaptic neuron to presynaptic stimulation (EPSP & IPSP). Types of synaptic plasticity: o Synaptic potentiation (enhancement): Increase in the efficacy of the synapse. o Synaptic depression: Decrease in the efficacy of the synapse. o Short-term plasticity: lasts from few milliseconds to minutes. o Long-term plasticity: lasts from minutes to months (even years). Synaptic plasticity is an important neurochemical mechanism of learning and memory (Hebbian theory: “cells that fire together, wire together”). Drugs and Synaptic Transmission Drugs modulate synaptic transmission to affect brain activity: o Medication (e.g., Antidepressants, Antipsychotics…) o Recreational drugs (e.g., Ecstasy, Cocaine, Opiates, Cannabis…) Effect of Ecstasy on Serotonin transmission: o In a healthy brain, serotonin is rapidly reuptake from synaptic cleft by the Reuptake Transporter. o In the presence of Ecstasy, the later blocks the job of the Reuptake Transporter, o Serotonin stays longer in the synapse and over stimulates the postsynaptic neuron. o Ecstasy causes euphoric sensation, increased heart rate, panic attacks, blurred vision, nausea, vomiting, convulsions, and death (overdose). o Very addictive even with low doses. Lecture 3 – The Somatosensory System Somatosensory Axis of the Nervous System Transmission of somatic (body) sensations from the body receptors to the CNS Information is Transmitted to: o Spinal Cord o Brainstem o Cerebellum o Thalamus o Cerebral Cortex Three important elements o Sensory receptors (reception) o Sensory pathways (transmission) o Sensory centers (processing) Classification of Somatic Sensations Mechanoreceptive Sensations → stimulated by mechanical displacements (tissue deformation) o Tactile Sensation (skin) ▪ Touch ▪ Pressure ▪ Vibration ▪ Tickle and itch o Proprioceptive Sensations (muscle and joints) ▪ Muscle condition sense (muscle contracted, stretched, relaxed) ▪ Joints position sense Nociceptive Sensations o Detect pain o Stimulated by any factor that damages the tissue Thermoreceptive Sensations o Detect temperature (heat and cold) o Stimulated by the change in temperature Classification of Somatosensory Receptors Classified based on the type of sensation they detect Mechanoreceptors: detect tissue deformation o Skin Tactile Receptors ▪ Free nerve endings ▪ Expanded tip receptors (Merkel’s Discs) ▪ Encapsulated Endings (Meissner’s Corpuscle, Pacinian Corpuscle, Krause’s Corpuscle, Ruffini’s end organ) ▪ Hair end organ (tactile hair) o Muscle Receptors ▪ Muscle spindles ▪ Golgi tendon organs Nociceptors: detect pain (tissue damage) o Pain receptors: specialized free nerve endings Thermoreceptors: detect change in temperature o Specialized free nerve endings ▪ Clod receptors ▪ Warmth receptors Receptor Potential Resting membrane potential o At resting state (no stimulus) Action potential o Result from transient changed in the resting membrane potential a stimulated neuron o Electrical signal that travels along axons o Long range transmission of information within the nervous system Receptors Potential o Result from transient changed in the resting membrane potential in the receptor of sensory neurons by an external stimulus Synaptic Potential o Results from the communication between neurons at synaptic contacts o Recorded at the postsynaptic neurons by the stimulated of the presynaptic neuron Receptor Potential Receptor Potential o When a stimulus (touch, pain) excites the receptor, the electrical potential of the receptors membrane changes and creates a receptor potential (like EPSP). The stimulation causes opening of ion channels (Na+, Ca2+), depolarization of the membrane. Mechanism of Stimulation of the Receptor o Mechanical deformation, which stretches the membrane (mechanoreceptors) o Application of chemicals (acid, alcohol, drugs) o Change in temperature (thermoreceptors) o Tissue damage (pain receptors) Transduction of Sensory Stimulus The receptor potential rises the membrane potential of the nerve fiber attached to the receptor. If the threshold is reached, an action potential appears in the nerve fiber Action potential travel through the nerve fiber to reach the brain (AP transmits encoded sensory information to the brain) The greater the intensity of the stimulus → The greater the receptor potential amplitude → The greater the rate of action potential Adaptation of Receptors Adaptation of the Receptor o When a continuous sensory stimulus is applied, the receptors respond at a high impulse rate at first stimulus and then progressively slow down their rate of response until many of them no longer respond (adaption of cold water) Speed of adaption varies with the type of receptors o Slowly Adapting Receptors → Decrease their rate of response slowly ▪ Pain receptors ▪ Merkel’s discs ▪ Ruffini’s organ o Rapidly Adapting Receptors → Decrease their rate of response rapidly ▪ Thermoreceptors ▪ Pacinian corpuscle ▪ Hair receptors ▪ Meissner’s corpuscle Examples: Pacinian corpuscle adapts very rapidly Hair receptors adapts rapidly Joints capsule and muscle spindle receptors adapt slowly Adaptation of Receptors Mechanism of Adaptation: 2 Ways o Accommodation of the Receptor ▪ The receptor potential appears at the onset of the stimulus (compression) but disappears rapidly even though the stimulus continues (receptor still under compression, squeezed ball) o Accommodation of the Nerve Fiber ▪ Decrease in the firing of AP that is caused by the inactivation of the Na+ channels (saturation of ions channels) Tactile Receptors and Sensations – 6 Types 1. Free Nerve Endings a. Found everywhere in the skin (mostly on the superficial layers) b. Connected with small unmyelinated sensory nerve fibers c. Detect crude touch and pressure sensations d. Slowly adapting receptors 2. Meissner’s Corpuscle a. Elongated encapsulated nerve endings b. Connected with large unmyelinated sensory nerve fibers c. Located in the superficial layers of the skin (non-hairy part) d. Detect fine touch (discriminative, movement of the objects on the surface of the skin) and low frequency vibrations e. Rapidly adapting receptors 3. Merkel’s Discs a. Located in the superficial layer of the skin (epidermis) b. Detect touch and light pressure c. Slowly adapting receptors 4. Hair end-organ (free nerve ending of hair root) a. In contact with the root of the skin hair b. Detect hair movement c. Rapidly adapting receptors 5. Ruffini’s end-organ a. Encapsulated endings located in the deeper layers of the skin (dermis) b. Detect heavy and prolonged touch and deep pressure signals c. Slowly adapting receptors 6. Pacinian Corpuscles a. Encapsulated endings located in the deeper layers of skin (dermis) b. Detect tissue vibration or other rapid changes in the mechanical state of the tissue c. Rapidly adapting receptors Pain Sensation Occurs whenever tissue is being damaged Protective mechanism for the body (causes the body to remove painful stimulus_ 2 Types of Pain o Fast Pain (pricking pain) ▪ Felt withing less than a second of the stimulus (< second) ▪ Sharp in character ▪ Hitting finger or toes on a hard object o Slow pain (aching pain) ▪ Begins after a second or more (> second) ▪ Throbbing and aching in nature ▪ Headache, toothache Pain Receptors Pain receptors are specialized free nerve endings Widespread in many locations of the body: o Superficial layers of the skin o Internal tissues (tooth, stomach) o Bones, joints, and muscle surfaces o Arterial walls o No pain receptors in the brain Can be stimulated by: o Mechanical (tissue damage, strong stretch) o Chemical (alcohol lactic acid) o Thermal (> 45 degrees) o Inflammation Pain receptors are slow adapting receptors (more stimulus, more response) o Protective mechanism to remove painful stimulus Thermal Sensation They can be graduated Thermal gradations are discriminated by 3 types of sensory receptors (specialized free nerve endings) o Cold receptors: sensible to cold temperatures o Warm receptors: sensible to hot temperatures o Pain receptors: sensible to extreme temperatures Many more cold receptors than hot receptors Freezing cold and burning hot are the same sensation because of the stimulation of pain receptors Thermal receptors are rapidly adapting receptors (swimming in cold water) Transmission of Somatosensory Information from the Receptor to the Brain The somatosensory receptor is the peripheral end process of this sensory neuron (pseudo unipolar) that transmits the sensory information to the central nervous system The cell body of the sensory neuron is in the sensory ganglion (spinal cord) Each type of somatic sensation (sensation modality) is transmitted by its specific sensory nerve (labelled line principle) Velocity (speed) of transmission depends on the fiber diameter and the myelinization of the fiber The larger the nerve fiber diameter, the faster the rate of transmission of the signal and the highest the quality of information (high discrimination) Velocity transmission varies from slow (0.5m/s) to fast (120m/s) Nerve fiber classification: o Type A – myelinated fivers of varying sizes ▪ , , large size - fast transmission speed. ▪ : small size - slow transmission speed. o Type C - unmyelinated fibers: ▪ small size - slow transmission speed. Sensory Transmission Muscle Spindle and Golgi Tendon Organ o Transmit signal through Type - A , myelinated nerve fibers (proprioceptive sensation) o Fast transmission and High discrimination Meissner’s Corpuscle, Hair Receptors, Pacinian Corpuscle, Ruffini organ o Transmit signal through Type - A , , myelinated nerve fibers (tactile sensation) o Fast transmission and High discrimination Free Nerve Ending Receptors o Transmit signal through Type - A delta myelinated nerve fibers (fast pain and pricking or cold) o Transmit signal through Type – B unmyelinated nerve fiber o Slow transmission and low discrimination The more critical the information the faster the rate of transmission Somatosensory Pathways Sensory fibres from the body (except the face) transmit to the brain through the spinal cord Segmental Distribution → each part of the body is connected to a specific portion of the spinal cord Sensory fibers enter the spinal cord through the dorsal root ganglion where their cell body are located Two pathways for sensory information: o Dorsal Column Medial Lemniscal System o Antero – Lateral System Sensory fibers in both pathways decussate (cross the midline to the contralateral side) o Sensory information from one side of the body will be transmitted to the opposite side of the brain Three order neurons make the relay to transmit sensory stimulation from the receptor to the final point in the cerebral cortex Dorsal Column Medial Lemniscal System Transmits tactile sensations (touch, vibration, fine pressure) and proprioceptive sensations (muscle stretch and joint position) Signal originates from tactile receptors (Meissner’s, Ruffini’s Pacinian, Merkel’s) or proprioceptive receptors (muscle spindles and Golgi tendon organs) They use large, myelinated nerve fibres such as type – A , , fibers for fast signal transmission and with high degree of discrimination (fine touch) Three order neurons o 1st order neuron has cell body in the dorsal root ganglion of the spinal cord. It makes synapse with 2nd order neuron at the dorsal column nuclei (Cuneate, Gracial) in the medulla. o 2nd order neuron decussates at the level of Medial lemniscus (medulla), and then forms the medial lemniscus tract (up to the thalamus). o 2nd order neuron makes synapse with the 3rd order neuron at the thalamus. o 3rd order neuron projects into the primary somatosensory cortex. Antero – Lateral System Transmits a broad spectrum of sensory modalities (pain, thermal, crude touch and pressure, tickle and itch, sexual sensation) Signal originates from free nerve ending receptors (pain receptors, thermal receptors, skin free nerve endings) They use smaller myelinated (Type A - ) and unmyelinated type C fibers for slow transmission and low degree of discrimination (vague types of sensory information, aching pain) Three order neurons o 1st order neuron has cell body in the dorsal root of the spinal cord. It makes synapse with 2nd order neuron at the Substantia Gelatinosa in the dorsal horn of spinal cord. o 2nd order neuron decussates at the level of the Anterolateral quadrant in the spinal cord, and then forms the Anterolateral (spinothalamic) tract (up to the thalamus). o 2nd order neuron makes synapse with the 3rd order neuron at the thalamus. o 3rd order neuron projects into the primary somatosensory cortex. Somatosensory Sensory Pathway of the Face Somatosensory information from the face and head is conveyed through the trigeminal nerve The trigeminal nerve enters the CNS through the brainstem Three order neurons o 1st order neuron has cell body in the trigeminal ganglion. It makes synapse with 2nd order neuron at the principal nucleus of trigeminal complex (brainstem). o 2nd order neuron decussates at the level of Medial lemniscus and form Trigeminal lemniscus tract (up to the thalamus) o 2nd order neuron makes synapse with the 3rd order neuron at the Ventral posterior medial nucleus of thalamus. o 3rd order neuron projects into the primary somatosensory cortex. Primary Somatosensory Cortex The primary somatosensory cortex is the terminal station of the somatosensory pathways. It is in the postcentral gyrus (parietal lobe) Highly organized with distinct spatial orientation o Each area of the cortex is devoted to a given body region, map of the sensory representation Each side of the cortex receives information from the opposite side of the body Penfield Homunculus It is an unequal representation of the body o Lips have the greatest representation followed by face, thumb, finger, hand o Trunk and lower body have the least area of representation More a region of the body has receptors the greatest area of representation in the somatosensory cortex o Lips have the most receptors Cellular Organization of the Primary Somatosensory Cortex Somatosensory cortex composed of 6 cellular layers (cytoarchitectonic organization) Layer IV is the input layer (3rd order neuron terminates in layer IV) Within the layers, the neurons are also arranged in vertical columns Each vertical columns is reserved from a specific sensory modality (pain, touch, pressure) Different columns interact between each other to allow the beginning of the processing of sensory signals (giving meaning to sensory information) Somatosensory Association Cortex The somatosensory association cortex is located behind the primary somatosensory cortex in the parietal lobe Association area receives input from the primary somatosensory cortex, ventral basal nuclei of the thalamus, visual and auditory cortex Functions is to decode somatosensory meaning and integrate it with other sensory information Loss of these areas result in various symptoms o Inability to recognize complex objects o Loss of high degree perception on the opposite side of the body (neglect syndrome) Modality of Sensation Sensation modality → each type of sensation: touch. (fine or crude) pressure (light or deep) stretch, vibration, hair movement, pain and temperature is called a sensation modality. The Labeled Line Principle → each sensation modality is transmitted by specific nerve fibers that terminate at a specific in the CNS o When a nerve fiber is stimulated at any level of the pathway and by any type of stimulus, the sensation is felt at the original point (pain receptor at finger) and this is determined or processed by the point in the central nervous system to which the fiber leads (the brain who feels the sensation not the peripheral receptor) Lecture 4 – The Visual System Anatomy of the Human Eye The eye is a fluid filled sphere enclosed by 3 layers of tissues o Sclera → Outermost Layer o Choroid → Intermediate Layer o Retina → Innermost Layer Two fluid compartments o Aqueous Humor ▪ It is in the anterior chamber ▪ Watery liquid ▪ Supplies nutrients to surrounding structures o Vitreous Humor ▪ Thick gelatinous that fills the space of the posterior chamber (between the back of the lens and the retina ▪ It maintains the shape of the eye and supplies nutrients to the surrounding structures Lens → refracts (bends) light rays Parts of the Eye Sclera White fibrous tissue forming the outermost layer of the eye At the front of the eye the Sclera becomes the Cornea The Cornea is a white transparent tissue that separates and protects the eye from external environment and allows light rays into the eye Choroid Intermediate later; ciliary bed that nourishes the retinal cells Ciliary Body A ring of tissue that encircles the lens and consists of a muscular and vascular part o Ciliary Muscle → controls the shape of the lens and is connected to the lens through the Zonule Fibers o Ciliary Processes → produces the aqueous humor in the anterior chamber Iris Colored portion of the eyes seen through the cornea Contains two sets or muscles that contract and adjust the size of the pupil Lens The lends bends light rays coming into the eye and generates a focused image on the surface of the retina Retina The innermost layer of the eye The retina is considered a part of the nervous system. It contains neurons that are sensitive to light and convert visual information to electrical signals and transmit it to brain Photosensitive (photoreceptor) Neurons o Rods o Cones Accommodation of the Lens Accommodation o Dynamic changes in the refractive power of the lens to form a shape image on the retina o These changes are made by the contraction of the Ciliary muscle Distant Vision o Lens becomes thin and flat o Less refractive power Near Vision o Lens becomes thick and round o Most refractive power Emmetropia o In the normal eye, the lens has a right accommodation level, the image is focused right on the retina Myopia o The lens over accommodates (high refractive power), the image is focused on the front of the retina. o Hard to see distant objects o Corrected by concave (diverging) eyeglasses Hyperopia o The lens does not accommodate enough (weak refractive power) the image is focused beyond the retina. o Hard to see nearby objects o Corrected by convex (converging) eyeglasses Rods and Cones Lowest Level of Illumination: only rods are active (high sensitivity to light) At about the Level of Starlight: cones begin to contribute to light perception, they are the only receptors that function under bright light spectrum (indoor lighting, sunlight) Structure of the Retina 1. Photosensitive Cells a. Rods b. Cones 2. Bipolar Cells a. Connect photosensitive cells to ganglion cells 3. Ganglion Cells a. Axons form optic nerve 4. Horizontal Cells a. Regulate the activity of the photosensitive cells 5. Amacrine Cells a. Allow lateral communication between neurons Electrical Activity of Photosensitive (opposite) In the dark → Rods and Cones are depolarized o Na+ and Ca2+ channels are opened, allowing for Na+ and Ca2+ to enter the cell and depolarize it When stimulated by light → Rods and Cones are hyperpolarized o Na+ and Ca2+ channels are closed and K+ channels are opened → hyperpolarization Electrical Activity of Photosensitive of Retinal Neurons* In the Dark (not stimulated by light) o Rods/Cones are depolarized o Rods/Cones inhibit (block) Bipolar Cells (hyperpolarized) o Bipolar cells cannot excite ganglion cells (hyperpolarized) o Visual information is not transmitted to the CNS In Light (stimulated by light) o Rods/Cones are hyperpolarized o Bipolar cells are released from Rods/Cones inhibition (depolarized) o Bipolar cells excite Ganglion cells (depolarized) o Visual information is transmitted to the CNS On – Center / Off – center Ganglion Cells Ganglion cells response differently to light and dark depending on the location of light and dark in the visual field (center or periphery) Two classes of Ganglion Cells o On Center cells → active to light spot in center of the visual field and silent to dark spot in center o Off Center cells → silent to light spot in center of the visual field and active to dark spot in center The activity of on center/off center ganglion cells is controlled by multiple interactions between: o Photosensitive cells o Bipolar cells o Horizontal cells The specific response to light constitutes an efficient manner to transmit visual information to the brain Projection of Images to the Retina On the retinal surface, the image is inverted top to bottom and reversed right to left The imaged is converted to its real position in the visual cortex Visual Field Visual field → part of a word seen by the eye o Divided into two fields: left and right o Each field is divided into: ▪ Median hemi – fields → seen by the Temporal Retina ▪ Lateral hemi – fields → seen by the Nasal Retina Visual fields of both eyes overlap in the central portion = Binocular Visual Field Central Visual Pathways Central Projection of Retinal Ganglion Cells Axons of the ganglion cells form the optic nerve (3rd order neuron) 60% of the optic nerve fibers decussate at the optic chiasm to the opposite side of the brain (contralateral) 40% of the fibers stay on the same side (ipsilateral) of the brain Fibers from the nasal retina project to the contralateral side of the brain (cross at the optic chiasm) Fibers from the temporal retina project to the ipsilateral side of the brain (do not cross optic chiasm) After the optic chiasm, the optic fibers form the optic tract Most of the optic fibers terminate in the Lateral Geniculate Body (LGB) in the thalamus Neurons from the LGB form the optic radiation (4th order neuron) and terminate in the visual cortex (occipital lobe) Axons in the ganglion cells project also to other midbrain areas o Pretectum → control pupillary light reflex o Hypothalamus → regulation of circadian rhythms (biological clock) o Super Colliculus → orienting the movement of the head and eyes Circuitry Responsible for the Pupillary Light Reflex 1. Optic nerves from both eyes project to the pretectum to control the pupillary light reflex 2. Pretectum neurons project into the Edinger – Westphal Nucleus 3. Edinger – Westphal Nucleus neurons send their axons via the oculomotor nerve to terminate on the ciliary ganglion neurons 4. The ciliary ganglion neurons (parasympathetic) innervate the constrictor muscle in the iris and cause the iris muscle to adjust the size of the pupil Visuaoptic Organization of the Visual Cortex Visual cortex = striate cortex = primary visual cortex = V1 = Brodmann’s area 17 The visual cortex processes the basic of sensory information sent by the retina (place back the inverted image to its original position, bine ocular vision, 3D images) Each region of the retina project to a dedicated region of the visual cortex (map of sensory representation) Sensory information from the central region of the retina (macula fovea) has a large representation of the visual cortex This central region of the retina sends the large portion of the sensory information to the visual cortex (bine ocular vision, HD vision) Organization of the Primary Visual Cortex There are 6 cellular layers in the visual cortex “Cytoarchitecture” Layer 4 is the main input layer (receives LGN fibers) Layers 5 and 6 are descending outputs (output of subcortical regions) Layers 2 and 3 are ascending outputs (outputs of other cortical regions) Binocular Vision The lateral geniculate nucleus receives input from both eyes. Geniculate neurons are monocular (receive the image of each eye separately) The LGN fibers project to layer 4 of the striate cortexes. LGN inputs are segregated in layer 4 in ocular dominance columns. Here the neurons of layer 4 are still monocular. Layer 4 neuron send their output to other cortical layers, it is at this stage where the images from both eyes converge to form one single image. o Cyclopean representation of the external world (binocular vision) Selectivity of Neurons in the Primary Visual Cortex Neurons in the primary visual cortex respond selectively to oriented edges Different neuron types respond selectively to different visual information (edge of orientation, color) Visual Areas Beyond the Striate Cortex Primary visual cortex project to other cortical areas “Extrastriate Cortex” (secondary visual cortex) involved in complex pathways perception o Dorsal Pathway (where pathways; action): ▪ Project to the parietal lobe ▪ Responsible of spatial aspect of vision (analysis of motion, positional relationship between object) o Ventral Pathway (what pathway; perception) ▪ Project to the temporal lobe ▪ responsible for object recognition Consequences of Damage at Different points along the Visual Pathway Pituitary Tumors o Pituitary gland sits in cella turcica near optic chiasm o This type of tumor can disrupt the visual pathway o Disrupt information coming from the nasal retina which crosses over at the optic chiasm o The visual information that crosses over at the optic chiasm originated at the temporal visual fields of both eyes o People with this kind of tumor may experience blockage of information coming from the temporal visual fields o This blockage leaves just the central visual field which is a condition called tunnel vision because you just see the center of the visual field Lecture 5 – The Vestibular System Overview The vestibular system is a sensory system located in the inner ear. It processes sensory information underlying: o Motion o Head position o Spatial orientation relative to gravity The vestibular system is responsible for the body’s equilibrium, it helps to stabilize gaze, head, and posture It uses special sensory neurons “hair cells” to transduce physical motion into neural impulses The Vestibular Labyrinth It is composed of two organs o Otolith Organs → Utricle and Saccule o Semicircular Canal → Superior, Posterior and Horizontal Vestibular Hair Cells Flask – shaped epithelial cells with a bundle of hair like processes (stereocilia) attached between each other by a tip link Kinocilium→ The Longest Process Hair cell is a very sensitive to movement. The movement changes its membrane potential: o When the hairs (stereocilia) move toward Kinocilium → depolarization (excitation) o When the hairs (stereocilia) move far from the Kinocilium → hyperpolarization (excitation) When the stereocilia is deflected toward the kinocilium, tip links are stretched and directly open K+ - selective channels near the tips of the stereocilia, allowing K+ to flow into the hair cell and depolarize the hair cell The resulting depolarization of the hair cell opens Ca2+ channels in the cell body, allowing Ca2+ entry and release of neurotransmitter to excite the post – synaptic neuron (vestibular nerve) and the electrical signal to the brain The hair cell (receptor) NOT directly attached to the nerve Otolith Organs Utricle and Saccule Each organ contains o Macula → sensory epithelia containing the soma (cell body) of hair cells and supporting cells o Gelatinous Layer → containing hair bundles o Otolithic Membrane → fibrous layer emending “otoconia”: crystals of calcium carbonate Movement Detection o They detect linear movement ▪ Translation movements of the head (forward and backward) ▪ Static head movement relative to the gravitational axis (head tilt) The otoconia (ear rocks) make the otolithic membrane heavier than the structures and the fluid surrounding it. o When the head tilts or moves, gravity causes the otolithic membrane to shift relative to the macula. The hairs in the gelatinous layer displaced and a receptor potential is generated. Utricle Positioned Horizontally o The Utricle detects the Horizontal Translational Movements of the head (moving forward or backwards = moving within the X – axis) ▪ Walking, being in a moving car Saccule Positioned Vertically o The Saccule detect the Vertical Translational Movements of the head (moving up and down = morning within the Y – axis) ▪ Being in an elevator Semicircular Canals Fluid filled tubes specialized for responding to rotations of the head 3 Semicircular Canals o Horizontal → turning left & right (no sign) o Superior → nodding up and down (yes sign) o Posterior → tilting to a side (towards the shoulder) Each semicircular canal works in opposite manner with its pair located on the opposite side of the head. o A tilt of the head to one side (right) activates the hair cells in the right horizontal canal but inhibit the hair cells in the left horizontal canal. Ampulla → An enlargement of the base of each semicircular canal. It continues the hair cells The cell bodies: o Hair cells are housed in the Crista (epithelial layer) o Hair bundles extend out into the Capula (gelatinous mass) Endolymph Fluid → circulated in the semicircular canals The rotation of the head causes the fluid to move the endolymph fluid in the opposite direction of the head movement, which pushes the Capula and displaces the hair bundles. As a result, the hair cell is depolarized and receptor potential is generated, the electrical signal is sent to the brain. Central Pathways of the Vestibular System The vestibular nerve (VIII cranial nerve = vestibulocochlear nerve) projects its fibers to the vestibular nucleus located in the brainstem. Neurons in the vestibular nucleus send projections to several brain regions (thalamus, cerebral cortex, abducens, nucleus, oculomotor nucleus, cerebellum, spinal cord) Neurons in the vestibular nucleus receive multisensory inputs (visual, somatosensory, motor, cerebellar) The vestibular system contributes to many gelatinous masses, such as o Reflexive eye movements that stabilize gaze (vestibulo-ocular reflex) o Rapid postural adjustments reflexes to maintain balance, o Higher-order processes that are important to our sense of spatial orientation and self-motion. Vestibular Pathway to the Cortex 1. The vestibular nerve projects its fibers to the vestibular nuclei (superior and lateral) located in the brainstem (1st order neuron) 2. The vestibular nuclei send axons to the ventral posterior nucleus complex of the thalamus (2 nd order neuron) 3. Thalamic neurons (3rd order neuron) project to a variety of cortical areas that respond to vestibular stimuli (vestibular cortex) as well as proprioceptive (3A, 2V) and visual stimuli (visual posterior sylvian area) o Reflecting the multisensory nature of central vestibular processing Many of these cortical neurons are activated by rotation of the body (even when the eyes are closed) as well as by moving visual stimuli suggesting that these cortical regions are involved in the perception of body orientation in extra personal space (the space that surrounds us) The Vestibulo – Ocular Reflex (VOR) (automatic) The vestibulo – ocular reflex produces eye movements that counter head movements (moving the eyes in opposing direction of the head) thus permitting the gaze to remain fixed on a particular point o When the head turns to the left, the VOR reflexes causes the eye movements to the right to stabilize the gaze Example When the head turns to a side, (e.g., left) 1. The left horizontal semicircular canal nerve sends signal to the vestibular nucleus. 2. The vestibular nucleus, in turn, sends 2 projections: a. 1 excitatory to the right abducens nucleus (red line) b. 1 inhibitory to the left abducens nucleus (black line), 3. The right abducens nucleus sends: a. an excitatory projection to the right lateral rectus muscle and force it to contact b. sends an excitatory projection to the left medial rectus muscle via the oculomotor nucleus, to force it to contact, 4. The left abducens nucleus is inhibited. a. cannot excite the lateral rectus muscle of left eye (relaxed), nor the medial rectus muscle to the right eye (relaxed), 5. The right lateral rectus and left medial rectus are contracted, and at the same time the left lateral rectus and right medial rectus are relaxed, 6. Both eyes move to the right. Turning the head to one side (e.g., left) activates the horizontal canal of the same side (e.g., left) that causes the eyes to move to the opposite side (i.e., right). The Vestibulo – Cervical Reflex (VCR) (automatic) VCR regulates head position by reflex activity of neck muscles in response to stimulation of the semicircular canals following a head movement. Example During a downward pitch of the body (e.g., tripping), the superior canals are activated, and the neck muscles reflexively pull the head up (dorsal flexion of the head) to maintain stability of the head. In VCR: The superior canal sends projections to the medial vestibular nucleus. Axons from the medial vestibular nucleus descend in the Medial vestibulospinal tract to terminate at the upper cervical levels of the spinal cord. In the spinal cord, they stimulate the cervical lower motor neurons to activate the neck/head extensor muscles to cause the dorsal flexion of the head The Vestibulo – Spinal Reflex (VSR) (automatic) The vestibulo-spinal reflex (VSR) causes → the extension of the forelimb and the flexion of the hindlimb, to stabilize the body and protect it against falling. In VRS, the information is sent from the otolith organs and project mainly to the lateral vestibular nucleus, Axons from the lateral vestibular nucleus descend in the lateral vestibulospinal tract to terminate at the thoracic levels of the spinal cord, The fibers of the lateral vestibulospinal tract descend in the lateral vestibulospinal tract to terminate at the thoracic levels of the spinal cord to excite the lower motor neurons that contract the extensor muscles (antigravity) in the trunk and limbs, By activating the pool of motor neurons innervating extensor muscles in the trunk and limbs, this pathway mediates balance and the maintenance of upright posture. Vestibular Cerebellar Pathways The vestibular system and cerebellum work together to coordinate body balance and equilibrium. The vestibular system and cerebellum have an extensive reciprocal connection: o The cerebellum is a major target of the vestibular pathways, and in turn it provides descending input to the vestibular nuclei. o Neurons in the vestibular nuclei receive input from the cerebellum, allowing the cerebellum to influence posture and equilibrium (e.g., adjusting VCR & VSR reflex). The vestibular-cerebellar pathways play a critical role in integrating and modulating vestibular signals to: o Enable adaptive changes to the VCR & VSR, o Distinguish head tilts from translational movements, o Distinguish passive movements of the head and body from those that are self-generated. Real – Life Situation Implicating the Vestibular System Blindfolded subject on a rotating chair: what happens to a blindfolded subject riding on a chair rotating at a constant speed? First, he will perceive that the rotation is slowing down, and after about 30 seconds, he feels that the rotation has stopped entirely, although the chair is still constantly rotating. o Explanation: during constant velocity, firing rates of vestibular fibers that innervate the semicircular canals return to baseline levels as the cupula returns to its undeflected state (even the head is still rotating). o Motion sickness (car sick): caused by repeated movements when travelling (e.g., acceleration, deceleration, going over bumps…). It causes dizziness, nausea, and vomiting. o Explanation: the brain receives conflicting information from the vestibular system, eyes, and somatosensory system (muscles). The vestibular system reports rocking back and forth while the car seems perfectly stable based on the visual and somatosensory information. Oscillopsia A condition in which a person with vestibular damage finds it difficult or impossible to fixate on visual targets while the head is moving (e.g., reading street signs, checking a car’s blind spot, shooting a target while moving, …). The underlying problem in such cases is that information about head movements normally generated by the vestibular system is not available to the oculomotor centers (VOR), so that compensatory eye movements cannot be made. The patient has illusion that the environment is moving when he moves his head. Lecture 6 – The Motor System – Lower Motor Neuron Motor Axis of the Nervous System Motor System o Responds adequately to the order of integrative and sensory divisions Controls the body motor activities o Skeletal (striated) muscles o Smooth muscles (internal organs, heart, stomach) Motor (pyramidal) pathways is composed of 2 order neurons o 1st order neuron → Upper Motor Neuron ▪ Starts at the motor cortex → Ends at the anterior (ventral) horn of the spinal cord ▪ Stimulates and modulates the activity of the lower motor neuron ▪ Longest fibers of the CNS o 2 order neuron → Lower Motor Neuron nd ▪ Starts at the anterior horn of the spinal cord → Ends at the neuromuscular junction ▪ Exists muscle ▪ Integrated in the circuit of motor reflexes Organization of the Central Nervous System Spinal Cord Level o Is the intersection that conducts sensory and motor signals from PNS → CNS and CNS → PNS The spinal cord is the centre of processing basic motor and sensory information: o Reflexes can work without the need of other levels o Circuit for motor reflexes: ▪ Stretch Reflex ▪ Golgi Tendon Reflex ▪ Withdrawal Reflex o Circuits for reflexes that control internal organ function ▪ Blood vessels, gastrointestinal, urination Neuronal Organization of the Spinal Cord Sensory Neurons (dorsal root ganglion) o Transmit somatic sensations (tactile, proprioceptive, pain) to the CNS o Synapse locally in the spinal cord to be integrated in motor reflexes o Transmit signals to higher centers (somatosensory pathways) Motor Neurons (ventral (anterior) horn of spinal cord) o Directly innervates skeletal muscle fibers (lower motor neurons) ▪ Alpha motor neurons (A – alpha axons) excite extrafusal muscle fibers (motor unit) for muscle contraction ▪ Gamma motor neurons (A – gamma axons) excite intrafusal muscle fibers (muscle sensory receptor) to adjust sensory input o In contact with the upper motor neuron (descending fibers) (receives the order to stimulates muscles) Interneurons (dorsal horn of spinal cord) o Local neurons that have interconnections with sensory and motor neurons o Synapse with motor neurons at the ventral horn of the spinal cord o Inhibitory and excitatory interneurons o Integrated in motor reflex Sensory Receptors of the Muscle Proprioceptive Sensation o Senses the state of the muscle (relaxed, contracted, stretched) and the tension practiced on the muscle Mechanoreceptors → sensory receptors of the muscles o They are stimulated by mechanical displacement of the muscle o Slowly adapting receptors Muscle spindles o Are distributed throughout the muscle belly (intrafusal fibers) o Sense muscle length (stretched, relaxed, contracted) and rate of change of length Golgi Tendon Organs o Are found in the muscle tendon o Sense the tension of the tendon and rate of change of tension Signals from both Muscle Spindle and Golgi Tendon Organs o Transmitted to higher centers (cerebral cortex, cerebellum) through: ▪ Dorsal Column Medial Pathway o Integrated in motor reflexes (spinal cord) Muscle Spindles Muscle spindle is a stretch receptor located at the intrafusal muscle fibers (no myosin and actin) It signals the length of muscles and changes in length of muscle Stretching the muscle → excites the receptors and increases the firing of AP in the fiber Contraction of the muscle → inhibits the receptors and decreases the firing of AP in the fiber Signals are transmitted through two fiber types: o Primary sensory ending (Ia) fibers → transmit information about the rate of change of the muscle length, (fast or slow) o Secondary sensory ending (II) fibers → transmit information about the degree of stretch of the muscle (too stretched, too contracted) Golgi Tendon Organ Encapsulated sensory receptor through which a bundle of muscle tendon fibers pass Stimulated when this bundle of muscle is “tensed” by the contraction of the stretching of the muscle (sensitive to muscle tension) Signals are transmitted through o Ib fibers → these fibers inhibit indirectly (through interneurons) alpha motor neuron at the spinal cord to allow the muscle to relax and thus to relieve the tension on the tendon (Golgi Tendon Reflex) Lower Motor Neuron Two types of lower motor neurons → their cell bodies are located in the ventral horn of the spinal cord o Large alpha MN’s → innervate extrafusal fibers of skeletal muscle that generate forces needed for posture and movement (contraction) o Small gamma MN’s → innervate intrafusal muscle fibers (muscle spindles) to modulate (adjust) sensory input Motor Unit Muscle fibers are innervated by nerve fibers (motor neurons) to cause muscle contraction One nerve fiber (motor neuron) innervated multiple muscle fibers The motor unit is the smallest subunit of muscle fibers that can be controlled by a single motor neuron Small motor unit → small muscles that require precision (hand, eye, laryngeal) have low innervation ratio (one nerve innervates 2 – 3 muscle fibers) Large motor unit → large muscles that do not require precision (soleus, quadricep) have high innervation ratio (one nerve fiber innervates 80 – 100 muscle fibers) The synapse between the motor neuron and muscle fiber is called: o Neuromuscular Junction → releases acetylcholine (type of synapse) Excitation Contraction Coupling Force Gradation – Summation A muscle twitch (contraction) is the tension developed in response to one motor neuron stimulation Summation means the adding together of individual muscle twitches to increase the intensity of overall muscle contraction Summation occurs in two ways: o Multiple Fiber Summation (size principle) ▪ Increasing the number of motor units contracting at the same time (recruitment) o Frequency Summation ▪ Increasing the frequency of stimulation of one motor unit Multiple Fiber Summation (size principle) Size principle: depending on the intensity of the stimulation, motor units are recruited in an orderly fashion according to their size o Smallest motor units are recruited first (for weak stimulation) o Largest motor units are recruited last (for strong stimulation) o It allows the graduations of muscle force from small steps (weak contraction) to great steps (strong contraction) Frequency Summation and Tetanization As the frequency of the signal increases individual twitches summate (there is no recovery from the previous contractions) When the frequency reaches a critical level, the submitted twitches fused together to form 1 continuous contraction Tetanization (tonic state) o Contraction reaches max level, no response to further stimulations o Ca2+ ions are maintained in the sarcoplasm; the contractile state of the muscle is sustained (actin and myosin stay attached) o There is a maximum summation when stimulations are very close together Spinal Cord Reflexes Spinal cord reflexes → it is a mechanism by which a sensory impulse is automatically converted into a motor response. Involuntary (no need for upper brain levels) and very rapid mechanism that is controlled by the spinal cord integration centre Protective mechanism to the body (can prevent an over stretch or contraction of the muscle, withdrawal from the pain stimulus, keep balance) Spinal Cord Reflexes o Stretch Reflex ▪ Patellar reflex (knee jerk reflex) ▪ Biceps reflex o Golgi Tendon Reflex o Withdrawal Reflexes ▪ Withdrawal reflex ▪ Crossed extensor reflex Stretch Reflex Mechanism of a stretch reflex o Stretching the muscle activates the muscle spindle o Associated with sensory fibers (Ia and II) that transmit impulses to the spinal cord 1. Sensory fibers synapse directly (monosynaptic) with a motor neuron to cause the contraction of the stretched muscle (extensor) 2. Sensory fibers synapse with an interneuron that inhibit the motor neuron of antagonist muscle (flexors) to prevent it from contracting (relax) Stretch Reflex – Neuronal Circuits – Patellar Reflex Stretch (patellar) reflex causes a sudden forward kicking movement of the lower leg in response to a sharp tap on the patellar ligament. Function of the stretch reflex → is to oppose sudden changes in muscle length (stretching). o Over-stretching = tear. Stretching the muscle activates sensory fibers of muscle spindles: o Sensory fibers excite monosynaptically (directly) alpha motor neurons of the stretched muscle (Quadriceps) and cause it to contract (extend of the lower leg). o Sensory fibers excite interneurons that in turn inhibit alpha motor neurons of the antagonist muscle (Hamstrings) to prevent it to contract (relax). ▪ Polysynaptic (indirect) inhibition. ▪ 2 synapses (excite interneuron; inhibit alpha motor neuron) Abnormality of the reaction suggest that there may be damage to the CNS or PNS. Stretch Reflex – Neuronal Circuits – Biceps Reflex Biceps reflex causes a sudden kicking movement of the lower arm in response to a sharp tap on the biceps tendon. This reflex opposes further stretch of the muscle. Stretching the muscle activates the muscle spindles: o Sensory fibers excite monosynaptically (directly) alpha motor neurons of the stretched muscles (Biceps & Brachialis) and cause them to contract (flexion of the lower arm). o Sensory fibers excite interneurons that in turn inhibit alpha motor neurons of the antagonist muscle (Triceps) to prevent it to contract (i.e. relax). ▪ Polysynaptic (indirect) inhibition. Abnormality of the reaction suggest that there may be damage to the CNS or PNS. Golgi Tendon Reflex Opposite of the stretch reflex (inverse stretch reflex). Sudden backward movement of the leg. Function → relieves excess tension on the tendon (prevent it from tearing). Contracting of a muscle (Quadriceps) creates a tension on the tendon attached to the same muscle and thus activates the Golgi tendon organs. Golgi tendon sensory fibers (Ib) transmit impulses to spinal cord: o Sensory neurons synapse with inhibitory interneurons that inhibit alpha motor neurons of the contracted muscle (Quadriceps) and cause it to relax. o Sensory neurons synapse with excitatory interneurons that excite alpha motor neurons of antagonist muscles (Hamstrings) and cause it to contract. Withdrawal Reflex (Flexor Reflex) The withdrawal reflex is initiated by a painful stimulus that causes automatic withdrawal of the threatened body part. Pain receptors will detect and initiate cascade of events Pain receptors goes through the anterolateral pathway Pain sensory neurons synapse with a pool of spinal interneurons: o Excite motor neurons of the flexor muscle (Biceps) and cause the muscle to contract. o Inhibit motor neurons of the extensor muscle (Triceps) and cause the muscle to relax. Withdrawal and Crossed Extensor Reflex – Neuronal Circuits Two reflexes: Withdrawal & Crossed Extensor reflexes: Painful stimulus elicits: o Flexor reflex (withdrawal reflex) in affected limb (limb withdrawal): ▪ Contraction of the flexor muscle and relaxation of the extensor muscle. o Extensor reflex (crossed extensor reflex) in the opposite limb (limb extension): o Contraction of the extensor muscle and relaxation of the flexor muscle. This series of reflexes serves to push body away from the stimulus, but also to shift weight to the opposite limb (prevent falls). Stretch Reflex – Clinical Application Abnormality in the stretch reflexes response suggests that there may be damage to the lower motor neuron or upper motor neuron. Hyporeflexia: weak (or absent) reflex response: o The problem is in the lower motor neuron. o Disruption of either afferent or efferent fibers (PNS) in the stretch reflex loop (cut in the fibers, neuropathies, nerve, or root compression) o Localisation of a spinal segment: ▪ Patellar reflex corresponds to L4 ▪ Biceps reflex corresponds to C5-C6 Hyperreflexia: strong reflex response: o The problem is in the upper motor neuron. o The upper motor neuron has an inhibitory control on the stretch reflex. o Loss of central command on the spinal cord leads to an enhanced stretch reflex (CNS lesions affecting the motor pathways). Lecture 7 – The Motor System – Upper Motor Neuron Motor Cortex The motor cortex is in the frontal lobe and divided into 3 sub areas: o Primary Motor Cortex o Premotor Area – part of Motor Association Cortex o Supplemental Motor Area – part of Motor Association Cortex Primary Motor Cortex Located in the precentral gyrus (frontal lobe) Map of motor representation of body muscles Unequal topographic representation, Penfield Homunculus: o Small muscles with low innervation have the greatest representation Stimulation of specific motor area (legs) leads to contraction of the associated region of the body (legs) Map of the Body Representation in the Sensory and Motor Cortex Organization of the Primary Motor Cortex 6 Horizontal Layers Layer 5 o House of the upper motor neuron (giant pyramidal neurons = Betz cells) o Descending output layer (gives rise to descending pyramidal tracts) Layer 2 – 4 o Input layers from other cortical areas (motor association cortex, somatosensory cortex) Layer 6 o Cortical output layer; gives rise to cortico-cortical fibers Vertical Columns o < 1mm in diameter o High density of neurons in each column o Function unit: each column stimulates a single muscle of a group of synergistic muscles (motor units) Motor Association Cortex – Premotor Area Premotor Area o Topographical organization like the primary motor cortex o Integrative processing system ▪ Uses information from input (sensory, visual) to determine output (motor) response o Function – planning of movement ▪ Activation of the premotor area results in more complex pattern movement (position shoulder and arms so hands are properly oriented for a specific movement) o Works in concert with other motor areas (primary motor, basal ganglia, thalamus, cerebellum) Motor Association Cortex – Supplemental Motor Area Supplemental Motor Area o Specific topographical organization o Often elicits bilateral movement (grasping of both hands) o Functions in concert with premotor area for control of posture and position (positional movement of the head and eyes) Motor Cortex – Specialized Area’s 1. Broca’s Area a. Dedicated to motor speed production (word formulation) b. Located in the dominant hemisphere (left hemisphere for right-handed people) c. Damage causes language production deficit (unable to form words) i. Broca’s Aphasia 2. Hand Skills Area a. For performing coordinated and purposeful hand movements b. Damage can cause the inability to perform coordinated hand movement i. Motor Apraxia 3. Eye Movement and Head Rotation Areas a. For coordinated voluntary head and eye movement towards specific objects b. Modulate the vestibulo – ocular reflex Pyramidal/Cortico – Spinal Pathway Upper Motor Neuron (1st order neuron): o Fibers originate mostly from the primary motor cortex (but also premotor or SMA areas) o Majority of the fibers (75-95%) decussate at the medulla (pyramids): ▪ Form the lateral corticospinal tract ▪ Synapse with the lower motor neuron at the lateral ventral horn of the spinal cord ▪ Stimulate distal (limb) muscles (arms and legs) o Minority of the fibers (10-25%) do not decussate in the medulla ▪ Form the anterior (ventral) corticospinal tract ▪ Decussate in the spinal cord ▪ Synapse with the lower motor neuron at the medial ventral horn of the spinal cord ▪ Stimulate proximal (axial) muscles (trunk) Pyramidal/Cortico – Spinal Pathway Lower motor neuron fibers leave the spinal cord through the ventral root At the periphery, motor nerves meet with sensory nerves to form mixed nerves Segmental distribution (each segment of the spinal cord is connected to a specific part of the body) Cortico – Bulbar Pathway This pathway stimulates facial muscles 2 order neurons o 1st order neuron (upper motor neuron) originates from the primary motor cortex and premotor areas (cingulate gyrus) o It synapses bilaterally with the 2nd order neuron (lower motor neuron) at different levels of the brainstem o 2nd order neuron (lower motor neuron) leaves the brainstem through many cranial nerves: ▪ Trigeminal nerve (V): stimulates skeletal muscle of mastication ▪ Facial nerve (VII): stimulates skeletal muscle of facial expression ▪ Hypoglossal nerve (XII): stimulates skeletal muscle of tongue Influence of Upper Motor Neurons on Muscle Activity The activity of upper motor neurons in the cortex controls movement, rather than individual muscle contraction (coordinated function) o The upper motor neuron has an inhibitory control on the motor reflexes o Stimulation of the motor cortex (upper motor neuron) initiates the excitation of several muscles and simultaneously suppression of others o A particular movement (hand movement) can be elicited by stimulation of widely separated cortical sites o Regions responsible for initiating different movement overlaps substantially o The force generated by contracting muscles (magnitude) depends on the firing rate of the upper motor neurons o The direction of the force produced by muscles is also dependent on the activity of upper motor neurons Extrapyramidal Pathway = Accessory (indirect pathway) Extrapyramidal pathway o Signals are related through multiple accessory pathways involving the basal ganglia, cerebellum, brainstem nuclei. Most of these pathways modulate movement through the pyramidal pathway (UMN & LMN) Basal Ganglia Pathways (complex motor actions) Cerebellum Pathways (movement coordination) Vestibulospinal Spinal Pathway (antigravity and equilibrium, see vestibular system VSR) Corticorubrospinal Pathway (fine motor control) Cortico – Rubro – Spinal Pathway This pathway serves as an alternative pathway to transmit cortical motor signals to the spinal cord (accessory root) Motor Cortex → Red Nucleus → Spinal Cord o Stimulation of the red nucleus causes motor movement in specific groups of muscles but not as precise as the primary motor cortex o Input from the primary motor cortex to the red nucleus (midbrain) goes through the Corticorubral Tracts ▪ Once arrived at the red nucleus primary motor cortex fibers synapse in the lower portion of the red nucleus called the magnocellular cortex o Magnocellular portion fibers create the Rubrospinal Tract ▪ The fibers from the magnocellular cortex decussate in lower medulla ▪ Descends adjacent and anterior to the pyramidal tract ▪ Terminates mostly on interneuron but also on motor neuron (LMN) Pathophysiology of the Motor System Pathophysiology of the Motor System – Babinski Sign (plantar reflex) Pathophysiology of the Motor System – Patterns of Facial Weakness The distribution of facial weakness provides important localising clues indicating whether the underlying injury involves the upper motor neuron or the lower motor neuron Injury to the primary motor cortex (lesion A) causes a weakness of the contralateral inferior facial muscles o Input to the inferior facial muscles from the UMN in the primary motor cortex is lost o Input to the superior facial muscles from the UMN in the pre-motor areas (cingulate gyrus) remains intact because the input projects bilaterally Injury to the corticobulbar tract (lesion B) causes a weakness of the contralateral inferior facial muscles o Input to the inferior facial muscles from the UMN in the primary motor cortex is lost o Input to the superior facial muscles from the UMN in the pre-motor areas (cingulate gyrus) remains intact because the input projects bilaterally Injury to the facial motor nucleus or its nerve (lesion C) causes a weakness of all muscle of facial expression on the same side of the lesion o Input to the superior and inferior facial muscles from the LMN is lost Pathophysiology of the Motor System – Aphasia Aphasia is a disorder in the comprehension and expression of speech o Broca’s (motor) Aphasia → loss of the ability to produce speech o Wernicke’s (non-motor) Aphasia (fluent aphasia) → not coherent speech Broca’s Aphasia Characterized by the loss of the ability to produce speech (inability to form words). Lesion in the Broca’s area → the posterior inferior frontal gyrus (inferior part of the motor cortex). For that is also called motor aphasia. Mostly caused by a stroke. Associated with hemiplegia (paralysis of contralateral side of the body). It varies from the complete loss of the ability to speak to struggle to speak (non-fluent speech). Possibility of recovery by speech therapy. Pathophysiology of the Motor System – Myasthenia Gravis Myasthenia gravis → a neuromuscular disease that causes weakness in the muscles (e.g. drooping of eyelids, difficulty swallowing, shortness of breath). o Pathology at the neuromuscular junction o Acetylcholine (Ach) receptors on the muscle cell membrane are blocked (or destroyed) by antibodies (autoimmune disease) o Reduced transmission of Ach in the muscle o Impaired muscle contraction o Treatment → Cholinesterase inhibitors → inhibit the metabolism of Ach by the cholinesterase enzyme (i.e., keep more Ach in the synaptic cleft), Thus, boost signals between nerves and muscles to improve muscle strength. Lecture 8 – Modulation of Movement by the Basal Ganglia Integrative Motor Control Overall Scheme for integration of motor function Integration of motor function = coordinated movements Basal Ganglia Basal Ganglia → refers to a large functionally diverse set of nuclei that lies deep within the cerebral hemispheres Consists of 5 Nuclei: o Caudate Nucleus – Striatum o Putamen – Striatum o Globus Pallidus ▪ External ▪ Internal o Substantia Nigra ▪ Pars Compacta ▪ Pars Reticula o Subthalamus / Subthalamic Nucleus Motor Function of the Basal Ganglia Influences movement by regulating the activity of the upper motor neuronal circuits o Regulate muscle contraction o Force initiation and termination of movement o Regulate multi-joint movements o Control movement sequencing o Oculomotor control (saccadic eye movement) o Motor habit learning Control of complex patterns of motor activity o Writing o Using scissors o Coordinating head and eye movements o Some aspects of vocalization (speech) Basal Ganglia Input Zone Input zone → striatum o Caudate o Putamen Receives and processes movement-related signals from o Cerebral cortex (frontal, parietal, and temporal cortex) o Substantia nigra pars compacta (dopaminergic neurons) Internal Circuits Medium spiny neurons → main neurons of the striatum o Inhibitory → release GABA Axons of the medium spiny neurons project to o Globus pallidus (external and internal) o Substantia nigra pars reticulata (no pars compacta) Output Zone Axons from globus pallidus 1. Neurons of the internal segment of globus pallidus (inhibitory) 2. Ventral anterior and ventral lateral complex nuclei of the thalamus (excitatory) 3. Motor complex of the frontal cortex (upper motor neurons) Axons from substantia nigra pars reticulata 1. Neurons of the substantia nigra pars reticulata (inhibitory) 2. Superior colliculus (excitatory) that command head and eye movements Efferent cells of the output zone (Globus pallidus and Substantia nigra pars reticulata) are inhibitory o Higher levels of spontaneous activity that prevent unwanted movement by tonically inhibiting cells in the thalamus and superior colliculus Chain of Nerve Cells Arranged in Disinhibitory Circuit At resting state o Striatum neuron is silent → NO inhibition of globus pallidus o Globus pallidus neuron is tonically inhibiting VA/VL thalamus neuron o NO excitation of the motor neuron At initiation of voluntary movement o Striatum neuron is excited by cortical inputs o Globus pallidus neuron is inhibited by striatum neuron o VA/VL thalamus neuron is disinhibited (released from inhibition) by excitatory sensory inputs o Upper motor neuron is excited by VA/VL thalamus neuron o Lower motor neuron is excited by upper motor neuron Globus pallidus (B) doing opposite of everyone (tonically active at rest) B inhibited → direct pathway so everything is excited/disinhibited Basal Ganglia Pathways 2 pathways that influence differently the activity of the upper motor neuron o Direct pathway ▪ Facilitate the initiation of voluntary movement ▪ Releases the thalamic neurons from tonic inhibition practiced by the globus pallidus inputs ▪ Excite upper motor neurons to initiate voluntary movements o Indirect pathway ▪ Prevent unwanted movement ▪ Increases the level of tonic inhibition on the thalamic neurons practiced by the globus pallidus inputs ▪ Blocks upper motor neurons to prevent unwanted movements Direct Pathway 1. Cerebral cortex sends excitatory (transient) signal to the caudate/putamen 2. Caudate/putamen receive also an excitatory (dopaminergic) signal from the substantia nigra pars compacta (through D1 receptors) 3. Caudate/putamen sends an inhibitory (transient) signal to globus pallidus internal segment, 4. Globus pallidus internal segment does NOT send a tonic inhibitory signal to the VA/VL complex of the thalamus 5. VA/VL complex of the thalamus is released from tonic inhibition a. Sends an excitatory (transient) signal to the motor cortex (upper motor neurons), 6. Movement is allowed to occur Indirect Pathway 1. Cerebral cortex sends an excitatory (transient) signal to the caudate/putamen 2. Caudate/putamen receive also inhibitory signals from the substantia nigra pars compacta (through D2 receptors) 3. Caudate/putamen sends an inhibitory (transient) signal to the globus pallidus external segment 4. Globus pallidus external segment send inhibitory signals to the subthalamic nucleus, which also receives a strong excitatory input from the cortex (don’t cancel out, slows down the excitation) 5. Subthalamic nucleus in turn send excitatory inputs to the globus pallidus internal segment 6. Globus pallidus internal neurons inhibit tonically the VA/VL complex of the thalamus 7. VA/VL complex of the thalamus DOES NOT send excitatory inputs to the motor cortex a. Movement is not allowed to occur Direct pathway, when activated increases excitatory influences on UMNs Indirect pathway, when activated increases inhibitory influences on UMNs Functional Organization of the Direct and Indirect Pathways Cortical input activates direct and indirect pathways o Direct pathway ▪ Disinhibits the VA/VL complex of the thalamus to allow the activation of intended motor programs in targeted muscles o Indirect pathway ▪ Increases inhibitory influences on other upper motor neurons to suppress competing motor programs → block unwanted movement in other muscles that do not contribute to the wanted movement Direct pathway can be seen as the accelerator and the indirect pathway as the brake Dysfunction can explain Parkinson’s disease and Huntingdon’s disease Principle Functions of Basal Ganglia in Motor Control Direct pathway → facilitates the initiation of motor programs that express movement o Promote voluntary movement in targeted muscles Indirect pathway → facilitate the suppression of competing or non-synergic motor programs o Inhibit movement in other muscles that do not contribute to overall wanted movement Simultaneous activity of the 2 pathways leads t