Exam 1 - Metabolism & Excretion PDF
Document Details
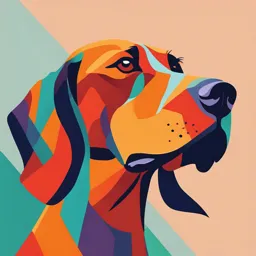
Uploaded by EngagingArtDeco
University of Missouri
Alex Bukoski
Tags
Related
- Pharmacokinetics Lectures PDF
- Pharmacokinetics II: Metabolism and Excretion PDF
- Pharmacokinetics: Drug Absorption, Distribution, Metabolism, and Elimination PDF
- Pharmacokinetics: Metabolism And Excretion Of Drugs, Kinetics Of Elimination PDF
- Introduction to Pharmacokinetics PDF
- Pharmacokinetics: Absorption, Distribution, Metabolism, and Excretion (ADME) PDF
Summary
This document describes metabolism and excretion (elimination) in animals, focusing on pharmacokinetics. It covers topics such as the rate of elimination, clearance, and the interaction of clearance and volume of distribution. The examples and figures illustrate different drug interactions. Provides a great overview of the topic, which can be used as a study guide for the topic or when you need a review.
Full Transcript
Metabolism and Excretion (Elimination) Alex Bukoski PhD DVM DACVAA VBMS 5507 - Instructional Period 7 University of Missouri College of Veterinary Medicine Veterinary Health Center A369 Clydesdale Hall [email protected] 1 Outline Phases of the...
Metabolism and Excretion (Elimination) Alex Bukoski PhD DVM DACVAA VBMS 5507 - Instructional Period 7 University of Missouri College of Veterinary Medicine Veterinary Health Center A369 Clydesdale Hall [email protected] 1 Outline Phases of the c(t) curve Rate of elimination 1. Terminal elimination half-life (t1/2 ) Body’s ability to eliminate drug 1. Clearance 2. Total body clearance (Cl) 3. Clearance models 4. Interaction of Cl and VD 5. Metabolism: Phase 1 and phase 2 reactions 6. Hepatic first-pass effect 7. Renal excretion 2 Elimination in a nutshell Figure 1: Overview of the ADME processes that determine blood concentrations of drugs. In this lecture we focus on elimination (metabolism and excretion). 3 Elimination and the concentration-time curve (IV) 10 10 9 9 Distribution 8 8 Plasma Concentration (mcg/mL) Plasma Concentration (mcg/mL) 7 7 6 6 5 5 4 Elimination 3 4 2 1 0 3 0 10 20 30 40 0 5 10 15 20 Time (hr) Time (hr) Figure 2: Regardless of route of administration [here IV: linear scale (left), log scale (right)], the decline of concentrations sufficiently far in time from administration (here ∼ 6-7 hrs) characterizes elimination processes which are generally first-order. 4 Elimination and the concentration-time curve (EV) 40 2 10 Absorption + Distribution 35 Plasma Concentration (mcg/mL) Plasma Concentration (mcg/mL) 30 1 10 25 20 15 0 10 Elimination 10 5 -1 10 0 0 5 10 15 0 5 10 15 Time (hr) Time (hr) Figure 3: Regardless of route of administration [here EV: linear scale (left), log scale (right)], the decline of concentrations sufficiently far in time from administration (here ∼ 6-7 hrs) characterizes elimination processes which are generally first-order. 5 Quantifying the rate of elimination First-order elimination behavior allows us to define a half-life for elimination processes Terminal elimination half-life (t1/2 ) The time it takes for the blood concentration of a drug to decrease by 50% during the terminal phase of the concentration-time curve. It is inversely related to the terminal rate of drug elimination (kel ) as ln 2 Bigger half life t1/2 =. kel longer to eliminate diva Inversely proportional to the rapidity at which terminal concentrations decline (as quantified by the rate constant kel ) 6 Terminal elimination half-life visualized 10 101 7.07 Concentration (µg/mL) 8 7.07 3.54 6 1.77 100 4 3.54 2 1.77 t1/2 t1/2 t1/2 t1/2 0 10−1 0 2 4 6 8 10 0 2 4 6 8 10 Time (hr) Time (hr) Figure 4: The terminal elimination phase for most drugs conforms to first-order kinetics, allowing one to define t1/2. In each t1/2 , half of the initial concentration is removed from the blood (left: linear y-axis, right: logarithmic y-axis). Here, t1/2 = 2 hr and numbers associated with each horizontal dashed line give specific concentrations. 7 Quantifying overall elimination To compliment knowledge of the rate (i.e., rapidity) of elimination, we desire a parameter that captures the body’s overall ability to eliminate drug Ideally, this parameter would be: – Independent of the drug’s distribution (i.e., VD ) – Computable from the c(t) curve without any specific knowledge of the drug’s elimination mechanisms The ‘clearance’ concept was developed to meet this need 8 Quantifying overall elimination Clearance (Cl) The ratio of the time rate-of-change of total amount of drug in the body (∆x/∆t) to the measured blood concentration (Cblood ) at the same time: ∆x/∆t Body'sabilityto Cl ≡. eliminate drug cblood (t) Quantifies the inherent ability of the body to remove (i.e., clear) drug from the tissue in which concentrations are measured (here the blood) For linear PK systems, clearance is a constant (because of first-order kinetics: ∆x/∆t ∝ ∆c/∆t ∝ c) Primary application: determination of steady state plasma concentrations (see dosing lecture) 9 Total body clearance 10 Cl = 1 ∆xSchreiod largerareaunder c(t) ∆t i chamfme 8 curve lowerability to ∆x = Cl · c(t) · ∆t Concentration 6 ∆t euminate 4 dwa c(t) Dose = Cl · AU C 2 0 0 2 4 6 8 10 Cl = Dose/AU C Time Figure 5: How to compute total body clearance from the concentration-time curve. After rearranging the clearance definition, consider the limit where ∆t and ∆x get very, very small and then add up all the ∆x’s on the left-hand side and all the c(t) · ∆t’s on the right-hand side from t = 0 to a later time when c = 0. On the left we must have the total administered dose, and on the right we must have the total AU C. 10 Total body clearance is a composite notion We know that multiple processes contribute to clearance (e.g., hepatic metabolism and renal excretion) and that these processes generally act independently Thus, total clearance must be the sum of each individual tissue/organ clearance Total Body Clearance (Cltotal ) Total body clearance is the sum of specific tissue/organ clearances. For example, Cltotal = Clhepatic + Clrenal + Cllung + · · · 11 Models of organ specific clearance Because clearance is a composite notion, it is instructive to consider different models of clearance as applied to specific organs We must not confuse the definition of clearance with a clearance model – All clearance models are consistent with the definition – A model is the definition applied to a specific, concrete example – No single model applies in every case Hepatic and renal elimination are the most important sources of total body clearance so we consider models for both 12 Physiologic model of clearance Physiologic model of clearance Derived from the definition of clearance, this model expresses clearance as the product of blood flow (Q) to a ‘clearing organ’ and an extraction ratio E ∈ [0, 1]. ! patient'sCenous " Cart − Cven Cl = Q × E = Q Cart extraction ratio proportionbeingremoved 0 1 This model applies intuitively to the liver, where Q is total hepatic blood flow But this model does not apply to all situations! Consider a drug that is rapidly metabolized in the blood itself by esterases short 1 2 life acting metabolizesAS it flows 13 Extraction ratio 2.5 1.0 0.9 2.0 Extraction Ratio 1.5 Clh (L/min) 0.7 1.0 0.5 0.5 0.3 0.1 0 0 0.5 1.0 1.5 2.0 2.5 QL (L/min) Figure 6: Hepatic clearance as a function of total hepatic blood flow for various extrac- tion ratios. Drugs are often described as having ‘low’ (closer to zero) or ‘high’ (closer to one) hepatic extraction ratios. 14 Impact of extraction ratio visualized ∆Q Clearance ∆Cl1 ∆Cl2 0 0 Organ Blood Flow Figure 7: For high extraction ratio drugs (blue line), a change in blood flow (∆Q) produces a more significant change in clearance (∆Cl1 ) than for low extraction ratio drugs (purple line; ∆Cl2 ). For low extraction ratio drugs, the extraction ratio must change for clearance to be substantially influenced. 15 Model of renal clearance Model of renal clearance Derived from the definition of clearance, this model expresses renal clearance as curine · V̇urine if Curine Cart MMated Cl = , cart they urine habith where cart is the drug concentration in arterial blood, curine is there the drug concentration in urine, and V̇urine is urine production in volume per unit time. Renal clearance can involve multiple mechanisms including glomerular filtration, tubular secretion, and tubular reabsorption Total renal excretion = filtration + secretion − reabsorption 16 Components of renal excretion n c retio Ex Tm Rate ec retion S ration Filt Plasma Drug Concentration Figure 8: The rate of renal excretion decomposed into filtration and tubular secretion components (here we ignore reabsorption). While filtration is a linear process, at suf- ficiently high blood concentrations, secretory pathways will become saturated, leading to nonlinear behavior. Tm is the maximum rate of tubular secretion. 17 Interaction of Cl and VD Clearance volumeof distribution VD and Cl determine t1/2 Total body clearance and the volume of distribution determine the terminal elimination half-life according to drugnotinblood VD itselsewhere t1/2 = ln 2. Cl The primary clearing organs (liver and kidneys) rely on blood flow to deliver drug for elimination fromperspectiveblood – For a given Cl, as VD grows there is less drug circulating and available to be cleared such that t1/2 increases VD 12 – For a given VD , as Cl increases, t1/2 decreases because the body is more able to eliminate the drug CIT t 2 18 Cl and VD are independent (!) Students often want to believe that Cl and VD are somehow dependent on one another After all, we have that t1/2 = ln 2 VD /Cl, which can be rewritten as Cl = ln 2 VD /t1/2 or VD = t1/2 Cl/ ln 2 But Cl and VD are separately and independently defined; they are more fundamental parameters than t1/2 By analogy consider the following well-known relationship. What determines what? MAP = CO · SVR ⇔ CO = M AP/SV R ⇔ SV R = M AP/CO 19 Cl and VD are independent (!) 2 10 1 10 Clearance (L/hr) 0 10 -1 10 -2 10 0 1 2 3 4 10 10 10 10 10 Volume of Distribution (L) Figure 9: Scatter plot of Cl versus VD for a selection of 33 drugs. There is no discernible correlation between Cl and VD ; these parameters are independent. Blue lines represent lines of constant t1/2 = ln 2 · VD /Cl in factors of 10 from 0.1 hr (upper left) to 100,000 hr (lower right). 20 Example: meloxicam Meloxicam is a nonsteroidal anti-inflammatory drug with greater COX-2 selectivity than ‘older’ NSAIDs and is used to treat pain It’s pharmacokinetics have been studied in both the horse and donkey Species VD (mL/kg) Cl (mL/kg·hr) t1/2 (hr) Horse 270 35 5.3 Donkey 93 188 0.34 Shorter life 21 Example: meloxicam Horse Donkey 1 2lifeshorter slope steeper Figure 1—Mean ± SD serum meloxicam concentration as a Figure 10: Mean ± standard deviation serum meloxicam concentrations versus time in 5 healthy horses (solid circles) and 5 healthy donkeys (open circles) after IV administration of 0.6 mg/kg. 22 Metabolism (biotransformation) Chemical transformation of a parent drug to one or more metabolites is one form of elimination – These metabolites may or may not be active The primary organ for metabolism is the liver where biotransformation is enzymatically driven – Liver blood flow is high (≈ 25 − 30 mL/kg·min) – The liver contains a lot of enzymes – The liver also has an excretory function via bile production Biotransformation typically functions to increase a drug’s polarity and water solubility, thus retarding tissue distribution (i.e., confining drugs to the extracellular space) and facilitating drug excretion from the body The various metabolic pathways can generally be divided into Phase 1 and Phase 2 reactions abilituto disserve He23 p Phase 1 and 2 reactions Drug Phase 1 Metabolite small change Drug Phase 2 Conjugate Large charge Figure 11: Schematic of the interaction of Phase 1 and Phase 2 biotransformation reactions. Drugs typically undergo Phase 1 reactions before Phase 2 reactions (purple path). However, some drugs may only undergo Phase 1 reactions (blue path), only undergo Phase 2 reactions (yellow path), or undergo Phase 2 reactions before Phase 1 reactions (green dashed path). Regardless, the overall goal of these reactions is to create more polar compounds which are more readily sequestered to the extracellular space and thus more easily excreted. 24 Phase 1 and 2 reactions Phase I Phase II Oxidation Glucuronidation/glucosidation Cyt P450 dependent Sulfation Others Methylation Reduction Acetylation Hydrolysis Amino acid conjugation Hydration Glutathione conjugation Dethioacetylation Fatty acid conjugation Isomerization Figure 12: Primary drug metabolism reactions. Phase 1 reactions modify the substrate drug while Phase 2 reactions create conjugates. The CYP450 family is a very important group of enzymes that primarily catalyze oxidation reactions. 25 Example: morphine polar more subtexteffasses Figure 14: Morphine undergoes Phase 2 metabolism by a UGT (UDP- Glucuronosyltransferase) enzyme in the liver to morphine-3-glucuronide (M3G) and, shown here, morphine-6-glucuronide (M6G). These glucuronides are excreted in urine and bile. whes also a potentanalgesic against 27 beneficial 1 Example: drug interaction Levetiracetam concentrations in dogs 40 35 Concentration (µg/mL) 30 25 Levetiracetam only 20 Levetiracetam + phenobarbital L 15 10 When w 5 Phenobarb phenobarb 0 bc oxidation 0 2 4 6 8 10 12 14 16 18 20 22 24Induces Time (h) enzymes Figure 13: Plasma levetiracetam concentrations in dogs following administration of a single oral dose when given alone or following oral phenobarbital for 21 days. AU C decreased from 184 to 88 µg·hr/mL and Cl/F increased from 125 to 253 mL/kg·hr. This PK effect has been attributed to phenobarbital induction of oxidative enzymes that participate in levetiracetam elimination. 26 Hepatic first pass effect Intravenous administration S y st e m i c c i r c u l a t i o n Oral administration Biotransformation Liver Hepatic portal vein Intestines Oral drug Figure 15: Due to the anatomy of the portal circulation, orally administered drugs are subject to hepatic metabolism and excretion before they ever reach the systemic circulation. 28 Hepatic first pass effect IV oval shunt blood bypassesliver influencesPKof oral lidocaine Figure 16: Plasma lidocaine concentrations after oral (open circles) and IV (filled circles) administration of 10 mg/kg to 6 dogs. Left panel: normal dogs. Right panel: after construction of a portacaval shunt. While the IV curves are largely unchanged, the AUC of the oral curve on the right is larger than on the left. 29 oral comparedto IV dueto 1ˢᵗ passeffect