Bio 114 Exam 1 Notes PDF
Document Details
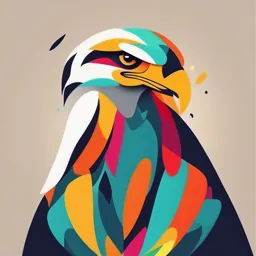
Uploaded by RomanticCombinatorics
State University of New York at Binghamton
Tags
Summary
These notes are an introduction to general biology. They cover fundamental concepts like scientific method, theory, and evolution, plus unifying themes like cell theory. The file is not a past paper and doesn't have an identifiable author or institution.
Full Transcript
Science Science is the process of identifying patterns and asking questions that help us to best understand those patterns. More specifically, science is the process of disproving hypotheses (specific, testable, rejectable statements) in an effort to focus on the true answer to a question. When...
Science Science is the process of identifying patterns and asking questions that help us to best understand those patterns. More specifically, science is the process of disproving hypotheses (specific, testable, rejectable statements) in an effort to focus on the true answer to a question. When several related phenomena are identified a much larger concept is described to explain them. This umbrella-like concept is what we call a theory. NOTE: in non-scientific terms, a theory is a statement that attempts to explain an event, and does not have much, or any, evidence to support it yet (sort of like a hypothesis). In science, a theory has a tremendous amount of evidence supporting the concept and patterns it describes. Theories are a way of bringing together multiple related, demonstrated hypotheses. Since scientific advancement is based on the assumptions of previous scientific discoveries, wide realms of scientific thought may be discarded by disproving a single hypothesis that supported one of the initial scientific assumptions. No amount of experimentation can ever prove me right; a single experiment can prove me wrong. -- Albert Einstein Such is the risk of scientific endeavors. However, because of this process, any hypothesis that has stood the test of time is likely one we can trust. Theories (and most scientific concepts) generally consist of 2 parts: Pattern and Process. Pattern refers to the general observations of repetition one sees within a system (This is akin to the observation phase of the scientific method). Process, on the other hand, is the mechanism, or way in which the pattern occurs. Frequently, there may be several processes involved in an identified pattern. This may depend on how specifically the pattern is considered. Additionally, processes may be divided into proximate vs. ultimate processes or reasons. Proximate refers to that which occurs in the short-term (usually measured in fractions of lifetimes), where ultimate refers to a longer timeframe (e.g., most of a lifetime or more). Example: While walking through the woods, you notice that under- and mid-story trees (those with leaves below the uppermost canopy), tend to have larger leaves than upper-story trees do. You also notice that this sometimes occurs within a single tree. That is, lower leaves tend to be larger than leaves higher up. This is your pattern. What is the process? This 1 question can be answered at many levels. You can think about it in terms of the physiology of the plant: what is the physiological mechanism involved in creating larger vs. smaller leaves (proximate)? Or you can ask it in a more ecological way: what environmental conditions exist that cause the tree to perform this pattern (proximate or ultimate, depending on timescale involved)? Also, underlying the whole system is an evolutionary question: what selective forces have caused this pattern and does this pattern increase the fitness (see below for definition) of the tree (ultimate)? II\. Unifying themes of Biology There are two unifying (or underlying) themes in biology: A. Cell Theory: All organisms are made of cells (a naturally occurring compartment bound by a thin, flexible plasma membrane, and contains chemicals in an aqueous solution that perform life functions), and all cells come from pre-existing cells. Implicit in this theory are two main ideas: a\. Since many of the limitations that exist among cells exist among all cells, studying cell biology is roughly the same as studying life (Which is Biology!). Therefore, in many ways, understanding how an organism works is a function of understanding the structure and function of its cells. Obviously, this is an over- exaggeration (if it were literal, the only branch of Biology would be Cell Biology!). It is not the whole story (see Emergent Properties below.). b\. Life is continuous. The 60 trillion cells that make up your body came from a single-celled, fertilized egg. That zygote came from the fusion of your parents' gametes (sperm and egg), each of which came from a single-celled, fertilized egg, which came from their parents' gametes, and so on. One conclusion that can be drawn from Cell Theory, is that if you trace a cell's lineage back far enough, you will conclude that all cells are descended from a common ancestral cell. Prior to the original description of Cell Theory, it was assumed that cells spontaneously generated. That is, cells come to being from certain conditions on their own. B. Evolutionary Theory: All life, as we know it, is the product of evolutionary processes. Evolution is the heritable (i.e. genetic) change in a population over time. If certain heritable traits help individuals produce more offspring, then those traits become more common in the population over time. The primary mechanism of evolution is Natural Selection (which we will cover later). Individuals live, give birth, and die, but their evolutionary success can only be seen over a time longer than their lifespan and by comparing their success with that of other members of the population. Living a year and giving birth to three offspring is relatively 2 successful if the average life span is 10 months with two offspring but relatively unsuccessful if the average is two years with six offspring. In the first case, the individual is favored by evolution, but the same individual is not favored in the second scenario. Evolution is a property of populations. There are two general ideas within evolutionary theory: a\. Evolution explains variation, how that variation came about, and what can happen if environmental conditions change. b\. All species are related to each other through common ancestry. Natural Selection acts on individuals, but evolutionary change affects only populations. III\. The Biological Hierarchy The biological hierarchy is a framework of organization regarding all life around us. It provides us with a simple way of guaranteeing we are talking about the same terms as we consider life across varying scales. This hierarchy may seem simplistic and out of place in a college Biology course. However, it has been my experience that when talking to students about biological concepts, the students frequently jump between levels of the hierarchy without meaning to and, as a result, speak incorrectly about their topic. For example, I frequently hear students referring to the "evolution of an individual", when this is incorrect. Evolution is a process that happens at the population level, not at the individual or organismal level. The Biological Hierarchy levels consist of: atoms, molecules, organelles, cells, tissues, organs and organ systems, organisms, populations, communities, ecosystems, and the biosphere. We will not be covering much information on the atoms, molecules, organelles, cells, tissues, and organ systems. These are more the realm of Biol 113. We will, however, cover the remaining levels throughout the course (with occasional dips into the former levels). A. Organisms: Our course is primarily concerned with this level of the hierarchy on up. a\. Organisms are individuals. They are typically a collection of organ systems. However, remember that organisms can be single-celled. b\. Individuals are acted on by nat. sel. c\. The organismal approach to studying biology is primarily concerned with how the organism behaves, its place in its population, community, etc and how it survives as a result of its behaviors and physiology. B. Populations are collections of individuals of the same species. Typically interbreeding. a\. Evolutionary change affects the population. b\. Population biologists are concerned with population growth and evolutionary processes and other changes through time. 3 C. Communities are a collection of populations of different species living together in the same area. a\. Boundaries may be set up by natural structure (eg mountains, islands, etc) or arbitrarily determined by us. b\. Community biologists are concerned with diversity of species and relationships each species has with other species. D. Ecosystems are communities plus their abiotic factors (water, temperature, geology, sunlight, etc.). a\. Although ecosystems are communities plus abiotic factors, we frequently refer to ecosystems as much larger agents than communities (e.g., the Sonoran Desert ecosystem). Additionally, for many people, ecosystems are the same as biomes. However, generally, biome refers to a collection of very similar ecosystems, largely bounded by global influences like weather and climate (e.g., Tropical Rainforest Biome). So, be careful that you know to which scale you are referring when talking about such things. b\. Ecosystem biologists are concerned with, among other things, energy flow and matter cycling E. The Biosphere is all the ecosystems (biomes) put together. a\. All that gets in is sunlight. All that leaves is heat energy. Everything else stays here - for good or bad. b\. Biosphere scientists are concerned with climate and climate change and other global issues. Notes About the hierarchy: \- There is similarity between individuals within each level, with respect to structural and functional complexity (even if they have different functions). \- Units of each level define the next level. Typically, the units of one level come together to create the next level (eg. Several organ systems come together to make an organism several organisms come together to make a population, etc.). \- A good question to ask at this point is: why organize the field of Biology in such a manner? Why not just study how individual plants and animals react to different factors and add up these responses in some fashion to predict what to expect from nature? The answer lies in the concept of emergent properties. Emergent property: The collection of the units at one level takes on a trait that is greater than the sum of the parts. This property is defined as (or helps to define) the next higher level in the hierarchy. Emergent properties are what provided the next higher level in the hierarchy an 4 evolutionary advantage. We'll see this again later when we cover general evolutionary trends. To understand this, you have to have a clear idea of what the hierarchy is. The hierarchy is a means of grouping units of something (individual animals, say) into nested levels of groups. Thus, in an organism, atoms are grouped into kinds of molecules, which are grouped into different kinds of organelles, which are grouped into different kinds of cells, which are grouped into different kinds of tissues, etc. This grouping is not just for convenience but is a real grouping (i.e. it exists in nature). The biological hierarchy is real also, and we study it as such because some phenomena are only observable by studying a whole level of organization in the hierarchy. The set of phenomena that can be explained only by looking at an entire hierarchical level are the emergent properties of that level. We study populations, because one may not be able to understand why a species is rare just by looking at how many offspring an organism can produce. The same reasoning leads us to consider community ecology as a separate subdiscipline, as it may be impossible to understand the change in one population without understanding its interactions with other species. So, we have a hierarchy of biological subdisciplines because nature is hierarchical and there are phenomena that are understood only when one studies the appropriate level within the hierarchy. Finally, having argued for separate subdisciplines, I want to caution you about considering them as independent of one another. All belong to the same hierarchy. As a result, changes in one of the levels frequently brings about a change in the other levels as well. \- More examples of emergent properties: Organ systems by themselves cannot reproduce themselves (not even a reproductive system), but an organism can. Therefore, you would describe reproduction as an emergent property of the organismal level. (You might be able to argue that it's really an emergent property of the population level for sexually reproducing species. Can you explain why?) Individuals do not have the emergent benefits of a social group (e.g. Safety in numbers, food sharing, etc.), but they do when you put them in a group. Evolution is the big emergent property of populations. Individuals do not evolve. Populations do. But natural selection acts on the individual. Intro To Darwin and Evolution A. For a long time, before the mid-1800s, the world new many important ideas about variation, species diversity, and species change. We knew: a\. Organisms have offspring similar to themselves. b\. We could select individual plants and animals on our farms to breed and produce offspring that we considered beneficial. c\. There exists a large amount of diversity/variation among organisms within a species. d\. Differences among the members of a population mean that not all are affected in the same way by ecological factors e\. The variation between species may mean that not all areas that can do so will support a population of a particular species or actually have a population present. f\. Typically, individuals produce more offspring than what the environment can support. g\. We also believed: i\. All organisms were created by a divine being (Special Creation) as recently as 6000 years ago. ii\. Species have never changed and are unchangeable. iii\. Aristotle's Great Chain of Being: All species are organized into a sequence based on increased size and complexity, with humans at the top. iv\. Variation between individuals is unimportant or misleading. B. Jean-Baptiste de Lamarck -- a\. First to state that species have changed through time (first formal evolutionary theory). b\. Thoughts were based on Aristotle's typological thinking and simple organisms spontaneously generated. c\. Species change through time via acquired characteristics -- That is, individuals change as a result of environmental pressures and then pass those traits to offspring. (Giraffe neck, and my son as examples) C. Along comes Darwin Although Charles Darwin and Russel Wallace independently came up with the theory of evolution by natural selection at roughly the same time, Darwin's name is more typically associated with its creation. As a result, from here on when talking about evolution, we will refer to Darwin only. While traveling on the H.M.S. Beagle around the world from 1831-1836, Darwin amassed a huge amount of evidence that there existed a tremendous amount of variation within species, between similar species, and between distinct species. He also noticed that the traits organisms had seemed to change and be predictable based on differences seen in environmental factors like weather, climate, geology, etc. 1 As a result of the accumulation of this evidence, Darwin went on to publish his now- famous book: On the Origin of Species. Within this and subsequent books, Darwin concluded: a\. Variation, in conjunction with environmental pressure, is the key to understanding diversity and how and why species change. b\. Darwin's theory on the Origin of Species by Natural Selection had 3 components: i\. All species have a common ancestor. ii\. All species show changes in characteristics through time. iii\. All species show changes in characteristics in different environments. c\. These three (esp. the 3rd) lead us to our Modern Theory of Natural Selection D. Modern Theory of Natural Selection a\. Evolution is the change in genetic (allelic) frequency within a population over time. b\. Natural Selection is a process by which evolution can occur. c\. Natural Selection requires: i\. Trait variation in a population. Variation is the fuel of natural selection. Without it there are no traits to select for and against. Future generations will be no different than previous generations (minus genetic drift). Almost all phenotypes have variation. These exist on a continuum, not normally an either/or situation. ii\. Heritability. The traits in question need to be heritable, that is, passed from parent to offspring by genes. This is where Lamarck's ideas fell apart. Acquired characteristics are not normally passed from one generation to another (However, pay attention when we get to the end of "Mendelian Inheritance".) iii\. Differential survival. There needs to be a difference in survival to reproductive age based on the condition of the trait (which leads to Differential reproduction below). Some individuals will produce more breeding offspring than others will. Non-breeding individuals are no different than dead individuals. Differential survival also refers to differences in the persistence of genes (traits) across time within a genetic line within a population. iv\. Differential reproduction. As a result of a condition of the trait, individuals will have more breeding offspring than others, thus passing the genes for that condition into the next generation As a result of the greater survivorship and reproductive success of individuals possessing a particular trait, they are considered to be "naturally selected", i.e., selected to survive in nature because of the traits they possess. Remember, it is the relative number of breeding offspring that counts: a king salmon producing 100 eggs is not very good when its neighbors are producing thousands! The individual producing more offspring is considered "more fit". Fitness, here, refers to the number of viable offspring you produce in your lifetime. "Survival 2 of the fittest" (a line so often quoted, but not understood) does not refer to the strength or constitution of an individual, but how many offspring that individual can produce or potential fitness -- how many offspring can they produce. They often mean the same thing, since those that are bigger and stronger frequently have more offspring, but this does not always work. Actually, I don't remember ever hearing a biology professor use the term "Survival of the fittest". Maybe because it frequently means something else entirely to the non-scientific community, scientists have steered clear of using it. d\. Where are the 4 components of NS in the example of your sinus infection (from the video lecture provided)? e\. Natural selection results in traits that are selected for. Adaptations. f\. Adaptation is: i\. A heritable trait that increases the relative fitness of individuals having the trait. ii\. A process by which individuals within a population acquire traits that increase their relative fitness. Here, one organism can be "better adapted" to a particular environment because the process of "adaptation" has taken place over a longer period of time. The term "adaptation" as a process is similar to "natural selection", although "adaptation" implies the formation of a fitness-enhancing trait rather than what/how selective agents operate. Artificial Selection Having defined a mechanism of genetic change in a population over time as Natural Selection, you would think there must be such a thing as Artificial Selection. Indeed there is. Artificial selection is a similar process to natural selection. In the case of artificial selection, however, the selecting agents (that which drives the evolutionary process) are humans. For thousands of years, people have been selecting individuals from their domesticated stock of plants and animals to breed. These organisms have been selected because they possess some desirable trait (e.g., high food yield, ability to assist humans, etc.), and the resulting offspring would continue that trait as well. This sort of selection is considered "artificial" because the selective pressure is not occurring as a result of non-human environmental conditions, and, more importantly, the resulting desirable traits do not necessarily allow the individuals to survive and reproduce more offspring in the wild. That is to say, the resulting traits are not adaptive in the wild, even if they are so in captivity. II\. Evidence for Evolution There are three main conclusions from evolutionary theory. 1) Species are related, 2) Species (and species diversity) change over time, and 3) Evolution should be able to be seen in the short- term. The first two of these, are typically referred to as Macroevolution, the change of one major taxonomic group into another (e.g., fish to amphibians to reptiles), or the creation, or extinction, of species. The third is often referred to as Microevolution, the change in a 3 population over generations that helps to separate populations from each other genetically. The accumulation of microevolution over a long period may result in the creation of new species (macroevolution). A. Macroevolution a\. Evidence that some species are related. i\. Geographic proximity of similar, non-interbreeding species. The birds in the figure (in the video) are 4 different species of mocking birds on the Galapagos Islands. Their extreme similarity and close proximity suggest relatedness. ii\. Homology -- similar traits in separate species due to a shared common ancestor 1\. Genetic Homology - similar gene sequences between individuals of different species. 2\. Developmental Homology - similarities in morphology of embryos of different species. 3\. Structural Homology- similarities in structure of body parts of different species. b\. Evidence that species (and species diversity) change over time. i\. Fossil Record 1\. Not all species were together at one time 2\. Extinction has taken place 3\. Transitional forms exist 4\. Major increases in species complexity takes billions of years 5\. Life began in the sea ii\. Vestigial Traits structures in organisms commonly found in the species that serve little or no function (as compared to an Atavism -- a vestigial trait found in very few individuals within a species). B. Microevolution We have already considered many aspects of microevolution. Consider the case of antibiotic resistance in the example of you fighting your sinus infection. III\. Misconceptions about Evolution Probably no other biological concept has generated as much controversy among the non- scientific community as evolutionary theory has. The unfortunate reality of the situation is that many of the problems people have with evolution are largely based in inaccuracies and misconceptions. Worse still, once these inaccuracies are used in arguments against evolution, 4 either in private or public settings, the listeners believe them and use them in their own arguments. What follows is a list of commonly held misconceptions about evolution. a\. If evolution is true, then there is no God. b\. Humans evolved from apes. c\. Individuals evolve. d\. Adaptation occurs because a species needs/wants it. e\. Evolution always results in a more complex or better organism f\. Animals do things for the good of the species g\. All traits are adaptive h\. Functional traits have unlimited adaptive potential IV\. Evolution as a scientific framework Apart from explaining how species are related and how species have changed over time, evolution provides us with a scientific framework within which we can investigate the world around us. Science is based on testable, rejectable hypotheses. Evolutionary theory allows us to predict events (form hypotheses) within biological systems as well. We can then design experiments to test for those events, and, thus, better our understanding of our world. Consider the example of Homicide (Daly and Wilson 1988) in the lecture video. V. Evolutionary trends. There have been some long-term trends that resulted from macroevolutionary processes over billions of years. These include: i\. An increase in multicellularity j\. An increase in complexity k\. An increase in ways to capture energy for use l\. An increase in ways to deal with the environment - biotic and abiotic m\. An increase in diversity (snowball effect)Introduction The beauty in evolution is not in how organisms have adaptations that are perfectly created to allow survival in their environment, but in that they are well-suited for survival at all. Though natural selection results in adaptation, nature is full of examples where organisms are not ideally suited for survival in their current environment. There are several reasons why this is so. A. Adaptations are compromises. The adaptive traits of most organisms are compromises produced by competing selection pressures. To successfully exist in any environment, organisms must have several adaptations (whether behavioral or morphological) to survive. Often, these adaptations exist in direct conflict with each other, one providing success in a situation, while another acting as a detriment. For example, sea turtles must lay their eggs on beaches because their embryos cannot acquire enough oxygen in water to fulfill developmental needs. Females are excellent swimmers due to the presence of flippers. However, these flippers are not well- suited for movement on land. If the sea turtle had legs allowing it to move more freely on land, it would not be nearly as efficient at swimming in the water. A sea turtle is poorly adapted for life on land, despite spending at least a portion of its life on land. B. The environment changes over time. When selection occurs in a population, it preserves alleles that are successful under the prevailing environmental conditions. That is, each generation is adapted for survival in the environment that existed during their parents' generation. When the environment changes, adaptations lag behind, and an individual may look maladapted. If the environment changes with every generation, adaptations will always lag behind, and, as a result, may never look appropriate given the current environment. In this specific case of constant change, a species may evolve a more generalistic trait that allows some success in any environment, which may look imperfect, and less than ideal, in any one particular environmental condition. C. Adaptations are limited by historical constraints. Natural selection is not an engineer that designs new organisms from scratch. Instead, it acts on new mutations and existing genetic variation. Because new mutations are fairly rare, natural selection works primarily with alleles that have been present for many generations. Thus, adaptive changes in the morphology of a species are often based on small modifications of existing structures. For example, the bipedal posture of humans evolved from the quadrupedal posture of our ancestors. Natural selection did not produce an entirely new skeletal design to accompany this radical behavioral shift. Instead, existing characteristics of the spinal column, and corresponding musculature of the legs and back, were modified, albeit imperfectly, for an upright stance. The result is a species that has the benefits of an upright posture and increased agility but is more likely to suffer with chronic back and leg problems. One may ask, therefore, how do you determine if an adaptation is truly novel (arising from a mutation in the genetic line) or if it is only a modification of previously existing behavioral or morphological phenomena? Generally speaking, almost all adaptations are built as slight modifications of ancestral traits and the variation that existed concurrently with them. Additionally, many adaptations are seen as imperfect solutions to current environmental problems, resulting from the reasons stated in A through C above. While examining a population through time, if you see a novel adaptation that in no way resembles the behaviors or mechanisms of the ancestor, then you may have a truly novel genotype and resulting phenotype. Below are a few terms that are frequently associated with this sort of discussion. 1\) Adaptation: a phenotype that is selected for within a population. Heritable trait that increases the fitness of the individual with that trait. 2\) Contrivance: an adaptation in an organism, where the adaptation exists as a result of the modification of the original (or previous) adaptation in an ancestor. In other words, a contrivance is an adaptation that has been evolutionarily modified during the course of its inheritance from the ancestral condition (the exaptation). 3\) Exaptation (pre-adaptation): A trait that was adaptive under a prior set of conditions and later provides the initial stage for evolution of a new adaptation (contrivance) under a new set of conditions (in descendants). In other words, an exaptation is an adaptation possessed by an ancestor that changes over evolutionary time and exists as an adaptation in the descendant (which is then called a contrivance). For example, previously, we spoke of birds evolving from reptiles and reptilian scales evolved into bird feathers. In this case, we would call the ancestral scales an exaptation and modern feathers the contrivance. There are several things to note here: 1\. Both contrivances and exaptations are adaptations. The trait increases the fitness of the possessor. 2\. In this discussion, the inclusion of one of the terms dictates the requirement of the other term. In other words, you cannot have a contrivance without an exaptation and vice versa. 3\. Even though the word exaptation is a reference to the ancestral condition, the trait itself may continue to exist in a separate line from the descendant described. For example, although reptilian scales (the exaptation) evolved into bird feathers (the contrivance), modern reptiles still possess scales. 4\. Exaptations/contrivances may exist as structural or behavioral traits. 5\. The exaptation/contrivance relationship may change over time, where a contrivance may exist at one time in evolutionary history, but can also be an exaptation to a future contrivance. For example, it is not likely that the original feathers on the ancestors to modern birds looked like modern feathers. Those feathers evolved over time to take on the functions of current feathers. Depending on where on the timeline of the evolution of feathers you are looking, any one feather may be an exaptation and contrivance. 4\) Vestigial trait: a trait the entire species has that is no longer used or has no use in the present environment, but was likely an adaptation for an ancestor. 5\) Atavism: a trait possessed by only a few members of the population that is no longer used or has no use in the present environment, but was likely an adaptation for an ancestor. (Although technically not correct, think of it as a vestigial trait that only a few possess). 6\) Homology vs. Homoplasy As covered in a previous lecture, homology is the similarity in traits between individuals as a result of the inheritance of those traits from a common ancestor. Homoplasy, on the other hand, is when organisms have traits in common, but do not have a common ancestor that provided them with those traits. Typically, the traits in question arose from the independent evolution of the traits by the different species or genetic lines. The most common form of homoplasy is convergent evolution: the independent natural selection of similar traits by unrelated individuals. For example, the glass snake has several traits in common with regular snakes, including being limbless. However, the glass snake is really a lizard, having, among other lizardly things, moveable eyelids and external ear openings. The most recent common ancestor between the true snakes and glass snake had legs. The genetic line that eventually gave rise to the true snakes evolved the loss of limbs for ease of movement through burrows. Similarly, the genetic line that gave rise to the glass snake also evolved to lose its limbs so that it too could move through burrows more easily. However, since their most recent common ancestor had limbs, we say that the two groups (true snakes and glass snakes) have adaptations that converged to be similar as a result of similar environmental pressures. Keep in mind, an individual is made of lots of different traits. As a result, an individual may be composed of a mix of homologies and homoplasies when compared to a member of another species.Genetic Heritability: Heritability of specific phenotypes is the key to understanding what Charles Darwin meant when he described natural selection as "descent with modification". Darwinian evolution requires heritability of phenotypes from one generation to the next. Without heritability, the variants that are selected for or against by the environment would be lost and evolution would not take place. In his book: On the origin of Species, Darwin acknowledged that his hypotheses lacked a mechanism by which variation could arise and how traits are passed from parent to offspring. Interestingly, the mechanism for inheritance was described within a few years after Darwin's book was published, but the world did not notice. This, and the following few lectures will cover those details. We now know that phenotypes (measurable traits) are caused by genotypes (genes that code for traits). However, you don't always get a predictable phenotype from a specific genotype. And not everything is so hardwired. (See below.). And it is that genetic variation that encodes for phenotypic variation. If genes did not cause phenotypes, there would be no variation among individuals within a population (it's the variation in phenotypes that allows the environment to select for or against members of a population). Variation is important for species survival in the face of selection. But genes are selfish and don't work for the good of the species. Consider Richard Dawkins (The Selfish Gene), who said that, because genes are inherited, it is every gene's "desire" to replicate itself and get to the next generation. The phenotype is really just the mechanism that genes use to get from one generation to the next. Much of what we call "instinct" are the genes using our phenotypes to protect themselves until they can be passed. II\. Genes: A. Definitions: 1\. Genome -- all of the hereditary information within an individual (includes non- gene stretches of DNA) 2\. Gene pool -- all the alleles of all the genes within a population. Each individual has only a small set of the genes in the population, unless the population is made of clones. 3\. Genotype -- all of the alleles of all the genes within an individual or may refer to a specific set of alleles of a set of genes under study (compare Phenotype). 4\. Genes -- section of DNA found on chromosomes that encodes for a polypeptide, which, in turn, causes (directly or indirectly) a trait (phenotype), may also regulate the activity of other genes. 5\. Locus -- (plural loci) the location of a gene on a chromosome. In a population the locus for a specific gene is considered to be the same throughout. 6\. Alleles -- particular versions of a gene that occur at the same locus on homologous chromosomes. B. Does Genotype Really Equal Phenotype? 1\. Similar genotypes can result in different phenotypes. Environmental pressures can have a dramatic effect on how phenotypes are expressed. a\. Two otherwise identical plants can be raised in two settings- one with plenty of light and the other with less light. The result is that one plant will look different than the other. b\. Can happen with people too. Even identical twins do not encounter the exact same developmental environments, which affect "regulator" genes, which result in slight differences in appearance, esp. as they age. 2\. Similar phenotypes can result from different genotypes. a\. For example: convergent evolution of gray (timber) wolf and Tasmanian wolf. The Tasmanian wolf is a marsupial (pouched mammal -- compared to the placental traits of the true wolves) and went extinct in 1936\. The similarities between them and true wolves include: sharp teeth (with many similar teeth forms), powerful jaws, raised heels, and same general body form. However, their most recent common ancestor looked nothing like a wolf. 3\. Keep in mind, all traits of individuals in similar environments, but possessing different genotypes, will not be the same. Nor will those with identical genotypes necessarily have the same phenotypes, due to differing environmental pressures. To have truly identical phenotypes, you need identical genotypes and homogeneous developmental conditions, which is nearly impossible for organisms not grown in a petri dish. III\. Variation in Eukaryotes A. Characteristics: 1\. In cells, genes are carried on a structure made of DNA and associated proteins, called a chromosome. Chromosomes occur in sets of chromosomes, and depending on the species, there may be multiple chromosomes within a set (eg. Humans have 23 chromosomes in each set). These different kinds of chromosomes, within a set, typically have some morphological distinctness among them. 2\. In Eukaryotes, especially, there may be more than one set of chromosomes in each cell. The cell's ploidy refers to the number of chromosomes in a set and the number of sets of chromosomes a cell contains. 3\. Homologous pairs of chromosomes are typical in eukaryote organisms, one copy of the same chromosome from each parent in the same nucleus (maternal= that from the female parent; paternal= that from the male parent) (2n= diploid). They have the same genes, but they can differ in their effects on gene expression based on the alleles present. Each parent provides a complete set of chromosomes to the offspring. 4\. Individuals may be haploid or diploid, meaning they may have just one copy (haploid = 1n), or a homologous pair (diploid = 2n), of each kind of chromosome. For example: ants, bees and wasps have haploid males but diploid females; most of what we see in mosses is haploid; and many organisms alternate between sexual (haploid) and asexual (diploid) generations (called Alternation of Generations). 5\. Dominance can occur where one allele of a gene is expressed in the phenotype even in the presence of another (recessive) allele at the same locus in diploid individuals. B. Meiosis -- Meiosis is the process of the production of gametes: egg and sperm, produced by individuals, by halving the number of chromosomes and placing them into gametic cells. A haploid gamete of one parent is joined with a gamete (also haploid) from the other parent to create a cell (zygote) with the proper number of chromosomes (diploid). This cell grows and develops to become a reproductive individual and the process begins again. Meiosis involves 2 cell divisions Meiosis I and II, both of which help to increase variation. 1\. Homologous pairs join up and exchange genetic material. During early meiosis I pairs of chromosomes (sister chromatids) come together with their homologues (one from one parent, the other homolog from the other parent). The actual mechanism involved in this attraction is unknown. The resulting tetrad (set of 4 chromatids) consists of two sister chromatids from the paternal homolog, and two sisters from the maternal homolog. Chromatids from the parental homologs (that is, one maternal and one paternal) are referred to as non-sister chromatids. 2\. First area of variation. Non-sister chromatids link up forming a chiasma. At this point, non-sister chromatids exchange genetic information by the maternal and paternal chromatids breaking and rejoining the remainder of the other chromatid, causing a crossover of genetic material. 3\. Second area of variation. Later in meiosis I tetrads migrate and line up along the center line of the cell. The orientation in which they line up is random with respect to other tetrads. As a result, the daughter cell may end up with all paternal, all maternal, or some combination of maternal and paternal homologs. 4\. Third area of variation. In meiosis II, sister chromatids separate into gametes with a single copy of each gene occurring in each gametic cell. The alignment prior to separation is again random, causing the genotype within the gametes to occur randomly. Because sexual reproduction requires two individuals, you could say that there is yet another source of variation to the puzzle of reproduction: that of the mate. All of the above variation occurs to form the gametes in one of the partners. It is occurring in the other partner as well, thus, in a way, adding to the variation. C. How much variation? 1\. Without cross-over: 2 homologous pairs yields 4 gametes (2n where n=haploid number of chromosomes). What about humans? 2\. With cross-over: the number of different gametes is almost infinite, because cross-over occurs randomly, and can occur anywhere along the chromosome. D. Other Sources of Variation: 1\. Mutations from a change in nucleotide sequence. Mutations caused by environmental mutagens like radiation, chemicals, and physical irritation. a\. Most mutations are not deleterious -- occur in junk DNA, are small, and not expressed. (These are used to measure genetic relationship of taxa. These mutations build up over time and are not selected for or against (since they are not expressed). The greater the differences between individuals, the longer their lineages have been apart.) b\. Most expressed mutations are harmful and are removed from the population by natural selection. 2\. Mutations from replication errors occur and are expressed much more frequently 3\. Nondisjunction -- during cell divisions in meiosis I and II, one cell ends up with both pairs of homologs of one chromosome (or both sister chromatids if occurring in meiosis II). The rest of meiosis occurs normally and 2 gametes are left with an extra chromosome (n+1) and 2 are left with one chromosome too few (n-1). a\. Example Down Syndrome is trisomy (3 copies of the chromosome after fertilization) at chromosome 21 (trisomy 21). n-1 is monosomy b\. Too many or too few chromosomes are referred to as aneuploidy Happens in 10% of meiotic divisions, but most are not expressed, since many end as fetal death. 4\. Unequal crossing over -- where one arm of a chromatid ends up longer than its sister Ex. Huntington's Disease IV\. What about asexually reproducing organisms? A. Here's the problem: Asexual organisms like bacteria typically reproduce by a process similar to mitosis, which is simply a splitting of the cell into two equal daughter cells. As mature cells, they are haploid. When they get large enough, they copy their genetic makeup and split. As a result, each generation would look the same and sexual organisms would not have evolved. Neither would different types of asexual organisms. So where do species like bacteria get their variation? They too can undergo mutations, in addition to three new possibilities. B. Transformation: -- bacterial cells take up DNA fragments from other cells and either incorporate into the bacterium's DNA or may be left as a separate plasmid which replicates independently from the parent cell. We have used this phenomenon to create stuff in the lab (e.g., golden rice, oil-eating bacteria). C. Transduction: Viral capsids attach to bacterial cells and inject their DNA, which fragments the host's DNA. Killing of the host cell releases both kinds of fragments into the environment. Other bacterial cells will encounter capsids with viral DNA, viral +bacterial DNA and/or just bacterial DNA. When the latter, the new bacterial DNA gets replicated with the new host's DNA. D. Conjugation: Cytoplasmic tube made from cell with a plasmid to a cell without the plasmid and the plasmid is transferred. Sometimes just a fragment of the plasmid is transferred, which introduces a new variant into the species.An Introduction to Mendelian Genetics: a\. When Darwin published his work on the origin, diversity, and changing of species through time, he acknowledged that he did not know the mechanism by which traits were passed from parent to offspring. Although he knew they were passed. Practical geneticists (i.e., farmers) had been demonstrating that for millennia! b\. In 1866 Gregor Mendel, an Austrian monk, biologist, mathematician, and gardener, published a paper announcing his findings about pea plant inheritance patterns and a couple of rules that made inheritance itself predictable. Mendel's findings provided an exact explanation of how traits are inherited, thus filling in the gaps of Darwin's work. c\. This link between evolution and inheritance largely went unnoticed by the scientific community (and even by Mendel himself) for a few reasons. The first was that the world was still reeling from Darwin's book. Darwin's message had far-reaching impacts that were being dealt with on a scientific, religious, and social scale, the likes of which the world had never seen. Second, Mendel's work was mathematical in nature, and even this relatively simple math (by today's standards) was considered too complex and out of place for most scientists who were interested in Biology. Third, in the middle 1800's information did not spread like it does today. Today you can quickly do an internet search to get information on your point of interest. A web search on Google for the word "inheritance patterns" today yields over 2 million hits. A search for inheritance in BIOSIS (a common search engine for primary biological literature) yields over 40,000 articles. The scientists of Mendel's and Darwin's time did not have this kind of access to information. If a scientific discovery was not Earth-shattering (like evolutionary theory), it went unnoticed by most. d\. Mendel's work went unnoticed until around 1900 when 3 research teams, working independently, formed the same conclusions that Mendel did. During their literature searches, they discovered that Mendel had already published this information. The three teams re-published Mendel's work, using their own data as support to his findings. Mendel finally got the credit he deserved. He had been dead for sixteen years. II\. Before Mendel: a\. Practical genetics- For millennia, farmers knew that you could select certain livestock/plants that provided some benefit and breed them. The resulting offspring would frequently provide the same benefits their parents did. i\. Ex. Jacob's herds in Genesis 29 and 30. b\. Blending- For over a century prior to Mendel's work scientists believed that offspring traits should be a blend of their parents. i\. Ex. A black cow mated with a white bull should result in a gray calf (or at least one with black and white patches in its fur). c\. Acquired characteristics- See previous notes about Lamarck. III\. Mendel's Experiments: a\. Mendel's 2 guiding questions: i\. Why do offspring resemble parents? ii\. Why are most offspring not 'blends' of parents? b\. Mendel worked with pea plants (Pisum spp.). Pea plants are self-fertilizing if undisturbed. Petals on pea flowers enclose the pistil and stamens, but can be pulled apart to cross pollinate plants using an artist's brush to transfer pollen from the anther of one plant to the pistil of the other. Cutting off the anthers from the recipient plant prevents self -pollination. Pea plants readily produce pure lines (homozygous condition at each locus) as a result of self-pollination. This was known and exploited by Mendel to produce pure lines of various traits, such as wrinkled and round peas. c\. Question: Are offspring blends of parents?: i\. Prediction: If blending occurs, then a cross of pure-breeding wrinkled and round peas (P generation) would produce all mildly wrinkled F1 offspring. And self- fertilizing of the F1 offspring would produce all mildly wrinkled offspring in the F2 generation. ii\. Results: All F1 peas were round and indistinguishable from those of the round parent, discounting blending. F2 offspring had a ratio of 3:1 round to wrinkled, further evidence that there was no blending. iii\. Interpretation 1: No blending. Mendel proposed that both the round and wrinkled traits were present in the F1 generation and that the wrinkled trait was not expressed in the F1 generation because the round trait dominated it. Hence dominant and recessive traits. iv\. Interpretation 2: Particles occur in pairs, and in order to occur in the 3:1 ratio in F2, the particles must segregate into the gametes (egg and sperm) before they leave the reproductive structures. v\. Mendel's Law of Segregation: Particles (alleles) separate, without dilution, into gametes where each gamete contains one particle (allele) of each trait (gene). Monohybrid Punnett Square for a cross of Round (R) and Wrinkled (r) peas. (Use this space to create your own Punnett Square map of the monohybrid cross.) d\. Question: Are different traits inherited together or separately? Mendel chose 2 traits: seed color and shape. He knew that yellow is dominant over green pea color and round is dominant over wrinkled. He crossed parents with both traits. He took pure parental plants that produced round, yellow peas and crossed with purebred green, wrinkled peas. i\. Prediction: If the 2 traits sorted dependently (ie, occurred together only), he would get one set of results in F2 (round yellow OR wrinkled green). If they assorted independently, he would get an entirely different result in the F2. ii\. Results: Round, yellow 315 (9/16), wrinkled, yellow 101 (3/16), Round, green 108 (3/16), and wrinkled, green 32 (1/16). iii\. Interpretation: Particles (alleles) of one particular trait (gene) are passed on to offspring independently of each other- Mendel's Law of Independent Assortment. 1\. Only works for genes occurring on different chromosomes. 2\. Remember, Mendel still didn't know about chromosomes. He thought about his "particles" as independent little bodies floating around in the cells. Almost as if each gene had its own chromosome, not many, many genes on a few chromosomes. 3\. Those genes on the same chromosome we'll talk about later. Dihybrid Punnett Square for a cross of Round (R), Yellow (Y) and Wrinkled (r), Green (y) peas. (Use this space to create your own Punnett Square map of the dihybrid cross.) e\. The Test Cross was used to determine the genotype of an individual showing dominant traits. If an individual displays a dominant trait, like yellow peas, how do you know whether it is homozygous dominant (having identical dominant alleles at the locus), or heterozygous (having different alleles at the locus)? A Test Cross is when this individual is crossed with a homozygous recessive individual. The resulting offspring phenotype distribution tells you what the unknown parent is. T.H Morgan worked with fruitflies. i. Morgan discovered a white-eyed male fruit fly in a population of wild type, red-eyed individuals. ii. Mated male with a red-eyed female. Resulting F1 offspring had red eyes. He concluded that white eyes is recessive to red eyes. iii. He performed a Reciprocal Cross: crossing the opposite way (in this case, switching the traits of male and female parents). He mated females with white eyes with males with red eyes. The resulting F1 females had red eyes, BUT males had white eyes! Doesn't seem to agree with Mendel. iv. There seemed to be some linkage between gender and eye color. B. N. Stevens worked with Tenebrio beetles. i. Stevens discovered 20 large chromosomes in diploid cells of female beetles, but 19 large and one small chromosome in males. ii. This one small one paired with a large that was called X during meiosis I and the two acted like homologs. She called it the Y chromosome. iii. The two together are called sex chromosomes. iv. She also determined the combination of the two determines an individual's gender: XX = female and XY = male. C. From Stevens' work, Morgan concluded some traits are transmitted by X-linked inheritance patterns because the gene is located on the X chromosome. The Y chromosome does not carry alleles for most genes occurring on the X chromosome, so the trait coded for by the allele on the X chromosome is observed in the phenotype. D. Inheritance of genes located on non-sex chromosomes is called autosomal inheritance. Inheritance of genes located on the sex chromosomes is called sex- linked inheritance. E. Case study question: What determines sex: the X or Y chromosome? Does the presence of 2 X's cause an individual to be a biological female, or does the presence of a Y make a biological male? F. Other sex-linked traits : Male patterned baldness, color-blindness, and hemophilia G. What about non-humans? H. Multiple allelism. The occurrence of more than two alleles for a locus in a population, even though only one occurs on each chromosome (2 on homologs in diploid cells). In Mendel's world two alleles creates 3 genotypes and two phenotypes only. i. In most populations, dozens of alleles can be identified for each gene. ii. It just so happened that Mendel worked with 7 genes of a species (peas), where there were only 2 alleles per gene in the population. And where one was completely dominant over the other. iii. Human Blood Types : Has 3 alleles at one locus (remember only 2 alleles per locus at a time can occur in a cell. iv. Beta-globin gene in humans for making hemoglobin has over 500 different alleles that affect loads of variations having to do with living at various altitudes, different temperatures and resisting diseases. I. Linkage and Crossing over. i. Morgan, working with fruit flies, wanted to know whether alleles of 2 traits could be located on the same chromosome and thus be assorted dependently. And did they always stay linked. In other words, does Mendel's Law of Independent Assortment hold true if alleles occur on the same chromosome? J. Other issues with Mendel's results: i. Incomplete dominance : Where a heterozygous genotype expresses an intermediate phenotype. Kind of like blending. ii. Codominance : Both genotypes are expressed, not as a dilution of one (like incomplete) but as a combination of the two. See blood types earlier. iii. Polygenic effects : a single trait is affected by many genes with the effects being additive. Leads to continuous variation rather than an either/or situation. iv. Pleiotropic effects : A single allele affects many traits. v. Environmental effects : It's important to distinguish how much of a trait is due to environmental pressures vs. how much is strictly genetic. vi. Epigenetic effects : Environmental cues can cause certain genes to be turned on and off. These traits may last for generations, and then be switched back for generations. This gives some credence to Lamarck's acquired characteristics