Lecture 07 - BIOT 732 Genetic Engineering II Lecture Notes PDF
Document Details
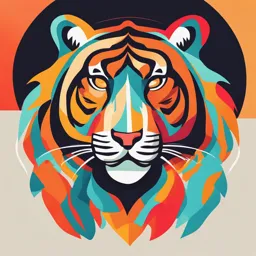
Uploaded by InvulnerableRomanArt8992
German University in Cairo
Dr. Ulrike Breitinger
Tags
Summary
Lecture 07 of BIOT 732 Genetic Engineering II, presented by Dr. Ulrike Breitinger, focuses on various cloning techniques and nuclease-mediated DNA editing methods.
Full Transcript
Lecture 07 - BIOT 732 Genetic Engineering II Dr. Ulrike Breitinger Faculty for Pharmacy and Biotechnology Cloning Techniques Nucl...
Lecture 07 - BIOT 732 Genetic Engineering II Dr. Ulrike Breitinger Faculty for Pharmacy and Biotechnology Cloning Techniques Nuclease-mediated DNA editing techniques 1 Learning outcome Describe and distinguish between the following cloning techniques: Revision: - Overlap Extension PCR - QuikChange Mutagenesis - Topo Cloning New Techniques: Gibson Assembly® Cloning Gateway Cloning Experiment Golden Gate Assembly Nuclease-mediated DNA editing techniques ZFN TALEN 2 Revision: Cloning techniques Overlap Extension PCR QuikChange Mutagenesis Topo Cloning 3 Revision Overlap Extension PCR (Cell Biology) Step 1: PCR 1A flanking primer A mutagenesis primer A PCR 1B mutagenesis primer B flanking primer B 5’ ATG TGA 3’ 3’ 5’ Standard Conditions (25-30 cycles) Result: PCR product PCR 1A 5’ ATG A Mut 3’ 3’ 5’ TGA 3’ X Y PCR 1B 5’ 3’ Mut 5’ B 1450 nts Step 2: Template = PCR products 1A +1B Standard Conditions (25-30 cycles) 5' ATG TGA 3' 3' 5' 4 Revision Overlap extension PCR Application: Introduction of Mutations What do you need? Gene of interest in own vector, standard enzymes (polymerase, ligase, alkaline phosphatase, restriction enzymes) Advantages: No sophisticated techniques, several steps available in every lab doing genetic engineering No extra cost for kits Disadvantages: Long procedure with many steps (2-3 days) 5 Revision QuikChange Mutagenesis (Cell Biology) Step 1 Mutant strand synthesis (normal PCR reaction) Perform thermal cycling to: - denature DNA template - anneal mutagenic primers containing desired mutation - extend and incorporate primers with PfuUltra DNA polymerase Step 2 DpnI digestion of template (digests parental methylated and hemimethylated DNA) Step 3 Transformation Transform mutated molecule into competent cells for nick repair DNA-hemimethylation: https://www.nature.com/articles/nmeth0507-455 only one of two complementary strands is methylated 6 Revision QuikChange Mutagenesis (Cell Biology) Application: Introduction of a Mutation What do you need? Gene of interest in own vector Enzyme: DpnI Advantages: Less steps than overlap extension method → quicker No sophisticated techniques No extra cost for kits Disadvantages: Difficult and very inefficient PCR reaction Random mutation inside vector inserted during PCR remains unnoticed 7 Revision Topo Cloning (GEI) Background: Topoisomerases can change the state of supercoiling of the DNA. In order to decrease gene activity, DNA topoisomerases introduce temporary single-strand breaks (type I) or double-strand breaks (type II) in the phosphate backbone of the DNA. The mechanism of topoisomerase action includes the transient formation of an ester bond between a tyrosine residue of the enzyme and the DNA molecule. Later on, the breaks are closed by reformation of the original phosphodiester-bond and the enzyme released from the DNA. These enzymes are capable of relaxing supercoiled DNA. Ligase-independent ligation of PCR products The ability of topoisomerase-I to form phosphodiester bonds can be used for ligation of DNA molecules. PCR products generated with Taq DNA polymerase have 3’ overhanging A’s. “Topo Cloning”, Invitrogen; Heyman et al, Genome Res. 9:383-392, 1999. 8 Revision Topo Cloning (GEI) Vector with covalently Taq polymerase leaves a 3’ A linked topoisomerase overhang on PCR products that can be used for cloning Topoisomerase Topoisomerase Step 1: PCR products generated with Taq DNA polymerase have Topoisomerase 3’ overhanging A’s. catalyses the covalent Step2: PCR products are mixed with linearized vector molecules linkage of the vector to the PCR product that have 3’ overhanging T residues which are “activated” by covalently bound topoisomerase I. Topoisomerase I catalyzes the formation of covalent phosphodiester bonds between the ligation partners and is Covalently cloned released from the DNA. vector is ready for Unlike DNA ligase, topoisomerase I requires that the 5’ ends transformation into have a free OH group. bacteria “Topo Cloning”, Invitrogen; Heyman et al, Genome Res. 9:383- 92, 1999. Fig 6-6 Genes and Genomics: A Short Course (3e) 9 Revision Topo Cloning Application: Insertion of PCR products / genes What do you need? Special Topo vector (used up after usage) You cannot use own vector → You need to buy the kit for every usage fresh Advantages: It is easy if you follow the company’s instructions Iess steps than comparable classical technique (no restriction digests or classical ligation needed) insertion of long fragments possible (specific Topo Kit) Disadvantages: much more expensive (prices of vectors for eukaryotic expression > 1000 €) very inflexible Example: I have problems in cloning a long fragment into my vector Solution for Topo cloning: either: Kit special for long fragments available but not for eukaryotic vector or: It is possible to introduce topoisomerase custom-made into own vector (4-6 weeks) However, both specialties in one experiment is not possible 10 New Cloning techniques 1. Gibson Assembly® Cloning 11 Introduction: Gibson Assembly® Cloning Introduced by NEB by Dr. Daniel Gibson Gibson Assembly was developed by Dr. Daniel Gibson and his colleagues at the J. Craig Venter Institute and licensed to NEB by Synthetic Genomics, Inc. It allows for successful assembly of multiple DNA fragments, regardless of fragment length or end compatibility. Gibson Assembly efficiently joins multiple overlapping DNA fragments in a single-tube isothermal reaction. The Gibson Assembly Master Mix includes three different enzymatic activities that perform in a single buffer: The exonuclease creates single-stranded 3´ overhangs that facilitate the annealing of fragments that share complementarity at one end (overlap region). The proprietary DNA polymerase fills in gaps within each annealed fragment. The DNA ligase seals nicks in the assembled DNA. The end result is a double-stranded fully sealed DNA molecule that can serve as template for PCR, or a variety of other molecular biology applications, including direct transformation. The method has been successfully used by Gibson’s group and others to assemble oligonucleotides, DNA with varied overlaps (15–80 bp) and fragments hundreds of kilobases long. 12 Introduction: Gibson Assembly® Cloning Exonuclease activity DNA polymerase activity DNA ligase activity Figure 1: Overview of the Gibson Assembly Cloning Method 13 Overview Gibson Assembly® Cloning Overview of Gibson Assembly Cloning Kit Protocol: Design primers to amplify fragments (and/or vector) with appropriate overlaps. PCR amplify fragments using a high-fidelity DNA polymerase. Prepare linearized vector by PCR amplification using a high-fidelity DNA polymerase or by restriction digestion. Confirm and determine concentration of fragments and linearized vector using agarose gel electrophoresis, a NanoDrop instrument or other method. Add fragments and linearized vector to Gibson Assembly Master Mix and incubate at 50°C for 15 min to 1 hour, depending on number of fragments being assembled. Transform into 5-alpha Competent E. coli or use directly in other applications. 14 Primer Design Gibson Assembly® Cloning Structure of the Overlapping PCR primers for use in Gibson Assembly must have two sequence components: an overlap sequence, required for the assembly of adjacent fragments; a gene-specific sequence, required for template priming during PCR amplification; - The non-priming overlap sequence is added at the 5´-end of the primer (overhang in the PCR reaction) → This sequence is homologous to the 5´-terminal sequence of the adjacent fragment. The length of overlap sequence is dependent on the GC content of the sequences. - The priming gene-specific sequence is added at the 3´-end of the primer. The priming sequence should meet the criteria required for template annealing during PCR amplification. The Tm of the 3´ gene-specific sequence of the primer can be calculated using the Tm calculator found on the NEB website at http://www.neb.com/TmCalculator. 15 Figure 2A: Primer design using in silico created final DNA sequence file. 16 Primer Design Gibson Assembly® Cloning General Recommendations for Design of Overlapping Primers To achieve efficient assembly of PCR fragments into a vector, use a 15–25 nt overlap with a Tm equal to or greater than 48°C (assuming A-T pair = 2°C and G-C pair = 4°C). - First, mark the junctions between the adjacent fragments 1, 2 and 3 (Fig. 2A, step II). - At or near each junction choose 15–25 nts sequences to serve as the overlap region between the two adjacent fragments. - Mark the first 5´ and the last 3´ nts of the overlap sequence on both DNA strands (boxed sequence). - Finally, starting from the first 5´ nt, copy the entire overlap sequence in the 5´ to 3´ direction and continue to add nts to the 3´ end until the gene-specific priming sequence length is reached (Fig. 2A, step IV). - The reverse overlapping primer is designed following the same steps as described above but copying the sequence from the complementary DNA strand in the 5´ to 3´ direction. Keep in mind that the two primers sharing the same overlap sequence are always used in separate PCR reactions, each in combination with the primer which primes the complementary sequence on the opposite end of the respective DNA fragment (Fig. 2A, step V). 17 Figure 2B: Primer design for PCR- generated vector and insert using in silico created final DNA sequence file. In silico PCR is a computer application-based analysis of the PCR reaction with the help of the primer information. Here, the software checks the designed primers against the genome of our interest. It examines the primer set selected against the target sequence or genome. 18 Example pET21 vector The pET21a forward primer (orange arrow) and the reverse primer (blue arrow) start at the position where the lacZ gene must be inserted. Both vector-specific primers completely match the vector sequence on the respective strands. → This inverse PCR strategy yields a linear vector fragment. Generally, 10–100 pg of a vector is recommended as a template in the inverse PCR reaction. - To amplify the lacZ gene, forward and reverse lacZ-specific priming sequences (gray) are fused with the respective vector sequences to be used as overlap sequences in assembly with the vector. - The length of the overlap sequence is determined by the number of nts needed to reach a Tm ≥ 48°C. If necessary, one may add additional nucleotides between the overlap sequence and the lacZ-specific sequence, for example, to introduce a unique restriction site. 19 Gibson Assembly® Cloning – Vector Treatment The vector needs to be linearized: (1) Restriction digest or (2) inverse PCR reaction on vector Vector Digestion with Restriction Enzymes The cloning vector can be linearized by any restriction endonuclease or by any combination of two restriction endonucleases displaying unique site(s) at the desired locations within the vector sequence. Note: Double-digestion of vector DNA with two restriction endonucleases is the best approach to reduce the uncut vector background. Some restriction endonucleases cannot efficiently digest supercoiled DNA and thus may leave behind different amounts of uncut vector DNA. If not gel-purified, the uncut vector is transformable and will show up after transformation of the Gibson Assembly reaction, thereby, reducing the overall fraction of recombinant clones. Restriction endonucleases might have a reduced activity on plasmid DNA purified using various plasmid purification kits. Inverse PCR reaction on vector PCR reaction with 2 primers in opposite direction. PCR product is complete linear vector. Template DNA has to be digested with DpnI 20 Gibson Assembly® Cloning – PCR Insert Recommendations for PCR Amplification of insert When using circular plasmid DNA as a template, it is important to use a minimal amount of DNA (0.1–0.5 ng) to reduce the template background after transformation. If higher template DNA is used, it is recommended to digest the PCR product with DpnI restriction endonuclease. Verify PCR product purity and yield by gel electrophoresis. If non-specific DNA fragments are obtained (complete purity < 90%), gel purification is needed. 21 Gibson Assembly® Protocol 1. Set up the following reaction on ice 2. Incubate samples at 50°C for 15 min (2-3 fragments) or 60 min (4-6 fragments) Following incubation, store samples on ice or at –20°C for subsequent transformation. 3. Transform competent E. coli cells with 2µl of the assembly reaction, following the transformation protocol. 22 Gibson Assembly® Cloning What do you need? Specific primers, having 15-20 nts overhang Gibson Assembly kit with including exonuclease, DNA polymerase and DNA ligase Restriction enzymes to cut vector or DpnI to digest template vector Advantages: You work in your own vector works with long inserts faster than classical cloning using restriction enzymes and ligation up to 6 fragments of DNA can be inserted in one step Disadvantages: Moderate cost for the kit (120 € for kit/ 10 reactions without competent cells) 23 New Cloning techniques 2. Gateway Cloning 24 Revision : Site-specific Recombination GEII Site-specific recombination occurs between DNA molecules that have only short regions of sequence similarity, possibly just a few base pairs. A region of extensive homology is not a prerequisite for recombination: the process can also be initiated between two DNA molecules that have only very short sequences (~20 nts) in common, e.g. during the infection cycle of bacteriophage λ. Integration of λ DNA into the E. coli genome: During the lysogenic phase the λ genome becomes integrated into the E. coli chromosome. Integration occurs by site-specific recombination between the attachment or att sites, attP on the λ genome attB on the E. coli chromosome. att sites = attachment site of ~20 nts The core sequence ‘O’ of the att sites present in bacteriophage λ and in the E. coli chromosome. 25 Background: Gateway Cloning Gateway cloning is a highly efficient alternative to restriction cloning and does not require the use of restriction enzymes. Instead, an insert is moved into a vector through a two-step recombination process that attP: phage takes advantage of integration and excision attB: bacteria reactions using attachment sites attL and attB. It is based on the site-specific recombination machinery used by phage (lambda) to integrate its genome into E coli. attL attR att sites = attachment site of ~20 nts 26 Background: Gateway Cloning The phage lambda, in an attempt to outwit the restriction enzyme defense of bacteria, evolved a lysogenic pathway. In this situation, the phage uses site-specific recombination to integrate itself into the bacterial genome and hide from the restriction enzyme defense mechanism. The enzymes used by phage lambda, to integrate and excise itself, attP: phage attB: bacteria are the basis of Gateway cloning. The integration system involves the specific DNA sequences found on the phage, attP-sites, as well as sites found on the bacterial genomic target, attB. Recombination between the B and P elements results in new recombination sequences flanking the inserted phage DNA. attL attR After insertion, the recombination sequences are now called attL (left) and attR (right). 27 Background: Gateway Cloning If the phage senses that the bacteria is under stress, it will excise itself. Upon excision, attL and attR are converted back to attP and attB, and the phage DNA is removed from the bacterial chromosome. The phage then begins its lytic growth cycle. The Gateway system uses both the integration and excision reactions to create an alternative to restriction enzyme cloning. Since Gateway Cloning is moving elements from one plasmid to another, they do not refer to integration and excision, but rather the BP reaction (BP→ LR) and the LR reaction (LR→ BP). These two recombination events are perpetually reversible. 28 Gateway Cloning Proceeds in two Steps attP1 attP2 attL1 attL2 A ccdB gene is attB1 attB2 a suicide gene and will kill any attB flanked PCR product attR1 attR2 bacteria that BP Clonase hosts it. Entry clones attL1 attL2 attR1 attR2 attB1 attB2 contain a single attP1 attP2 fragment of interest that is located between attL sites LR Clonase Gateway BP Clonase enzyme contains both Int (Integrase) and IHF (Integration Host Factor) proteins that catalyze the in vitro recombination of PCR products or DNA segments from clones (containing attB sites) and a Donor vector (containing attP sites) to generate Entry clones. 29 Designing a Gateway Cloning Experiment Entry Vectors and Donor Vectors are used to capture a gene or gene fragment of interest, to create an Entry Clone. An entry clone is a plasmid carrying a fragment of interest located between attL sites. Conventionally, entry vectors depend on restriction enzyme cloning to introduce your fragment of interest between the attL sites. Gateway cloning: Donor vectors require the use of PCR to add attB sites to the fragment of interest then the use of the Gateway BP Clonase reaction (Step 1 BP reaction) This product is an Entry Clone: The fragment of interest is now located between attL sites, and ready for subsequent Gateway Reactions (Step 2 LR reaction). Positive and Negative Selection: The Donor Vector pDONR201 contains multiple genes for selection including: A Kanamycin resistance gene which will positively select for the presence of the plasmid A ccdB gene, which is a suicide gene and will kill any bacteria that hosts it Following the BP reaction all plasmids transformed will be able to survive Kanamycin selection, but only recombinants, which have lost the ccdB gene will be able to grow. 30 Cloning Options Choice of three molecular approaches to create your Entry Clones. 1. Restriction enzyme cloning into an Entry Vector, such as pENTR1A. Two small multicloning sites are located inside each attL site allowing standard restriction cloning reactions to be carried out. 2. BP Clonase reaction into a Donor Vector. Several DONR vectors exist which allow you to create an entry clone using BP clonase. To do this you need to create PCR primers with tails on them which add the required attB sites to your fragment of interest. 3. TOPO cloning into a pENTR plasmid. Several Gateway vectors allow direct capture of a PCR product by TOPO cloning. All vectors allow you to quickly create an entry clone, which includes attL1 and attL2, often using primers you already have on hand. 31 Creating Expression Clones Entry Clones are transferred to Destination Vectors using the Gateway LR Clonase reaction to create an Expression Clone. There are numerous Destination Vectors pre-engineered for specific experimental applications. All of these destination vectors include attR1 and attR2 and are therefore compatible with standard Gateway Entry clones, whether they are made by restriction enzyme cloning, BP clonase, or TOPO cloning. 32 Multisite Gateway cloning Multiple fragments can be assembled into a single destination vector. Technique utilizes site-specificity of four different att sites to recombine multiple fragments into a single construct in one step. First step starts with the BP reaction. Multiple DNA fragments are created containing different pairs of flanking attB sites. Each Entry Clone is built and sequenced separately. These entry clones are used in the LR reaction. Corresponding att sites on the Entry Clones and the Destination Vector must align correctly. The compatible flanking sequences are then recombined and incorporated into the desired destination vector to create the final expression clone containing the multiple DNA fragments of interest. 33 Gateway Cloning: Top 5 Tips 1. Buy it ☺ 2. Verify that proteins are in-frame Gateway Cloning is frequently used when building clones for expression in vivo or in vitro. These clones will often include a protein tag for subcellular targeting, visualization or purification. Double-check start and stop codons, and the correct reading frame is maintained in your designed construct. 3. Pay attention to quantities ENTRY CLONES: pDONR to PCR insert 1 to 1 molar ratio (~50 femtoM each) EXPRESSION CLONES: Single fragment Gateway: Expression vector / Entry Clone: 1 / 1 molar ratio (~20 femtoM) Multi Fragment Gateway: Expression vector 20 femtoM; each Entry Clone 10 femtoM 4. Pay attention to time BP clonase is recommended to incubate for 1 hour. If you are getting poor yields, consider extending the time. LR clonase can take longer, particularly if you are performing multisite Gateway cloning. 5. Combine BP and LR reactions When cloning a PCR product for Gateway expression you can save time and money if you skip the transformation and isolation steps after your BP clonase reaction to create your Entry Clone. 1. Combine the attB modified PCR product with pDONR and your chosen expression vector. 2. Incorporate both BP clonase and LR clonase 34 Pros & Cons of Gateway Cloning Pros Large libraries of cloned and sequenced gene fragments are available for purchase. Gateway cloning is high throughput. The same LR reaction can be applied to a single clone of interest, to easily transfer it to pre-configured destination vectors that allow you to ask common experimental questions. Gateway cloning allows you to re-use your sequence validated fragment of interest in multiple experiments, without additional sequence verification. Multisite Gateway technology allows you to quickly test different gene fragments in different combinations Cons: There are a couple of major disadvantages to using Gateway Cloning Large scars (25-bp unwanted junk sequence of att site) are left in your molecular constructs. For simple applications of single fragment Gateway cloning, the scars have little impact. In multisite Gateway constructs, the scars can have potential undesired effects on experimental outcomes. Gateway cloning has high barriers to entry. Gateway cloning can be more difficult to master than other techniques. In addition, you may need to make several Gateway compatible entry clones until you can fully take advantage of the system. High costs: 616.00 $/ 12reactions AFTER CLONING: If you are observing a significant proportion of background colonies after LR reactions, consider shortening the length of your post-transformation incubation. The escape from negative selection is powerful. If the ccdB gene acquires a mutation, you will observe the growth of clones that do not carry insert. 35 Gibson Cloning versus Gateway Cloning Although Gateway cloning has been widely used in many experimental systems, the following are the four main disadvantages with this method: (i) the associated two-step (BP and LR recombination reactions) cloning process is labor-intensive and time-consuming (ii) the recombination site leaves a 25-bp unwanted junk sequence (scar) (iii) the assembly of multiple fragments is relatively inefficient (iv) the commercial enzyme mixes available for this method are expensive, especially for laboratories in developing countries. As an alternative, Gibson assembly is a quick and easy method that enables the linking of multiple overlapping DNA fragments in a single isothermal reaction (Gibson et al., 2009). 36 New Cloning techniques 3. Golden Gate Assembly 37 Golden Gate Assembly - Introduction What is Golden Gate Assembly? Golden Gate assembly, also known as Golden Gate cloning, is a one-pot, one-step cloning procedure created by Carola Engler and colleagues in 2008. The method takes advantage of Type IIS restriction enzymes (e.g. BsaI), which cleave DNA outside their recognition sequences. The result is an ordered assembly of a vector and one or more DNA fragments. How Does Golden Gate Assembly Work? Golden Gate assembly can be split into two distinct steps that occur within the same reaction: (1) Type IIS restriction enzyme digestion (2) DNA ligation 38 Golden Gate Assembly – Restriction Enzyme Digestion Step 1: Type IIS Restriction Enzyme Digestion The destination vector and insert fragment(s) both contain compatible Type IIS restriction sites. Golden gate single insert cloning 39 Golden Gate Assembly - Restriction Enzyme Digestion Unlike conventional restriction cloning, which utilizes Type IIP restriction enzymes that recognize a palindromic sequence and cleaves within the recognition site, Golden Gate assembly uses Type IIS restriction enzymes. Step 1: Digest with Type IIS restriction enzymes Type IIS restriction enzymes have various unique properties that make Golden Gate assembly possible. Non-palindromic recognition site: The recognition site is non-palindromic. In general, sites range from 4 to 7 nucleotides. Shifted cleavage: Cleavage is performed outside the recognition site. The shifted cleavage site allows for sequences to be digested for cloning without disruption of important sequences. Variable sticky ends: Cleavage on each strand is staggered, resulting in unique overhangs (1 to 5 nucleotides) associated with a single recognition site. 40 Golden Gate Assembly - Restriction Enzyme Digestion For example, with a 4-base overhang, up to 256 different overhang sequences are possible, which enables multiple DNA fragments to be assembled in the same reaction. The unique four base pair overhangs are often referred to as Fusion Sites. Type IIP v. Type IIS restriction enzymes Type IIS restriction enzymes exhibit great diversity in the length of their recognition site, the offset of the cleavage site from the recognition site, and the nature of the overhang. 41 Golden Gate Assembly - Ligation Step 2: DNA Ligation Once the destination vector and DNA insert(s) are digested, their complementary overhangs are joined together by DNA ligase to create an ordered assembly. The process is often denoted as “scarless” or “seamless” since undesired nucleotides are not added between the DNA fragments and the restriction sites are eliminated in the final construct. Multi-insert Golden Gate Cloning 42 Golden Gate Assembly - Reaction Components of Golden Gate Assembly Gateway Reactions are processed in a thermocycler. Golden Gate assembly works by mixing the following components into a single reaction tube: Destination vector DNA insert(s) (e.g. amplicon or pre-cloned) Type IIS restriction enzyme (e.g. BsaI) T4 DNA ligase Reaction buffer Assemblies of more than 3 fragments will cycle through reaction temperatures to achieve optimal cloning outcomes. 43 Golden Gate Assembly - Vector Destination Vector Golden Gate destination vectors are available either through commercial sites (e.g. New England Biolabs), individual labs, or Addgene. Alternatively, you can adapt your own vector to be Golden Gate ready. The vector should contain two Type IIS recognition sites (e.g. BsaI) that flank the desired insert region and a screening marker (e.g. lacZ). The enzyme recognition sites must be oriented with an “outward” orientation so that after digestion the vector insert and the enzyme’s recognition sites are removed from the vector. It is critical to remove undesired Type IIS cleavage sites from your destination vector; this is known as domestication. Unwanted cleavage sites can be easily removed with site- directed mutagenesis. Golden gate destination vector 44 Golden Gate Assembly - Insert DNA Insert(s) Any double-stranded piece of DNA can be used as the source of insert for Golden Gate Assembly, including both PCR product and plasmid DNA. A fragment of interest is adapted for Golden Gate Assembly by the addition of Type IIS restriction sites. Recognition sites must be oriented with an “inward orientation” so that after cleavage the Type IIS recognition site is removed from the fragment. Golden gate DNA insert For amplicon preparation, PCR primers are designed that contain flanking bases, the Type IIS recognition site and an overhang sequence. After amplification, the PCR product can either be cloned into a Golden Gate Destination vector or into a Golden Gate Entry Vector, such as pGGA. 45 Golden Gate Assembly – PCR Example Forward Primer: 5’ tt GGTCTC a GGAG attcacacccaaaacattc Reverse Primer: 5’ tt GGTCTC g ATGG atcaactgaattgaaaagag As with the destination vector preparation, it is also important that the DNA insert(s) do not contain Type IIS restriction sites. Unwanted cleavage sites can be removed with site-directed mutagenesis. If the Type IIS recognition site is in a coding sequence, the mutation(s) you introduce must be silent. 46 Golden Gate Assembly-Based Kits There are various pre-designed kits available that utilize Golden Gate technology, such as transcription activator- like effector nucleases (TALENs), Golden GATEway and modular cloning (MoClo). TALENs Thomas Cermak and colleagues first described the design and assembly of custom TALEN constructs in 2011. TALENs are fusions of transcription activator-like (TAL) effectors to a Type IIS restriction endonuclease (e.g. FokI), which is achieved through Golden Gate assembly. TAL effectors contain a customizable array of amino acid repeats that bind to desired regions of DNA. Once bound to DNA, the fused nuclease creates a targeted double-strand break. An alternative to CRISPR, TALENs are useful genome engineering tools that can be used to create gene knockouts or introduce specific DNA sequence modifications. 47 Nuclease-mediated DNA editing techniques 1. Zinc-Finger Nucleases (ZFN) 2. Transcription Activator-Like Effector Nucleases (TALENs) 48 Zinc-Finger Nucleases (ZFN) Introduction: Zinc-finger nucleases (ZFNs) are artificial endonucleases targeting DNA that have been adopted as gene-targeting tools Zink finger proteins were first identified in a study of transcription in the African clawed frog, Xenopus laevis in the laboratory of Aaron Klug. 1985. ZFNs were first described by Chandrasegaran and his team in 1996. These sequence-specific chimeric proteins containing DNA binding domain are fused to a nonspecific cleavage domain (derived from type II restriction enzyme FOKI) ZFN-induced double-strand breaks are subject to cellular DNA repair processes that lead to both - targeted mutagenesis and - targeted gene replacement at remarkably high frequencies. Zinc Finger Nucleases (ZFNs) were the first widely used programmable DNA binding protein system. 49 Background: Zink - Finger Proteins - Revision The classical C2H2 zinc finger typically contains a repeated 28– 30 AA sequence, including two conserved cysteines and two conserved histidine residues. However, other combinations of Cys/His as the zinc‐chelating residues are possible. About half of all human TFs are from the C2H2 zinc finger class. Each of these TFs uses an array of zinc finger (ZF) domains to bind to DNA. The full-length sequence preference of a TF is determined by the collection of the sequence preferences of its DNA-binding ZFs. 50 ZIF268 recognizes its binding site using three Cys2His2 zinc fingers (a) Overall arrangement of Zif268 bound to DNA. Each finger inserts its α helix into the major groove, and DNA recognition is mediated by amino acids at positions −1, 2, 3 and 6 from this helix, with the majority of contacts made to one strand of the DNA (primary strand). Interactions that are mediated by hydrogen bonds between the fingers and DNA are indicated by arrows in the panel on the right; hydrophobic interactions are indicated by open circles. (b) Amino acid sequence of the three Cys2His2 zinc fingers. Avocado: Adjacent fingers in this structure are connected by ‘canonical’ linkers. Blue: residues from each finger involved in defining DNA specificity Purple: residues involved in zinc coordination The secondary structure elements are indicated above the sequence: arrows = β strands and cylinder = α helices. Wolfe, 2000: Combining structure-based design with phage display to create new Cys2His2 zinc finger dimers 51 Zink - Finger Nucleases A ZFN is an artificial Each zinc finger nuclease recognizes triple DNA code endonuclease that consists of a designed zinc finger protein (ZFP) fused to the cleavage domain of the FokI restriction enzyme. For genome engineering, a ZFN is targeted to cleave a chosen genomic sequence which provokes cellular repair processes that in turn mediate efficient modification of the targeted locus. The carboxyl terminal region of Sp1 containing 3-zinc finger region as DNA binding domain can also serve as nuclear localization signal (NLS). 2010 Urnov et al: Genome editing with engineered zinc finger nucleases 52 Zink - Finger Nucleases If the ZFN-induced cleavage event is resolved via non- homologous end joining, this can result in small deletions or insertions, effectively leading to gene knockout. Approach has been used to establish efficient reverse genetics in Drosophila melanogaster, zebrafish, rats, Arabidopsis thaliana and mammalian somatic cells. In reverse genetics, the starting point is the gene. A ‘reverse geneticist’ aims to specifically modify a gene, or its expression, and 2010 Urnov et al: Genome editing with characterize the phenotypic consequences of this modification. engineered zinc finger nucleases 53 TALEN Transcription Activator-Like Effector Nucleases 54 Transcription Activator-Like Effector Nucleases (TALENs) Transcription Activator-Like Effector Nucleases (TALENs) are artificial restriction enzymes that have the ability to cut DNA at the point of contact with a series of nucleotides. TALENs involve the synthesis of the C-terminal with type II restriction enzyme, Fokl, in order to produce heterodimers that form a double-stranded break (DSB) in DNA. Recent advancements in TALEN include nonspecific DNA-splitting nuclease combined with a DNA binding domain that can be easily designed to facilitate TALENs for effective targeting of various gene sequences (2010). 55 Transcription Activator-Like Effector Nucleases (TALENs) 2010 (a) Schematic diagram of a TALEN. TALE repeats are shown as colored cylinders with a final carboxyl-terminal truncated “half” repeat. Letters inside each repeat represent the two hypervariable residues. TALE-derived N and C-domains are required for DNA-binding activity. The nuclease domain from the FokI endonuclease is non-specific. (b) TALENs bind and cleave as dimers on a target DNA site. Cleavage by the FokI domains occurs in the “spacer” sequence that lies between the two regions of the DNA bound by the two TALEN monomers. (c) The amino acid sequence of a single TALE repeat is expanded below with the two hypervariable residues highlighted in orange. A single DNA nucleotide can be recognized by the amino acids at the 12th and 13th position. 34-AA repeat sequence (d) TALE-derived DNA-binding domain aligned with its target DNA sequence. Note the matching of repeat domains to single bases in the target site according to the TALE code. Also note the presence of a 5’ thymine preceding the first base bound by a TALE repeat. 56 2013 Joung et al: TALENs: a widely applicable technology for targeted genome editing TALEN – Origin TALEs proteins were first reported in 2009, derived from phytopathogenic bacterial genus Xanthomonas. TALE is a special class of proteins that can bind DNA. General schematic of the TALE polypeptide chain - The N-terminal region (NTR) contains the type III translocation system - the central repeat domain (CRD) contains the conserved 34 amino-acid repeats for sequence- specific DNA-binding 34-AA repeat sequences (4 AAs per helix turn) - C-terminal region contains nuclear localization each recognizing one DNA base signals - and an acidic activation domain. Co-crystal structure of the PthXo1 TALE bound to its specific DNA target, with only the CRD region and a portion of the NTR shown for display. 57 Transcription Activator-Like Effector Nucleases (TALENs) 2010 https://www.cell.com/trends/cell-biology/fulltext/S0962-8924%2813%2900057-3 58 Transcription Activator-Like Effector Nucleases (TALENs) 2010 (A) TALEs contain nuclear localization signals (NLS) and an activation domain (AD) to function as transcriptional activators. A central repeat domain confers specific DNA-binding and host specificity. Each 34 amino acid (aa) long repeat in the CRD binds to one nucleotide with specificity determined mainly by aa at position 13. One sample repeat is shown below the protein scheme. Numbers 12/13 refer to aa positions within the repeat. (B) Structure of an individual TALE repeat module. The repeat has 34 amino acids in length and takes a loop– helix secondary structure where two α-helices are linked by short ‘RVD loop’. The residue 13 is responsible for preferential binding of the repeat module to a single specific nucleotide in the major groove of target DNA sequence (C, in this case). (C) Repeat types have specificity for one or several nucleotides. Only bases of the DNA leading strand are https://www.ncbi.nlm.nih.gov/pmc/articles/PMC4168661/ shown. 59