Essentials Of Anesthesiology PDF
Document Details
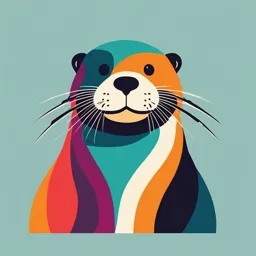
Uploaded by ElatedEquation
NYU
David C. Chung, Arthur M. Lam
Tags
Summary
This book, Essentials of Anesthesiology, is a textbook covering the basic principles of surgical anesthesia. It discusses various anesthetic drugs, techniques, and equipment. Detailed information on airway management and postoperative pain management is also included.
Full Transcript
- -..... hird Edition ESSENTIALS OF DAVID C. CHUNG /ARTHUR M. LAM ¥ Digitized by the Internet Archive in 2012 http://www.archive.org/details/essentialsofanesOOchun AMKTURini nnv HIiLO...
- -..... hird Edition ESSENTIALS OF DAVID C. CHUNG /ARTHUR M. LAM ¥ Digitized by the Internet Archive in 2012 http://www.archive.org/details/essentialsofanesOOchun AMKTURini nnv HIiLO ilLOlULUu I Third Edition ESSENTIALS OF MNto ntOlULUl] I i DAVID C. CHUNG, M.D. Associate Professor (Clinical) Department of Anesthesia University of British Columbia Vancouver, British Columbia, Canada ARTHUR M. LAM, M.D. Professor Department of Anesthesiology University of Washington Seattle, Washington SAUNDERS An Imprint of Elsevier SAUNDERS An Imprint of Elsevier The Curtis Center Independence Square West Philadelphia, Pennsylvania 19106 Library of Congress Cataloging-in-Publication Data Chung, David C. Essentials of anesthesiology / David C. Chung, Arthur M. Lam- 3rd ed. p. cm. Includes bibliographical references. ISBN 0-7216-6675-2 1. Anesthesiology — Handbooks, manuals, etc. I. Lam, Arthur M. II. Title. [DNLM: 1. Anesthesia. 2. Anesthetics. WO 200 C559e 1997] RD82.2.C48 1997 617.9'6 dc20— DNLM/DLC 96-22873 ESSENTIALS OF ANESTHESIOLOGY ISBN 0-7216-6675-2 Copyright © 1997, 1990, 1983 by W. B. Saunders Company All rights reserved. No part of this publication may be reproduced or transmitted in any form or by any means, electronic or mechanical, including photocopy, recording, or any information storage and retrieval system, without permission in writing from the publisher. Permissions may be sought directly from Elsevier's Health Sciences Rights Department in Philadelphia, USA: phone: (+1)215-238-7869, fax: (+1)215-238-2239, email: [email protected]. You may also complete your request on-line via the Elsevier Science homepage (http://www.elsevier.com), by selecting 'Customer Support' and then 'Obtaining Permissions'. Printed in the United States of America. Last digit is the print number: 9 8 7 6 Preface to the Third Edition In this edition, we heeded the advice of our colleagues to consolidate Essentials as an introductory textbook on sur- gical anesthesia. To fulfill this goal, we have de-emphasized chapters dedicated to subspecialty areas and expanded on knowledge dealing with basic principles and practice. Two new chapters have been created to reflect current interest: one on the management of postoperative pain and the other, anesthesia for outpatient surgery. In addition, the chapter that was dedicated only in previous to tracheal intubation editions has been replaced by one on airway management, in which management of the difficult airway and use of the laryngeal mask and emergency airways are discussed in de- tail. Similarly, we have improved all the chapters on the pharmacology of anesthetic drugs, included detailed check- out procedures in chapters dealing with anesthestic equip- ment, and added a section on assessment of the airway in the chapter on assessment of the patient. No effort was spared and all chapters have been revised. Students of an- esthesiology should be able to move on to specialty text- books after reading Essentials and also keep it as a pocket companion in the wards and operating rooms. Once again we would thank our students and col- like to leagues for sharing their ideas with us. We are also indebted to Andrea Aikens, Vicky Earle, and Dale Northey for con- tributing to the new illustrations in this edition; to Intavent Research Ltd., Henley-on-Thames, U.K., Vitaid, Toronto, On- tario, Canada, and Gensia Inc., San Diego, California, U.S.A. for providing information on the Laryngeal Mask Airway; to Cook (Canada) Inc., Stouffville, Ontario, Canada for helping with the illustration of the cricothyrotomy airway; to An- vi Preface to the Third Edition aesthesia, Anesthesia & Analgesia, Anesthesiology, British Journal of Anaesthesia and Clinical Pharmacology and Therapeutics for permission to reprint copyrighted material; to Berta Steiner of Bermedica Production, Ltd. for editorial assistance; and to Joan Sinclair of W. B. Saunders Company for supervising the production of this edition. David C. Chung, M.D. Arthur M. Lam, M.D. Preface to the Second Edition When preparing this new edition, we were troubled with what should reasonably be included in an introductory text- book of anesthesiology. After all, the specialty has made pro- gress in many areas: monitoring, new drugs, management of surgical pain, definition of standards of safe practice, quality assurance, and so forth. In the end we have included all these topics and revised others, but have increased the length of the text only slightly. We believe this edition re- tains the basic conciseness and comprehensiveness of the first edition. We trust it will continue to serve as a compan- ion to our students; and again we welcome the opinion of our readers. David C. Chung Arthur M. Lam vn Preface to the First Edition Anesthesia is a recognized subject in the curriculum of most medical teaching facilities, and many monographs have been published on the subject. However, we have had dif- ficulty in recommending a basic textbook for our students. Indeed, there is more than one good introductory anesthe- siology textbook on the market, but they are directed more to students at an intermediate level rather than to those be- ing introduced to the specialty for the first time. The idea of writing this book was conceived to fill this need, and our students and colleagues urged us on. Anesthetic procedures can be learned only in the operating room. The goal of this book is to provide the scientific basis of anesthesia, not to replace practical experience. In twenty- four chapters, anesthesia-related problems in the patient undergoing surgery are defined and the principles of safe anesthetic practice are discussed. Every attempt to be com- prehensive and concise has been made so that the reader can easily acquire a firm foundation in anesthesiology. The student is encouraged to regard this book as his com- — panion to bring it with him to the ward and into the op- erating room; to read it; and to refer to it. Anesthesiology is still a growing specialty. Many of our col- leagues are practicing only in areas of special interest in the operating room — for example, in cardiovascular and tho- racic surgery or neurosurgery; others are active in the emer- gency room, the intensive care unit, and the pain clinic. We have chosen to limit the scope of this book to the principles of surgical anesthesia and resuscitation. Other than the an- esthetic management of obstetric, pediatric, geriatric, and ambulatory patients, areas of subspecialty are omitted by in- ix x Preface to the First Edition tention; we student should not be expected to feel that the be involved in these areas in an introductory course. Although this book is written for clinical clerks, it should be a useful primer for all students of anesthesia. It should also be instructive for physicians, dental surgeons, nurses, and respiratory technicians who are involved in the care of the surgical patient. We trust this book will serve the needs of many, and we welcome the opinion of our readers. David C. Chung Arthur M. Lam Contents i General Anesthesia — Basic Principles 1 2 Intravenous Anesthetics 11 3 Inhalation Anesthetics 28 4 Opioids 45 5 Muscle Relaxants 59 6 Local Anesthetics 79 7 The Anesthetic Machine and Accessories 92 8 Anesthetic Circuits 109 9 Mechanical Ventilators 119 10 Effect of Anesthesia on Respiratory Function 127 xi xii Contents 11 Anesthesia and Systemic Illness 137 12 Preoperative Assessment and Preparation of the Patient 153 13 Airway Management in Anesthetized Patients 162 14 Monitoring Principles and Practice 188 15 Techniques of General Anesthesia 214 16 Management of Complications during Anesthesia 223 17 Techniques of Local and Regional Anesthesia 237 18 Care of the Patient during Recovery 252 19 Management of Acute Postoperative Pain 262 20 Special Considerations in Surgical Outpatients 281 21 Fluid and Electrolyte Requirements of Surgical Patients 290 Contents xiii 22 Blood Transfusion in Surgical Patients 299 23 Anesthetic Mishaps, Quality Assurance, and Risk Management 314 APPENDIX I Further Reading 321 Index 327 General Anesthesia — Basic Principles The noun anesthesia was coined by Oliver Wendell Holmes to describe the temporary and reversible state of "unawareness" induced by drugs to render surgery painless. Before the discov- ery of anesthetic drugs, surgery was largely limited to the ex- cision of lesions on the body wall, the amputation of digits and limbs, and the extraction of teeth. Through the centuries, many methods have been tried to relieve the agony of surgery including hypnosis, ingestion of alcohol or herbal concoc- tions, application of pressure to nerve trunks, and local — hypothermia but none was effective or reliable. In 1823, Henry Hill Hickman, a physician-scientist from Shropshire, England, set out on a quest to find an inhaled an- esthetic. Carbon dioxide attracted his attention because of its anesthetic-like properties at high concentrations. Unfortu- nately, carbon dioxide is not an anesthetic, and Hickman died young in 1830 with his dream unfulfilled. After him no others made deliberate attempts to find an anesthetic until some 20 years later, when American physicians and dentists discovered almost simultaneously the anesthetic properties of nitrous ox- ide and ether. The discovery of nitrous oxide anesthesia came on December 10, 1844, when Horace Wells, a dentist from Hartford, Con- necticut, attended an exhibition by Gardner Q. Colton, who pre- pared nitrous oxide and administered the gas to another audi- ence member named Samuel A. Cooley. While he was intoxicated, Cooley accidently injured his leg but did not cry out in pain. Realizing the significance of what he observed, Wells decided to try out the effectiveness of this agent in ob- tunding the pain of dental surgery. The following day he ar- ranged to have one of his own teeth extracted by an assistant while Colton gave him nitrous oxide. He felt no pain! Embold- ened by this experience, Wells began to use nitrous oxide in his practice. In January 1845, he arranged a public demonstra- tion of his technique at the Harvard Medical School in Boston, but the event turned into a fiasco and was declared a failure. 2 General Anesthesia — Basic Principles Although Wells and other dentists continued to use nitrous ox- ide in their practice, took another 2 decades before nitrous it oxide regained its rightful place as an effective anesthetic. Whereas the discovery of nitrous oxide anesthesia was well recorded, the discovery of ether anesthesia is shrouded. There is evidence to show that William E. Clarke of Rochester, New York, gave his first and only ether anesthetic to a young woman for dental extraction as early as January 1842, but no personal record of this event was made. Crawford W. Long, a physician practicing in Jefferson, Georgia, gave the first recorded ether anesthetic on March 30, 1842. Subsequently he continued to administer ether anesthesia occasionally in his own practice, but he did not report his experience until 1849, when ether anesthesia was already being practiced worldwide. It was Wil- liam T. G. Morton, a dental colleague of Wells, who successfully carried out a public demonstration of ether anesthesia on Oc- tober 16, 1846, at the Massachusetts General Hospital in Bos- ton. Within weeks of this event, ether anesthesia was practiced across the United States and Canada and met with equal en- thusiasm in Great Britain, continental Europe, Australia, and South Africa. Arguably William T. G. Morton is regarded by some to be the forefather of anesthesia. The inscription on his tombstone reads: Inventor and Revealer of Inhalation Anesthesia; Before Whom, in All Times, Surgery was Agony; By Whom, Pain in Surgery was Averted and Annulled; Since Whom, Science has Control of Pain. In the ensuing years the art and science of anesthesia devel- oped rapidly. In 1847, James Simpson, a Scottish obstetrician, introduced chloroform as an alternative to ether; and in 1863, Gardner Q. Colton re-established nitrous oxide as an adjunct in anesthetic practice. During these early years of inhalation an- esthesia, morphine quickly established itself as a preanesthetic medication and as an intraoperative supplement. It is not surprising that the first anesthetics discovered were all given by inhalation. After all, the respiratory and the gastro- intestinal tract were the only routes routinely used in the ad- ministration of medicinals. The parenteral route awaited the arrival of the hollow hypodermic needle and the syringe, which were not invented until the 1850s. The dawn of intravenous anesthesia came in 1873, when Pierrre-Cyprien Ore of Bor- deaux, France, published his experience with intravenous chlo- ral. Subsequent clinical trials were carried out also with hexo- barbital (a short-acting oxybarbiturate), but the technique was not firmly established until 1935, when J. S. Lundy of the Mayo Clinic demonstrated the safe use of thiopental (an ultra-short- acting thiobarbiturate), still a popular induction agent in use today. Although the muscle relaxant curare had been used to General Anesthesia — Basic Principles treat spastic disorders for a number of years, it was not until 1942 that Griffith and Johnson of Montreal reported its use in surgical anesthesia. Thus, the discovery of all four groups of anesthetic drugs used in modern practice (i.e., the intravenous anesthetics, in- haled agents, narcotic analgesics, and muscle relaxants) spanned 100 years. Since then, new members in each group have been introduced to meet specific needs. At the same time, the science and practice of anesthesia has developed into a well-recognized medical specialty called anesthesiology. The anesthesiologist is no longer just an averter and annuler of sur- gical pain. The anesthesiologist works hand in hand with sur- geon and other medical colleagues in both evaluation and prep- aration of the patient before surgery, is the patient's primary care physician during the intraoperative period, and has direct input in the postoperative management of the patient. Using expertise gained in the operating room, the anesthesiologist has also become a significant contributor in other areas of health care, such as the intensive care unit and pain clinic. By training and by action, the anesthesiologist has earned his or her posi- tion as a medical consultant. In English-speaking countries other than the United States, the anesthesiologist is commonly called an "anesthetist." This term, although perhaps confusing to American students, has an entirely reasonable basis: One who administers an anesthetic is an anesthetist. However, in the United States, only half the an- esthetics given annually are administered by anesthesiologists (physician anesthetists); the other half are given by nurse anes- thetists. Whereas the anesthesiologist is a graduate physician who has had 4 or more years of postgraduate training in the management of surgical patients both inside and outside the operating suite, of critically ill patients in intensive care and trauma units, and of patients with acute or chronic pain syn- dromes, the certified registered nurse anesthetist (CRNA) is a professional who has had 2 or 3 years of postgraduate training in surgical anesthesia after basic nursing education and acquir- ing work experience in critical care. In many hospitals and clin- ics, nurse anesthetists are integral members of the surgical team. Molecular Mechanisms of Anesthetic Action Anesthesia is a state of reversible loss of awareness and reflex reactions to noxious stimuli. A large number of agents, ranging from inert gases and volatile liquids to water-soluble and -in- soluble organic compounds, can induce the general anesthetic General Anesthesia — Basic Principles state. The diversity of molecular species that can produce this state and the lack of a structure-activity relationship have elim- inated a molecular mechanism of action that is common to all. Yet this diversity does not rule out a common target in their mode of action. Action on Nerve Cell Membrane Ion Channels In recent years a growing body of evidence has indicated that anesthetic drugs exert their effect through interference with the function of ion channels in nerve cell membrane. Whereas the nerve cell membrane is composed of a bimolecular layer of phospholipids, the ion channels (or ionophores) are formed by globular protein subunits (typically five such subunits) pene- trating the full thickness of the membrane. These protein sub- units have been designated a, (3, 7, or 8 according to their amino acid composition. Ion fluxes through these channels are re- sponsible for impulse generation (neuronal excitation) and im- pulse transmission (axonal conduction). Three types of ion channels have been identified: 1. Ligand-gated (ligand-activated) channels have binding sites on the channel protein subunits. When molecules with agonist activity [the ligand) bind to these sites, the channel pro- teins undergo conformational changes and the channels open to allow the passage of specific ions. These ionophores have binding sites for drugs that can modulate the activities of the natural ligand. These modulator sites are adjacent to and dis- tinct from receptor sites for the physiologic ligand. Examples of physiologic ligands are acetylcholine, gamma-aminobutyric acid (GAB A), L-glutamate, and serotonin. 2. Voltage-gated (voltage-activated) channels can sense changes in the electrical potential across the nerve cell mem- brane. Changes in this potential change the conformation of the globular channel proteins and open the channels to the flux of specific ions. Some voltage-gated ionophores also have receptor sites for drugs that can modulate the behavior of the channel. 3. Metabotropic receptor-gated channels are indirectly ac- tivated by ligands. The binding of agonist molecules to receptor sites sends a signal that is transduced via a guanosine triphosphate— binding protein (G protein) to the channel pro- tein subunits to open the channel. In addition to G protein, intracellular second messengers may be involved also in signal transduction. Intravenous Anesthetics at GABA A -Gated Channels Many intravenous anesthetics depress central nervous sys- tem activityby enhancing inhibitory synaptic transmission. General Anesthesia — Basic Principles n a < < ? o CD m n LL H N < < h- a UJ CD (9 0) CL 00000 ooooo ooooo 000(30 FIGURE 1-1. Illustration of a GABA A -gated chloride channel in nerve cell membrane. Whereas the nerve cell membrane is a bimolec- ular layer of phospholipids, the ion channel is a pentameric structure made up of five protein subunits penetrating the full thickness of the membrane. The channel protein subunits contain binding sites for the natural ligand gamma-aminobutyric acid (GABA) as well as modulator sites for benzodiazepines (BZDP), thiopental (STP), etomidate (ETMD), and propofol (PPF). These modulator sites are adjacent to and distinct from the GABA sites and are unique for each drug. Occupation of GABA sites by the natural ligand causes conformation changes in the protein subunits, and the channel opens to allow negatively charged chloride ions to enter the neuron, leading to hyperpolarization of the nerve cell membrane and rendering the neuron less excitable. Occu- pation of the modulator sites by the respective drug molecules en- hances the action of GABA. The main inhibitory neurotransmitter in the central nervous system is gamma-aminobutyric acid. Two subtypes of GABA receptors have been identified Subtype A (GABA A ) receptors are found on postsynaptic neuronal membranes. Occupation of GABA A binding sites by the physiologic agonist GABA activates chloride channels (GABA-gated chloride channels). Negatively charged chloride ions entering the neuron through opened channels cause hyperpolarization of the nerve membrane (the resting membrane potential becomes more negatively charged), rendering the neuron less excitable. Benzodiazepines, thiopental, etomidate, and propofol can oc- cupy binding sites adjacent to and distinct from GABA A sites on the postsynaptic nerve cell channel protein subunits and enhance the inhibitory effect of GABA on postsynaptic neu- rons. Whereas the benzodiazepines achieve this positive mod- ulation on GABA by increasing the frequency of opening of the chloride channels, thiopental achieves its effects by increasing the opening time of these channels. The binding sites on the postsynaptic nerve cell membrane are different among agents (Fig. 1-1). General Anesthesia — Basic Principles Ketamine at NMDA-Gated Channels Unlike the other intravenous anesthetics, ketamine depresses central nervous system activity by suppressing excitatory syn- aptic transmission mediated by L-glutamate. Binding of L-glu- tamate to channel protein sites on the postsynaptic nerve cell membrane activates calcium channels. The flux of positively charged calcium ions into the cell through these opened chan- nels causes depolarization of the membrane potential and fa- cilitates neuronal excitation. A subtype of glutamate receptors binds the agonist N-methyl-D-asparate (NMD A) selectively, and the ionophores associated with this subtype of receptors are called NMDA receptor channels. (NMDA is used to classify these glutamate-gated channels only; the natural ligand at these channels is L-glutamate.) Ketamine, a dissociative anesthetic (see ''Ketamine" in Chapter 2), is a noncompetitive antagonist of L-glutamate at NMDA receptor sites and inhibits the post- synaptic excitatory action of L-glutamate. Ketamine binding sites are adjacent to and distinct from NMDA receptor sites. Action of Inhalation Anesthetics There is also convincing evidence that inhalation anesthetics depress central nervous system excitability through modulation of normal ion channel function. However, their site of action is less clear. At the turn of the century, Meyer and Overton ob- served a direct relationship between potency of inhalation an- esthetics and their solubility in oil. More recently, it has been confirmed that the minimum alveolar concentration required to maintain anesthesia is lower for more lipid-soluble inhalation agents and vice versa (see "Minimum Alveolar Concentration" in Chapter 3). The Meyer-Overton rule implies that inhalation anesthetics act on the lipid environ of the brain, and nerve cell membranes of phospholipids have been suggested as their site of action. However, the lipid solubility of inhalation anesthetics does not rule out the action of these molecules on amphophilic pockets of channel protein subunits. Lipid Solubility Hypothesis There is more than one version of the hypothesis relating lipid solubility to action. The volume expansion hypothesis postulates that anesthetic drug molecules taken into the lipid matrix of nerve cell membrane cause expansion of these sites and increase lateral pressure on the protein units of ionic chan- nels that penetrate the membrane. When a critical volume of expansion is reached, ionic flux through these channels is ob- structed and neuronal excitability inhibited. This hypothesis is General Anesthesia — Basic Principles supported by the observation that exposure to high hydrostatic pressure, perhaps by restricting the expansion of the lipid ma- trix, can partially antagonize the effect of inhalation anesthetics in some animal species. The membrane fluidization hypothesis, in contrast, proposes that the protein units forming the ion channels are embedded in a bilayer of phospholipid molecules arranged in an orderly way in the gel phase. The presence of anesthetic drugs in- creases the motility and disrupts the orderly arrangement of these lipid molecules — that is, a transition from the gel to the fluid phase occurs. As a result, the protein molecules forming Conformation Change Action Potential Inhalation \{ Anesthetic ((§)) No* Partial Conformation Change -* No Action Potential FIGURE 1-2. Illustration of lateral phase separation, a version of the lipid solubility hypothesis of the action of gaseous anesthetics. A, A closed ion channel in the axonal membrane is surrounded proximally by phospholipids in the fluid phase and farther laterally by those in the gel phase. B, During excitation, the channel springs open for pas- sage of ions, a process facilitated by transforming some of the lipids from the high-volume fluid phase to the low-volume gel phase. C, In the presence of anesthetic molecules, some of the outlying gel-phase lipids become more fluid. D, These fluid-phase lipids fail to condense into the low-volume gel phase, so there will not be room for the ion channel to open on receiving an excitatory impulse. (From Trudell JR:A unitary theory of anesthesia based on lateral phase separations in nerve membrane. Anesthesiology 46:5, 1977; reprinted with permission.) General Anesthesia — Basic Principles the ion channels lose their structural support and function. In a more elaborate version of the fluidization hypothesis, it is said that protein molecules of closed ion channels in nerve cell membrane are surrounded proximally by phospholipids in the fluid phase and further laterally by those in the gel phase (Fig. 1 — 2 A). During excitation, these channel proteins undergo con- formational changes to allow the passage of ions, a process fa- cilitated by transforming some of the lipids from the high-vol- ume fluid phase to the low-volume gel phase (Fig. 1-2B). In the presence of anesthetic molecules, some of the outlying gel- phase lipids become more fluid (Fig. 1 — 2C). Furthermore, these fluid-phase lipids fail to condense into the low-volume gel phase to make room for the conformation changes to take place in the channel protein units (Fig. 1 — 2D). Consequently these channels become obstructed and neuronal excitation is inhibited. Protein Interaction Hypothesis The most convincing evidence that fat-soluble inhalation an- esthetic molecules can bind proteins come from studies in fire- flies. In the lanterns of fireflies, the enzyme luciferase binds the substrate luciferin to emit light. Inhalation anesthetics, binding to luciferase, inhibit this light-producing reaction. The protein interaction hypothesis postulates that inhalation anesthetic molecules, acting directly on amphophilic sites of channel proteins, modulate the gating mechanism of ion chan- nels. For example, it has been shown that inhalation anesthet- ics can increase the opening time of GABA A -gated chloride channels and enhance GABA-mediated inhibitory synaptic transmission — an action similar to that of thiopental described above. Inhalation anesthetics have been shown also to reduce the opening time of NMD A channels and inhibit L-glutamate- mediated excitatory synaptic transmission, an action similar to that of ketamine. This direct interaction with channel proteins implies a more direct and specific action of inhalation anes- thetics on ion fluxes, whereas the lipid solubility hypothesis suggests a less direct mode of action. Stages of Ether Anesthesia Whereas knowledge of the mode of action of anesthetic drugs continues to expand, the clinical effects of these drugs have been described in detail since their discovery. Guedel described the progressive depression of the central nervous system by ether in four stages: analgesia, excitement, surgical anesthesia, and impending death. Although ether is no longer used in mod- General Anesthesia — Basic Principles 9 em anesthesia practice, many of the signs of anesthesia de- scribed by Guedel are still in popular usage to describe the anesthetic state. Analgesia (Stage I). The stage of analgesia lasts from onset of drowsiness to loss of eyelash reflex (blinking in response to stroking of the eyelash). Excitement (Stage II). The stage of excitement is character- ized by agitation and delirium. Respiration is irregular, and co- pious salivation can occur. Pupils are large and the eyes are divergent. Toward the end of this stage, respiration is again rhythmic (automatic respiration). Surgical Anesthesia (Stage III). This stage is subdivided into four planes: Plane 1 Respiration is rhyth- Plane 3 Lasts from onset of mic and rapid eye paresis of intercostal movement from side muscles to paralysis to side is seen of these muscles Plane 2 Lasts from cessation Plane 4 Lasts from paralysis of rapid eye move- of intercostal mus- ment to onset of cles to paralysis of paresis of intercostal the diaphragm; the muscles patient ceases to breathe at the end of this plane Impending Death (Stage IV). The stage of impending death lasts from onset of apnea to failure of the circulation and rep- resents medullary depression. Components of General Anesthesia When ether is replaced by modern volatile inhalation agents, the stages of anesthesia described for ether remain more or less intact, although progression from consciousness to the surgical planes is rapid and landmarks between stages are less obvious. Concurrent use of intravenous anesthetics, opioids, and muscle relaxants, in contrast, can obscure all the stages and signs of anesthesia observed with inhalation anesthetics. Rees and Gray therefore described the state of general anesthesia in terms of three basic components — the triad of unconsciousness (hyp- nosis), analgesia (areflexia), and muscle relaxation. Unconscious (Hypnosis). With development of uncon- sciousness, the patient is oblivious of all sensation, but somatic and autonomic reflexes to pain and noxious stimuli can still occur. Analgesia (Areflexia). Withdrawal of a limb or flight (so- matic reflexes) and hypertension, tachycardia, and sweating 10 General Anesthesia — Basic Principles (autonomatic reflexes) are part of the subconscious reaction to pain. These potent reflexes must be subdued during anesthesia. Muscle Relaxation. The degree of muscle relaxation re- quired varies according to the operation. In general, only a mild degree of muscle relaxation is necessary for superficial opera- tions on the body wall or extremities, but moderate to profound muscle relaxation is required for operations within body cavities. Anesthetic Drugs In the past, the triad of general anesthesia was obtained by progressive depression of the central nervous system with ether or chloroform alone. In current practice, a multiplicity of agents with specific actions are used to provide unconsciousness, an- algesia, and muscle relaxation. These include intravenous an- esthetics, inhalation anesthetics, narcotic analgesics, and mus- cle relaxants. The pharmacology of these agents is discussed in the next four chapters. Intravenous 2 Anesthetics The intravenous route is a popular method for administration of drugs used in anesthetic practice. Injection of a drug directly into the circulation allows rapid distribution to the site of action and quick onset of pharmacologic effects. By giving the drug in small increments, dose can be titrated against observed effects. Once the desired actions are achieved, they can be maintained by repeated bolus injections or continuous infusion. The intravenous route is not without drawbacks. Once the drug is injected, its effect cannot be reversed readily and there is potential for catastrophe. Side effects can be unexpectedly se- vere as a result of the high, although transient, plasma concen- tration attained following rapid intravenous injection, and the danger of anaphylaxis is ever present. In addition, phlebitis and thrombophlebitis are relatively common problems. Unless sterile technique is followed, the intravenous route can become the gateway for bacteria, pyrogens, and other foreign bodies to enter the circulation. Last but not least, there is the risk of fluid over- load and air embolism. Pharmacokinetics When plasma concentration of an intravenous anesthetic (e.g., thiopental) plotted on a logarithmic scale against time is after it is given intravenously as a bolus, the curve thus ob- tained has three distinct features (Fig. 2-1). An initial peak is followed by a phase of rapid decline in plasma level (the dis- tribution phase) and a later phase of slower decline (the elim- ination phase). Peak Concentration Following a bolus injection, plasma concentration peaks within one or two circulations of the drug in the body. The 11 12 Intravenous Anesthetics 200 E o o o < CO < _l Q_ INJECTION TIME AFTER INJECTION (mm) FIGURE 2 — 1. The rise and fall in plasma concentration of an intra- venous anesthetic following injection of a bolus. The concentration rises to a peak following injection, then falls at a rapid rate in the distribution or a-phase, when the drug is distributed to and taken up by tissues. During the elimination or 3-phase, when the drug is being metabolized and/or excreted, the rate at which the plasma concentra- tion falls is considerably more gradual. drug-laden blood circulates most rapidly to the vessel-rich group of organs, where the drug is taken up instantaneously (see "Tissue Blood Flow and Tissue Mass" below). This period of peak plasma concentration is only transient. As tissue uptake progresses, plasma concentration falls. Distribution The phase of relatively rapid decline in plasma level initial is known as the distribution phase [a. phase). During this pe- riod, decline in plasma level is almost solely the result of dis- tribution and redistribution of drug to and uptake of drug by various organs. The pharmacologic effect of intravenous anes- thetics is determined by drug concentration in brain tissue, which is largely related to blood level. Therefore, the rate at which the plasma level falls determines the duration of action after a single bolus injection of intravenous anesthetic. The rate of this decline (i.e., the slope of the distribution phase) is de- termined by tissue uptake. Several factors act independently to influence tissue uptake and therefore this decline: protein bind- ing, physical characteristics of the drug, and tissue blood flow and tissue mass. Intravenous Anesthetics 13 Protein Binding In the circulation, all intravenous anesthetics are bound to plasma protein (usually albumin), but the degree of binding varies according to the agent used. Because only free, non-pro- tein-bound drugs can diffuse across cell membranes, protein binding decreases tissue uptake and drug action at tissue sites. These binding sites are nonspecific, so many foreign com- pounds can compete for them. In the presence of such a sub- stance or in the event of a decreased plasma concentration of albumin (e.g., in liver disease), more of the drug will be present in the unbound form after intravenous injection of a usual dose. Because unbound drugs are pharmacologically active, this higher concentration of free drug can be the cause of an unex- pected overdose. Physical Characteristics of the Drug Some physical characteristics of the drug itself are major de- terminants of how rapidly it is taken up by tissues. These in- clude lipid solubility, molecular size, and the state of ionization. Of these, lipid solubility is the most important. Highly lipid- soluble drugs (e.g., all intravenous anesthetics) are taken up rapidly by tissues. Lipid-soluble drugs also pass readily into "special circulations" (e.g., brain and placenta). With water-soluble agents, molecular size is an important de- terminant of diffusibility across plasma membranes. The smaller the molecule, the more easily it can cross into tissue. With highly lipid-soluble agents, molecular weight plays little role in influencing diffusibility. For drugs that are ionizable, the state of ionization greatly affects how rapidly they enter tissues. Only nonionized mole- cules diffuse across tissue barriers readily. As equilibrium ex- ists across plasma membranes only for the nonionized form of a drug, so the total drug concentration (the ionized plus the nonionized fractions) on either side of the membrane can be very different. The pK a of a drug is the pH at which 50% of the drug is ionized. The degree of ionization is determined by this value and the ambient pH, according to the Henderson-Hassel- balch equation: [acid form of drug] [base form of drug] This equation can be rearranged for convenience as follows: [acid form of drug] _ _ [base form of drug] There is more acid form than base form of the drug when the difference between pK a and pH is positive; there is more base 14 Intravenous Anesthetics form when the difference is negative. A drug is an acid if it is a donor of protons; it is a base if it is an acceptor of protons. Depending on the particular drug, the ionized fraction can be either a base or an acid. For example, the ionized fraction of sodium thiopental (NaTP) is a base. It can accept a proton ac- cording to the formulas: NaTP ^ Na + + TP" (sodium salt) (ionized base) TP" + H+ ^ HTP (ionized base) (proton) (nonionized acid) Conversely, the ionized fraction of lidocaine hydrochloride (XHC1) is an acid. It can donate a proton according to the formulas XHC1 - XH + + cr (hydrochloride salt) (ionized acid) XH + ^ X + H+ (ionized acid) (nonionized base) (proton) Ion trapping is a phenomenon related to this pH-dependent ionization of many molecules when there is a pH difference across tissue barriers. It is the cause of increase in urinary ex- cretion of salicylate with alkalinization of urine. Sodium salic- ylate (NaS) can exist as an ionized base or a nonionized acid, according to the formulas NaS ^ Na + + S" (sodium salt) (ionized base) S" + H+ - HS (ionized base) (proton) (nonionized acid) After the nonionized acid has crossed into the lumen of renal tubules, a highly alkaline urine (i.e., urine abundant in hy- droxyl ions) favors the equilibrium heavily to the right of the equation HS + OPT ^ S" + H 2 (nonionized acid) (hydroxyl ion) (ionized base) Therefore, most of the salicylate will exist as negatively charged ions "trapped" in the tubular lumen. They cannot be reabsor- bed from the urine and are excreted. Tissue Blood Flow and Tissue Mass All intravenous anesthetics are highly soluble in lipid; they are taken up readily by most tissues. The major factors influ- encing tissue uptake are tissue blood flow and tissue mass. The tissues of the body can be divided into four groups ac- cording to their regional blood flow. Approximately 70% of the cardiac output goes to vessel-rich viscera (brain, heart, liver, kidneys), 25% to lean body mass (muscle), 4% to fat, and only 1% to the vessel-poor group (skin, cartilage, bone). Following Intravenous Anesthetics 15 bolus injection, a drug is first distributed to tissues receiving the greatest portion of cardiac output. Distribution to the vessel- poor group usually can be ignored. Distribution of thiopental among the plasma pool and other tissues after a single injection is illustrated in Figure 2 — 2. As the fraction in the plasma falls, it is first taken up by the brain and other visceral organs, which account for only 10% of body weight. The fraction taken up by this vessel-rich group of tis- sues peaks at 30 seconds to 1 minute after injection. As time goes on, the fraction taken up by skeletal muscle, which ac- counts for approximately 50% of total body weight and has a relatively good blood supply, becomes more significant. This fraction surpasses that in the viscera by 5 minutes and peaks at approximately 20 minutes, while the fraction accumulated in brain and viscera falls. It is this redistribution of thiopental from brain to lean body mass that accounts for emergence from anesthesia after a single injection of this agent. Fat accounts for nearly 20% of body weight and has a high affinity for fat-sol- uble agents, but its blood supply is poor. For this reason, the fraction of thiopental taken up by fat plays no role in the emer- gence from thiopental anesthesia after an induction dose. This physiologic pharmacokinetic model of thiopental uptake and 100 r- i/ie '/a '/4 '/2l 2 4 8 16 32 64 128 TIME AFTER INJECTION (mm) FIGURE 2-2. The distribution of thiopental among the plasma pool, viscera, muscle, and fat following an intravenous bolus. As the fraction of the dose in the plasma pool falls, the fraction taken up by viscera (brain, heart, liver, kidneys) peaks while that taken up by muscle con- tinues to increase. Subsequently the fraction taken up by viscera falls and that taken up by muscle peaks. That is, there is first a distribution of the injected drug to viscera and then a redistribution of the drug from viscera to muscle. The fraction of the dose taken up by fat rises only slowly. (From Price HL, Kovnat PJ, Safer JN et al: The uptake of thiopental by body tissues and its relation to the duration of narcosis. Clin Pharmacol Ther 1:16, 1960; reprinted by permission.) 16 Intravenous Anesthetics distribution also applies to the other intravenous anesthetic drugs. Elimination The phase of slower decline in plasma level represented by the latter portion of the graph in Figure 2-1 is the elimination phase (|3 phase). It is the result of elimination clearance of the intravenous anesthetic from the body by metabolism and biliary and renal excretion. Elimination starts immediately after a bo- lus injection is given for induction of anesthesia, but the rapid decline in plasma level and emergence from anesthesia that follow are due to redistribution of drug to lean body mass. Elimination plays no role in emergence from anesthesia after a single induction dose. However, elimination is important in other clinical settings: 1. After regaining consciousness following a single induc- tion dose of an intravenous anesthetic, the residual sedative effect seen is due to a low level of drug in plasma that is in equilibrium with lean body tissue. The termination of this re- sidual effect is quicker for drugs with a faster rate of elimina- tion and slower for drugs with a slower rate of elimination. 2. After a large dose of an intravenous anesthetic drug has been given by multiple dosing or continuous infusion, the role played by elimination in terminating the anesthetic effect in- creases. If the dose is large enough to saturate the lean body depot, then elimination becomes the sole determinant of recov- ery from anesthesia. In these situations, time to awakening is shorter for drugs with a faster rate of elimination and vice versa. Note: The emergence phase of anesthesia begins with discontin- uation of anesthetic drugs and ends with awakening of the pa- tient. The recovery phase begins with regaining of consciousness and ends with recovery from the residual effects of anesthetic drugs. Hepatic Clearance With few exceptions, intravenous anesthetics are cleared (re- moved) by the liver via biotransformation and biliary excretion. The capacity of the liver to extract a drug from the combined hepatic arterial and portal venous inflow can be expressed as a ratio known as the hepatic extraction ratio (HER) for that drug: Hepatic arterial & portal Hepatic venous ~" venous drug concentration drug concentration Hepatic arterial & portal venous drug concentration Intravenous Anesthetics 17 The product of this ratio and hepatic blood flow determines the rate of hepatic clearance of that drug according to the formula Hepatic extraction ratio X hepatic blood flow = hepatic clearance The maximum value of the hepatic extraction ratio is 1. Hepatic clearance is drugs with high extraction ratios and faster for slower for those with small extraction ratios. Intravenous anesthetic drugs have hepatic extraction ratios ranging from 0.15 to 1. Because of their fast rate of hepatic elimination, intravenous anesthetics with high hepatic extrac- tion ratios cause less residual sedation after a single induction dose and may be used for continuous infusion to maintain an- esthesia. Drugs with a low hepatic extraction ratio can cause prolonged residual sedation even after a single bolus dose and can cause delayed emergence from anesthesia when used as a continuous infusion. Renal Clearance The kidneys are not a major depot for drug metabolism. Instead, renal clearance of drugs involves (1) the passive glomerular filtra- tion of the non-protein-bound fraction and (2) an energy-depen- dent, carrier-mediated secretion of both the bound and unbound fractions by cells in the proximal tubules. After excretion, drugs may be reabsorbed in the distal tubules to a varying degree. This tubular reabsorption is dependent on the same physical charac- teristics discussed earlier: lipid solubility enhances reabsorption and delays excretion, whereas ionization impedes reabsorption and enhances excretion. Occasionally, specific transport mecha- nisms are also involved in reabsorption. With few exceptions, only a minute fraction of the given dose of lipid-soluble intrave- nous anesthetic drugs is excreted unchanged by the kidneys, al- though their water-soluble metabolites are. Specific Agents Intravenous anesthetics have a rapid onset and short duration of action. They are commonly given in a bolus for induction of anesthesia. Agents that are eliminated rapidly are sometimes given repeatedly or infused slowly to maintain anesthesia. Barbiturates Thiopental Thiopental, a derivative of barbituric acid, is an ultra-short- acting barbiturate. It has been in use for induction of general 18 Intravenous Anesthetics anesthesia for 6 decades. Although its popularity is being chal- lenged by propofol, it remains a commonly used induction agent in modern anesthetic practice. Its anesthetic action is ob- vious within one arm-to-brain circulation time. Induction of anesthesia with thiopental is pleasant. The induction dose is 3-5 mg/kg, which should be given slowly over 30-60 seconds so that the dose can be titrated against observed effects. Thiopental has a pK a of 7.6. Almost 60% of the molecules are in the nonionized form at normal body pH. In the presence of acidemia, even more becomes nonionized. This drug is also highly soluble in lipids, and approximately 70% of an injected dose is bound to plasma albumin. Decrease in plasma albumin concentration (e.g., in hepatic disease) or presence of sub- stances competing for binding sites on these protein molecules (e.g., acetylsalicylic acid) may result in unexpectedly severe ef- fects following the administration of a usual clinical dose. The duration of anesthetic action following an induction dose is approximately 5 minutes. This ultra-short action is a result of rapid redistribution of the drug from brain to muscle. It is broken down in the liver by oxidative metabolism to a carboxylic acid derivative via the cytochrome P450 system. The hepatic extraction ratio of thiopental is only 0.15, and less than 1% of an injected dose is excreted unchanged in urine. This slow rate of elimination is responsible for the residual sedative effect seen with thiopental during recovery. It also makes thio- pental unsuitable for maintaining anesthesia by repeated dos- ing or continuous infusion. (Continuous infusion of thiopental is used clinically for induction of barbiturate coma and cerebral protection but not for maintenance of anesthesia.) Thiopental for injection is prepared as a 2.5% (25-mg/ml) aqueous solution of the sodium salt. It contains 6% anhydrous sodium carbonate as a buffer and has a pH of 10.8. Whereas extravascular injection of such an alkaline solution can pro- duce tissue necrosis, intra-arterial injection will cause severe arterial spasm that may result in ischemic necrosis of tissues supplied by the artery. Therefore, sodium thiopental should be given only via a secure and freely running intravenous line. The effect of thiopental on the central nervous system is com- plex. It generally depresses cerebral cortical function and is an effective anticonvulsant. By itself, thiopental has no analgesic properties. In fact, it is said to be antianalgesic because it ap- pears to increase the subjective feeling of pain at subanesthetic doses. Following induction of anesthesia, there is a fall in ce- rebral metabolic rate for oxygen accompanied by a similar fall in cerebral blood flow. Intracranial pressure also declines. On this basis it has been suggested that thiopental may protect the brain from hypoxic or ischemic insults. The principle forms the basis for the induction of barbiturate coma in patients with ce- rebrovascular accidents and head injuries. Recent evidence sug- gests that it is not useful in global hypoxia or ischemia but can Intravenous Anesthetics 19 offer protection when given before the occurrence of focal is- chemic events. Thiopental depresses the vasomotor center of the brain stem, depresses the myocardium, and encourages peripheral vascular pooling, all of which can combine to produce hypotension. It must be given with extreme caution to patients with hypovo- lemia or myocardial disease. The action of thiopental on the respiratory center at the brain stem is biphasic. Following a normal induction dose, it is not unusual to observe a big yawn or a brief period of hyperventi- lation (two to three large breaths) followed by transient apnea that can last for 30 seconds or more. Therefore, thiopental should not be given in the absence of resuscitative equipment and is contraindicated when a patient's airway or ventilation cannot be guaranteed following induction of anesthesia. In ad- dition, thiopental can increase airway irritability, leading to lar- yngospasm or bronchospasm. This is seen soon after induction of anesthesia and is associated with premature instrumentation in the airway (e.g., insertion of an oropharyngeal airway im- mediately following loss of consciousness), which should be avoided. Injection of thiopental into a vein can produce wheals and flares along the course of the vein as well as a characteristic mottled erythematous flush over the upper chest and neck. Both phenomena are related to the release of histamine. An increased incidence of bronchospasm in asthmatics following administration of thiopental is also attributed to this histamine- releasing property. It is absolutely contraindicated in patients acutely ill with asthma, and it is relatively contraindicated in those whose disease is in remission. Like other barbiturates, thiopental can precipitate an acute crisis in patients who have porphyria, and so is contraindicated for them. Other contraindications include hypothyroidism and a history of hypersensitivity to other barbiturates. Thiamylal Like thiopental, thiamylal isalso a thiobarbiturate. These two compounds are comparable in potency and action. Thiamylal injection is a 2% (20-mg/ml) aqueous solution buffered with an- hydrous sodium carbonate. The induction dose is 3-5 mg/kg. Methohexital Methohexital is another ultra-short-acting barbiturate fre- quently used for the induction of anesthesia. Its pharmacologic action is similar to that of thiopental but, unlike thiopental, it is an oxybarbiturate. It is prepared as a 1% (10-mg/ml) aqueous solution with anhydrous sodium carbonate added but not a pre- servative. The induction dose is 1-2 mg/kg. 20 Intravenous Anesthetics Methohexital has a hepatic extraction ratio that varies be- tween 0.5 and 0.85. This faster rate of hepatic clearance imparts to it two major advantages over thiopental: less residual drowsi- ness during recovery and less cumulative effect with repeated injections. However, induction of anesthesia with methohexital is associated with a higher incidence of excitatory phenomena (cough, hiccup, and other myoclonic movements). It is also as- sociated with a transient but unpleasant ache at the site of injection. Alkylphenols Several alkylphenol derivatives have hypnotic properties, but propofol is the only one useful in clinical anesthesia. Propofol Propofol is the newest intravenous anesthetic introduced into clinical practice.The induction dose is 1.5 — 3 mg/kg. Following an intravenous bolus, plasma concentration peaks and then falls off at a much faster rate than thiopental during the distri- bution phase because of extensive tissue uptake. It is metabo- lized primarily to water-soluble glucuronides and sulfates, which are excreted in urine. Less than 1% of a given dose is excreted unchanged by the kidneys. The hepatic extraction ra- tio of propofol is estimated to be 1. Therefore, the rate of he- patic clearance is equal in magnitude to hepatic blood flow of 1500 ml/min (see equation under "Hepatic Clearance" above). However, the rate of total body clearance of propofol is as high as 2200 ml/min, suggesting extensive extrahepatic metabolism. The site of extrahepatic clearance has not yet been identified. Because of its rapid redistribution and elimination, recovery from anesthesia following an induction dose of propofol is more rapid than that following other intravenous agents. This property makes it the most popular agent used in outpatient anesthesia. Its rapid elimination and lack of cumulative effect have also made it ideal for use in a continuous infusion to maintain anesthesia in the so-called total intravenous anesthe- sia (TIVA) technique. Because propofol is water insoluble, it is formulated as a 1% (10-mg/ml) milky-white aqueous emulsion containing 10% soybean oil, 1.2% egg phosphatide, and 2.25% glycerol. De- spite the presence of egg phosphatide in its formulation, it is safe in patients with a history of egg allergy because the aller- gen in these instances is egg albumin. The pharmacologic action of propofol is similar to that of thiopental, but there are differences: Intravenous Anesthetics 21 1. Propofol does not have antianalgesic activity. The inci- dence of nausea and vomiting after propofol is very low. It ac- tually has clinically useful antiemetic properties. 2. Propofol causes a smaller fall in cerebral metabolic rate for oxygen than a comparable dose of thiopental. The latter is a better choice for cerebral protection in focal ischemia. 3. Like thiopental, propofol is an anticonvulsant. Yet cases of convulsion following the administration of propofol have been reported. Myoclonus can occur following induction of an- esthesia using propofol. The incidence of these involuntary movements is more frequent, and the intensity more severe, in children than in adults. 4. An induction dose of propofol causes a larger drop in blood pressure than an equivalent dose of thiopental. This fall in blood pressure is not accompanied by a significant increase in heart rate. It is largely the result of a fall in systemic vascular resistance. Other factors contributing to hypotension include myocardial depression, venous pooling, and impaired barore- ceptor reflex. (When the baroreceptor reflex is impaired, tachy- cardia in response to hypotension is depressed.) 5. An induction dose of propofol causes transient apnea in approximately 30% of inductions. This period of apnea is longer after propofol than after an equipotent dose of thiopen- tal.However, propofol does not increase airway irritability, and instrumentation of the upper airway (e.g., insertion of laryngeal mask) is well tolerated immediately following loss of con- sciousness. Propofol does not cause bronchospasm and is not contraindicated in asthmatic patients. It may even have bron- chodilating properties, but this effect is not as potent as that with ketamine. 6. When propofol is used for induction of anesthesia, some degree of pain occurs at the site of injection in all patients. This can be minimized by avoiding using small veins in the hand or by adding 40 mg of lidocaine to each 20-ml ampule of propofol. 7. Vivid dreams and sexual fantasies during emergence from propofol anesthesia have been reported. These episodes are dif- ferent from the hallucinations and dysphoria seen during emer- gence from ketamine anesthesia. A careful explanation of the situation is usually enough to alleviate anxiety. Benzodiazepines The benzodiazepines are members of a large family of struc- turally related compounds classified pharmacologically as mi- nor tranquilizers. They have found widespread use in anesthe- sia practice as nighttime and preanesthesia sedatives and for sedation during local anesthesia. Two of them, diazepam and 22 Intravenous Anesthetics midazolam, are also used for induction of general anesthesia. Flumazenil, a competitive antagonist, can bind benzodiazepine receptor sites and reverse all the central nervous system effects of agonist compounds. All benzodiazepines have anticonvulsant, anxiolytic, seda- tive, amnesic, hypnotic, and mild muscle relaxant properties. The relaxant effect on skeletal muscles is probably mediated by inhibition of spinal reflexes. Following induction of general an- esthesia with diazepam or midazolam, the cerebral metabolic rate for oxygen falls together with cerebral blood flow. The ben- zodiazepines have been shown in animal studies to protect the brain against ischemic insults, but the degree of protection is less than that by pentobarbital and they are not used clinically for this purpose. Diazepam and midazolam are promoted for induction of anesthesia in cardiac and critically ill patients be- cause of their modest cardiovascular side effects. Nevertheless, hypotension similar in magnitude to that from an equipotent dose of thiopental is not uncommon, particularly when opioids are also used during induction. Like the barbituates, the ben- zodiazepines (particularly midazolam) depress activities of the respiratory centers in the brain stem. The incidence of apnea following equipotent doses of midazolam and thiopental is similar. Diazepam When diazepam is used to induce general anesthesia, the on- gradual (2-3 minutes), and a wide variation in set of action is dose requirement is observed (0.2-0.6 mg/kg). Therefore, the dose should be titrated against the desired effect. Whereas midazolam is considered a short-acting benzodiaz- epine, diazepam is intermediate to long acting. After an intra- venous bolus, the duration of action of both, as with other in- travenous anesthetics, depends on redistribution to lean body mass. This rate of redistribution is slower for diazepam than for midazolam, thus accounting for its longer duration of action. Diazepam is broken down in the liver, yielding two active metabolites: oxazepam and AT-desmethyldiazepam. The hepatic extraction ratio is less than 0.05. Over 70% of its metabolites are excreted in urine, and approximately 10% in feces. Because of the low hepatic extraction and the presence of active metab- olites, the residual sedative effect of diazepam is prolonged during recovery from anesthesia and the effect of repeated or continuous dosing is cumulative. Valium injection is prepared as a 0.5% (5-mg/ml) solution in an organic solvent of mixed composition (40% propylene gly- col and 8% ethyl alcohol together with 1.6% benzyl alcohol as preservative, sodium benzoate and benzoic acid as buffers, and sodium hydroxide to adjust the pH). It becomes cloudy when Intravenous Anesthetics 23 mixed with aqueous intravenous solutions, but there is no ap- parent loss of potency. Pain at the site of injection is common when it is given intravenously, and the incidence of phlebitis is high. Diazemuls is a 0.5% (5-mg/kg) injectable emulsion of diazepam prepared in purified soybean oil, acetylated mono- glycerides, purified egg phospholipids, and glycerol, with so- dium hydroxide added to adjust the pH to 8. This latter prep- aration is less irritating to veins. Midazolam Midazolam is a water-soluble benzodiazepine. The injectable preparation comes either as a 0.1% (1-mg/ml) or a 0.5% (5-mg/ ml) solution with disodium edetate and benzyl alcohol added as preservatives, together with hydrochloric acid or sodium hy- droxide added to adjust the pH to 4 or less. As much as 95% of an injected dose is bound to plasma protein, mainly albumin. It is transformed to hydroxymidazolams in the liver, which are then conjugated and subsequently excreted in urine. The he- patic extraction ratio is 0.5. Compared to the parent compound, the pharmacologic activity of the hydroxyl metabolites is insignificant. Midazolam has all the pharmacologic actions of diazepam and is associated with a higher frequency of anterograde am- nesia. It is two to three times more potent than diazepam, causes little venous irritation, and is more reliably absorbed after intramuscular injection. The induction dose is variable (0.15—0.4 mg/kg) and the onset of action gradual. Although its duration of anesthetic action is less than that of diazepam, it is two to three times as long as that of thiopental. Flumazenil Flumazenil is a benzodiazepine with high affinity for ben- zodiazepine receptors but no agonist activities. It is capable of competitively antagonizing the central nervous system depres- sant effects of agonist benzodiazepines, including their anti- convulsant, anxiolytic, sedative, amnesic, and hypnotic prop- erties. Like other benzodiazepines, it is metabolized in the liver to N-desmethylflumazenil, iV-desmethylflumazenil acid, and flumazenil acid. Subsequently these metabolites are conjugated to form water-soluble glucuronides, which are then excreted in urine. The hepatic extraction ratio is near 1. Flumazenil injection is an aqueous solution containing flu- mazenil 0.1 mg/ml. Acetic acid, disodium edetate, methylpar- aben, and propylparaben are added for preservation, and hy- drochloric acid or sodium hydroxide to adjust the pH to 4. The recommended dose to antagonize the anesthetic effect of diaz- epam or midazolam is 0.2 mg intravenously initially and 0.1 mg every 60 seconds thereafter to a maximum of 1 mg to obtain 24 Intravenous Anesthetics the desired effect. Because the spectrum of central nervous sys- tem depression, from anxiolysis to sedation to hypnosis, by ag- onist benzodiapines increases with the population of receptors occupied, it is possible to titrate the dose of flumazenil to ob- tain the desired degree of reversal. (The population of receptors occupied is directly related to the dose given.) For example, a small dose of flumazenil will decrease the population of recep- tors occupied by the agonist and rouse the patient from uncon- sciousness but spare the anxiolytic effects. Because of its rapid hepatic clearance, the effect of flumazenil is short lived and sedation and hypnosis can recur. In this instance, an intrave- nous infusion of 0.1 — 0.4 mg/hr is useful. Phencyclidines Both phencyclidine and cyclohexamine (a congener) ante- date ketamine as intravenous anesthetic agents, but both pro- duced severe psychotic side effects and were removed from clinical practice. Ketamine Ketamine is a derivative of cyclohexanone. It produces a dis- sociative mental state characterized by catalepsy, sedation, am- nesia, and analgesia. Analgesic effect accompanies subanes- thetic doses, and this property is unique among intravenous anesthetic drugs. Ketamine for injection is prepared either as a 1% (10-mg/ml) or 5% (50-mg/ml) aqueous solution. Both contain 1:10,000 ben- zethonium chloride as a preservative. Approximately 30% of an injected dose is protein bound. It is metabolized by the cy- tochrome P450 system in the liver first to norketamine, which has 20—30% of the potency of the parent compound. Subse- quently norketamine is hydroxylated to hydroxynorketamines, which are then conjugated to form glucuronides. The hepatic extraction ratio is approximately 0.8. More than 90% of the water-soluble glucuronide metabolites are excreted by the kid- neys, and less than 3% in feces. Ketamine can be given intravenously or intramuscularly. The normal dose is 1-2 mg/kg intravenously over 1 minute or 6.5- 13 mg/kg intramuscularly. The duration of action is 5 — 10 minutes after an intravenous dose and 15 — 25 minutes after an intramuscular injection. It has found application in pediatric diagnostic procedures, in burn surgery, and in critically ill patients. In anesthetic doses, ketamine produces a seizure-like electro- encephalogram (EEG) pattern in humans without the associated convulsive muscular activity. It also causes increases in intra- Intravenous Anesthetics 25 cranial pressure, cerebral metabolic rate for oxygen, and cere- bral blood flow. Part of the increase in cerebral blood flow may be attributed to hypercapnia resulting from respiratory depres- sion. Because the increase in cerebral metabolism is matched by the increase in blood flow, cerebral oxygen demand and sup- ply remain in balance in normal subjects. Although the effect of ketamine on intracranial pressure would seem to make it unsuitable for patients who have intracranial hypertension or who are at risk of developing cerebral ischemia, there are studies suggesting that ketamine has cerebral protective effects because it depresses central nervous system activities by sup- pressing excitatory synaptic transmission mediated by L-gluta- mate at NMDA-gated channels. Patients under ketamine anesthesia usually retain the laryn- geal reflex and can maintain a patent upper airway without assistance, although aspiration and airway obstruction some- times occur. These properties make ketamine a safer agent when anesthesia is required under primitive conditions, as at the scene of an accident. The effect of ketamine on the cardio- vascular system is stimulatory; a rise in blood pressure of 20- 40 mm Hg and an increase in heart rate of 30—40 beats per minute are usual. As a result, myocardial work is increased, so ketamine is contraindicated in hypertensive patients and in pa- tients with ischemic heart disease. However, it is useful for in- duction of anesthesia in patients who are hypovolemic when the operation is urgent. Because it is a potent bronchodilator, it is also useful for induction of anesthesia in asthmatic patients. Ketamine is related to the hallucinogens. Emergence from ke- tamine anesthesia is frequently associated with bad dreams and dysphoria. Patients should be allowed to recover undisturbed in a quiet, dark area. The administration of 5-10 mg of diaze- pam intravenously can reduce the incidence of emergence de- lirium without prolonging recovery. Imidazoles Etomidate Etomidate the only imidazole derivative that is used in is clinical anesthesia. It exists both as positive and negative iso- mers, but only the positive form has anesthetic properties. As an intravenous anesthetic, it has an onset and duration of action similar to those of thiopental. It is water insoluble and is for- mulated as a 0.2% (2-mg/ml) solution in a solvent containing 35% propylene glycol. The induction dose is 0.2-0.4 mg/kg. About 75% of an injected dose is protein bound. It is hydro- lyzed in the liver by esterases, and the hepatic extraction ratio 26 Intravenous Anesthetics is 0.9%. Extrahepatic breakdown by plasma esterases is also reported. Approximately 75% of a given dose is recoverable from urine as water-soluble metabolites, and another 15% from feces. Because of its rapid elimination, the residual sedative effect following emergence from anesthesia and the cumulative effect from repeated dosing or continuous infusion are short lived. Like thiopental, etomidate decreases cerebral metabolic rate for oxygen, cerebral blood flow and intracranial pressure; un- like thiopental, it causes only minor respiratory depression, lit- tle change in circulatory function, and no histamine release. Therefore, it can be used safely in hypovolemic, cardiac, and asthmatic patients. However, it is associated with a high inci- dence of pain at the site of injection, myoclonic jerks and hic- cups (a facilitatory phenomenon not accompanied by epilepti- form EEG patterns), and postoperative nausea and vomiting. Because a single dose of etomidate can suppress adrenocortical function for several hours, prolonged infusion to maintain se- dation (e.g., in the intensive care unit) is discouraged, but its use as an induction agent is not contraindicated. Butyrophenones The butyrophenones are major tranquilizers. Whereas halo- peridol is a popular antipsychotic drug, droperidol is used in anesthesia for both sedation and hypnosis. Droperidol Droperidol can produce a neuroleptic state of cognitive dis- sociation.It is also a useful antiemetic that acts directly on the chemoreceptor trigger zone in the medulla. The injectable prep- aration comes as a 0.25% (2.5-mg/ml) aqueous solution with lactic acidadded to adjust the pH to 3.0-3.8. Innovar is an aqueous solution containing droperidol 2.5 mg and fentanyl (an opioid) 50 |jLg/ml. Although droperidol itself has no analgesic properties, it is used in neuroleptanalgesia, a technique in which it is com- bined with a narcotic analgesic (usually fentanyl). This tech- nique has been used to supplement regional anesthesia and to sedate patients during invasive diagnostic procedures. Together with fentanyl, nitrous oxide, and a muscle relaxant, droperidol is also used to induce and maintain neuroleptanesthesia. The neuroleptanalgesic dose in healthy adults is 2.5-5 mg of droperidol together with 50-100 u-g of fentanyl. To induce neuroleptanesthesia, the dose is 15 — 25 mg of droperidol and 300-600 (xg of fentanyl. Intravenous Anesthetics 27 Droperidol has several noteworthy adverse side effects. It has some alpha-blocking properties that can cause hypotension when large doses are given. It can cause an anxious, agitated state (dysphoria) in some patients when it is given alone. Oc- casionally extrapyramidal dyskinesia and parkinsonian rigidity are seen because droperidol interferes with dopaminergic trans- mission in the central nervous system. Inhalation 3 Anesthetics The first anesthetics introduced into clinical practice were inhalation agents (nitrous oxide, ether, chloroform). They were used for both induction and maintenance of anesthesia. Since the introduction of thiopental in 1934, however, the use of in- travenous agents for induction has largely superseded the use of inhalation agents. Nevertheless, induction of anesthesia with inhalation anesthetics is still popular in children and in pa- tients in whom intravenous agents are contraindicated. Unlike that with ether, induction with modern inhalation agents is both pleasant for the patient and rapid in onset. Except for tran- sient agitation and irregularity of breathing, the second stage described for ether anesthesia is seldom obvious. Definition of Commonly Used Terms Administration of a drug via the respiratory tract to act on a distant target organ is unique to the practice of anesthesia. Be- fore reviewing alveolar and tissue uptake of inhalation agents, it is necessary to define the concentration, partial pressure, and minimum alveolar concentration (MAC) of these agents. These terms apply to both gases and vapors. Concentration The fraction of a gas in a mixture is equal to the volume of that gas divided by the total volume of the mixture, and the concentration of this gas in the mixture is this fraction ex- pressed as a percentage. For example, the concentration of ni- trous oxide in a mixture of 7 liters of nitrous oxide and 3 liters of oxygen is 70%; a 10-liter mixture of oxygen, nitrous oxide, and 1% isoflurane has 100 ml of isoflurane vapor (not the liq- uid). Concentration is a convenient way of describing the com- position of an anesthetic mixture. However, concentration gra- 28 Inhalation Anesthetics 29 ISOFLURANE 1 °/ COMPARTMENT A ISOFLURANE GAS MIXTURE ( 7.6 mmHg) COMPARTMENT B -ISOFLURANE BLOOD ; (7.6 mmHg) ADDING ISOFLURANE AT EQUILIBRIUM FIGURE 3-1. The movement of isoflurane across a gas-blood inter- face.At equilibrium, partial pressures of isoflurane in both compart- ments are equal. dient is not the force behind the movement of gas molecules between a gas-liquid or a liquid-liquid interface. Consider the example in Figure 3 — 1, in which a gas mixture in compartment A is separated by a semipermeable membrane from blood in compartment B. If isoflurane is added to com- partment A so that its final concentration is 1%, some of the isoflurane molecules will cross into the blood in compartment B. With time, an equilibrium will be established, and the num- ber of molecules crossing from compartment A into compart- ment B will equal those crossing from compartment B into com- partment A. That is, the net movement of isoflurane molecules is zero. The force behind this movement of isoflurane mole- cules and the eventual state of equilibrium is partial pressure. If compartment B is, in turn, separated by another semiper- meable membrane from a compartment C containing tissue fluid, as in Figure 3 — 2, isoflurane molecules will also cross into compartment C until equilibrium is established. At equilib- rium, the partial pressures of isoflurane in all three compart- ments are equal. Partial Pressure Gas molecules are in constant motion, whether they are in a mixture or in solution. Molecules in a gas mixture are con- stantly bombarding the walls of their container; those in solu- tion are always leaping into the atmosphere above the liquid they are dissolved in or are crossing the boundary of a liquid- 30 Inhalation Anesthetics ISOFLURANE 1% COMPARTMENT A ISOFLURANE GAS MIXTURE ( 7.6 mmHg ) mAd ;ISOFLURANE COMPARTMENT B BLOOD ; ( 7.6 mmHg ) COMPARTMENT C ^ISOFLURANE TISSUE FLUID :i ( 7.6 mmHg ) ADDING ISOFLURANE AT EQUILIBRIUM FIGURE 3-2. The movement of isoflurane across a gas-blood and a blood— tissue fluid interface. At equilibrium, partial pressures in all three compartments are equal. liquid interface. Partial pressure (also called tension) may be regarded as a measure of these activities. By definition, the partial pressure of a component gas in a mixture is equal to the fraction it contributes toward total pres- sure. That is, Partial pressure of _ Total pressure x Concentration of component gas of mixture component gas In the example in Figure 3 — 2, the partial pressure of isoflurane in compartment A is 1% of the atmospheric pressure (760 mm Hg), or 7.6 mm Hg. When a gas is in solution, its partial pressure can be deduced from the composition of the gas mixture with which the solvent is in equilibrium. At steady state, the partial pressure of the gas in both phases is equal. In the example in Figure 3-2, the par- tial pressure of isoflurane in the blood of compartment B is equal to that in compartment A, 7.6 mm Hg. Similarly, the par- tial pressure of isoflurane in the tissue fluid of compartment C is 7.6 mm Hg. It should be noted that this example also de- scribes gas exchange between alveolar gas and pulmonary blood, and between blood and tissue fluid. Inhalation Anesthetics 31 Minimum Alveolar Concentration The MAC of an inhalation agent is the concentration of that agent in alveolar gas necessary to prevent movement in 50% of patients when a standard incision is made. Because the MAC is in effect the median effective dose (ED 50 ) of an inhalation agent, it follows that an alveolar concentration higher than MAC will be required to retain immobility in the remaining 50% of patients when the same standard stimulus is used. Nor- mally immobility can be achieved in 95% of patients when the alveolar concentration of the anesthetic is 30% above its MAC value. Alveolar concentration is a convenient method of quantifying the dose of an inhalation agent a patient receives because it can easily be translated into partial pressure. At equilibrium, the partial pressure of the agent in alveolar gas equals that in blood as well as that in the brain (the site of action). The setting of the vaporizer is not a true reflection of the dose received be- cause some of the anesthetic is lost to the tubing of the anes- thetic circuit or to the surrounding atmosphere before it reaches the respiratory tract. Nor is the inspired concentration, mea- sured at the upper respiratory tract, an accurate reflection of this dose, because a gradient exists between alveolar and in- spired concentrations during the course of a normal anesthetic procedure (see "Alveolar Uptake" below). This dose can be monitored only by measuring end-tidal concentration of the va- por using an anesthetic vapor analyzer (see "Nitrous Oxide and Anesthetic Vapor Monitors" in Chapter 14). The MACs of commonly used inhalation agents are listed in Table 3 — 1. For convenience, the alveolar concentration of an inhalation agent is commonly expressed as a multiple or a frac- tion of its MAC; for example, an alveolar concentration of 2.3% isoflurane is expressed as 2 MACs of isoflurane and an alveolar concentration of 0.85% enflurane as 0.5 MAC of enflurane. In general, the anesthetic effects and the MACs of inhalation TABLE 3-1. Minimum Alveolar Concentration (MAC) of Inhalation Anesthetics AGENTS (IN ORDER OF DECREASING MAC POTENCY) (%) Halothane 0.77 Isoflurane 1.15 Enflurane 1.70 Sevoflurane 2.05 Desflurane 6.0 Nitrous oxide 104.0 32 Inhalation Anesthetics agents used in combination are more or less additive. That is, an alveolar concentration of 70% nitrous oxide (0.7 MAC) and an alveolar concentration of 0.57% enflurane (0.3 MAC) will keep 50% of patients immobile when they are subjected to the standard stimulus. This definition of MAC has made it possible to compare the potency of inhalation anesthetics. An agent that has a lower MAC value is more potent than one with a larger MAC value (see Table 3-1). Similarly, the definition of MAC allows com- parison of side effects of different agents at equipotent anes- thetic doses. There are many factors that will increase or decrease the MAC of an inhalation agent. Pyrexia and the administration of a central nervous system stimulant (e.g., dextroamphetamine, a drug that promotes the release of catecholamine in the central nervous system) increase MAC. Advancing age, hypothermia, and administration of a central nervous system depressant (nar- cotic analgesics, tranquilizers, and barbiturates) all decrease MAC. Other MAC include severe hyper- factors that decrease capnia carbon dioxide tension [P a C0 2 > 90 (arterial Hg), ] mm severe hypoxemia (arterial oxygen tension [P a 2 < 40 Hg), ] mm and severe anemia (hematocrit < 10%). In contrast, mild hy- percapnia, profound hypocapnia, mild hypoxemia, mild ane- mia, circadian rhythms, hyperthyroidism, hypothyroidism, and the duration of anesthesia have no discernible effect on MAC. Alveolar Uptake As pointed out in the previous section, the alveolar concen- tration of an inhalation agent must be raised to a certain level in order to achieve anesthesia. At equilibrium, the partial pres- sure of the anesthetic agent in the alveoli equals that in blood and brain. In practice, the anesthetic is delivered to the upper respiratory tract, from which it is inhaled. The rate of alveolar uptake is gradual and follows an exponential growth pattern illustrated by the curves in Figure 3 — 3. Equilibration between alveolar concentration and inspired concentration occurs only at infinity and is not reached within the course of an anesthetic procedure. The rate of alveolar uptake is determined by three different factors: inspired concentration, washout of alveolar gas, and uptake by pulmonary blood. However, both washout of alveolar gas and uptake by pulmonary blood are influenced by other independent Those that affect alveolar washout are al- factors. veolar ventilation and functional residual capacity, and those that influence uptake by pulmonary blood are solubility of the agent in blood, cardiac output, and alveolar-mixed venous ten- sion gradient. Inhalation Anesthetics 33 HIGH o UJ (J.Z LOW o o cc h—-H INDUCTION TIME (Y) TIME ( mm h «M INDUCTION TIME ( X ] FIGURE 3-3. The influence of inspired concentration on the rate of alveolar uptake of an inhalation anesthetic and on induction time. A, When the inspired concentration is 1%, the alveolar uptake curve fol- lows X; when the inspired concentration is doubled to 2%, the alveolar uptake curve follows Y. The faster rate of rise in alveolar concentration in Y (the slope of the curve) is obvious. B, When inspired concentration is higher, induction time is shorter because of the faster rate of rise in alveolar concentration. Inspired Concentration It is not surprising that the rate of rise in the alveolar con- centration of an anesthetic agent is faster if more of it is deliv- ered in the inspired gas mixture. Curve X in Figure 3 — 3^4 rep- resents the exponential growth in alveolar concentration of a volatile anesthetic when the inspired concentration is 1%. When inspired concentration is doubled to 2%, alveolar con- centration will grow along curve Y. The more rapid rate of rise in alveolar concentration when inspired concentration is in- creased is obvious. This effect is seen with both insoluble and soluble agents. When pulmonary capillary blood takes up anesthetic vapor molecules from alveolar gas, the vapor concentration in the al- veolar gas mixture falls. Therefore, the solution of a soluble agent in pulmonary capillary blood tends to reduce the rate at which its alveolar concentration increases. This retardant effect on the rate of rise decreases as the inspired concentration in- creases. This phenomenon is called the concentration effect. It 34 Inhalation Anesthetics is an additional factor that contributes to increasing the rate of alveolar uptake of soluble agents at higher inspired concentra- tions. The importance of this factor increases with the solubil- ity of the agent. Because all volatile agents currently in use are relatively insoluble, the influence of concentration effect on the alveolar uptake of these agents can be regarded as minimal. A practical application of increasing inspired concentration on alveolar uptake is found in inhalation induction. In Figure 3 — 3B, alveolar uptake follows curve X when a low inspired concentration of inhalation anesthetic is given, but it follows curve Y when the inspired concentration is increased. The con- sequence of giving a higher inspired concentration during in- duction is a shorter induction time. Once anesthesia is estab- lished, the inspired concentration can be adjusted to lower values for maintenance (curve Z). Alveolar Ventilation Because inhalation anesthetics are delivered only to the up- per airway, from which they are inhaled, hyperventilation in- creases the rate of alveolar uptake and hypoventilation de- creases it. Functional Residual Capacity Functional residual capacity (FRC) is the volume of gases in the lungs at the end of a normal expiration; it acts as a buffer to changes in the composition of alveolar gas. The time required to complete 63% of alveolar uptake (the time constant) is re- lated to FRC and alveolar ventilation per minute (V A ) by the following formula: FRC Time constant = — =- Thus the rate of rise in alveolar concentration is fast (short time constant) when FRC is small or alveolar minute ventilation large, and the rate of rise is slow (long time constant) when FRC is large or alveolar minute ventilation small. Solubility of Anesthetic Agent The solubility of an inhalation agent in blood is defined as the amount of anesthetic agent required to saturate a unit vol- ume of blood at a given temperature, and can be expressed as Inhalation Anesthetics 35 the blood-gas partition coefficient. The relative solubility of agents in clinical use and their blood-gas partition coefficients are: desflurane (0.42) < nitrous oxide (0.47) < sevoflurane (0.65) < isoflurane (1.4) < enflurane (1.8) < halothane (2.3) < meth- oxyflurane (13.0). The more soluble the agent, the greater is the amount that will be carried away by blood in the pulmonary capillaries during uptake. Thus the rate of increase in the alve- olar uptake curve is slowed. In contrast, the alveolar concentra- tion of a relatively insoluble agent increases faster. In practical terms, the solubility of the inhalation agent in blood is the most important single factor in determining the speed of induction and of recovery in individual patients. Induction and recovery are fast with highly insoluble agents and slow with soluble ones. Cardiac Output Gases in the alveoli are in equilibrium with blood in the pul- monary capillaries. Therefore, how much anesthetic is removed from the lungs by pulmonary blood depends not only on the solubility of the agent but also on pulmonary blood flow. Alve- olar anesthetic concentration rises slowly when cardiac output (pulmonary blood flow) is high. Cerebral blood flow usually remains normal in high cardiac output states, and most of the increase in flow is distributed to other tissues. Therefore, the slower rise in alveolar anesthetic concentration will slow the induction of anesthesia. Conversely, a low cardiac output will allow a faster rise in alveolar concentration and will speed up induction, provided cerebral blood flow is maintained. Alveolar-Mixed Venous Tension Gradient At the beginning of the alveolar uptake curve, the difference between the tension (partial pressure) of the anesthetic agent in alveolar gas and that in mixed venous blood is large. This gradient enhances the uptake of anesthetic by pulmonary blood and tends to slow the increase in alveolar concentration. As tissues and blood take up more anesthetic, this gradient de- creases, and the effect of the alveolar— mixed venous tension gradient on uptake is less obvious. Second Gas Effect In previous sections it has been assumed that the volatile anesthetic is delivered to the airway in oxygen only. If a high concentration of nitrous oxide is also added to the inspired 36 Inhalation Anesthetics mixture, the uptake of a large amount of this agent into blood will decrease the volume of the mixture in the alveoli, increase the alveolar concentration of the volatile agent, and "draw in" more anesthetic mixture from the upper airway. This phenom- enon, called the second gas effect, is an additional factor that contributes to increasing the rate of alveolar uptake of a volatile agent whenever nitrous oxide is used. Practical Implications Of the factors mentioned, inspired concentration, alveolar ventilation, solubility in blood, and the second gas effect can be manipulated to promote alveolar uptake and increase the speed of induction. The inspired concentration can be set high initially, ventilation can be enhanced with assistance, and ni- trous oxide can be added to the anesthetic mixture. Choosing a less soluble agent is attractive, but the choice is often limited by other considerations (e.g., unwanted side effects). Distribution and Tissue Uptake Once an inhalation anesthetic is taken up by pulmonary blood, it isdistributed to tissues of the body according to regional blood flow (see "Tissue Blood Flow and Tissue Mass" in Chapter 2). Tissues with the richest blood supply (brain, heart, liver, kid- neys) take up the anesthetic rapidly. This rapid uptake of anes- thetic by the brain means that its anesthetic partial pressure will very quickly come into equilibrium with that in the alveoli. This accounts for the efficiency of the respiratory tract as a route for administration of these inhaled anesthetic drugs. Elimination Excretion Most of the inhalation agents are exhaled unchanged by the lungs.The fall in alveolar concentration follows an exponential decay curve (Fig. 3-4). The factors that influence this decay in alveolar concentration are exactly those that influence uptake, but the direction of the changes is reversed. Hyperventilation, a small FRC, a low solubility, a low cardiac output, or a large mixed venous— alveolar tension gradient increases the rate of Inhalation Anesthetics 37 o ~z. o u or < _i o UJ > TIME mm FIGURE 3-4. The fall in alveolar concentration of an inhalation an- esthetic during emergence. It is an exponential decay curve, exactly the reverse of the exponential uptake curve that describes the rise in alveolar concentration during induction (Refer to text for details.) decay. (Notice that "venous-alveolar" gradient instead of "al- veolar-venous" gradient is used in describing excretion). Hy- poventilation, a large FRC, a high solubility, a large cardiac output, or a small mixed venous— alveolar tension gradient de- creases the rate of this decay. Because the inspired concentra- tion of the agent is near zero during excretion, this factor has no influence on the rate of alveolar decay. Metabolism Until the mid-1960s, it was believed that all inhalation an- esthetics were exhaled unchanged. Since then, it has been ob- served that a significant portion of these inhaled agents is me- tabolized by mixed-function oxidases (the cytochrome P450 system) in the liver. The degree of biotransformation varies ac- cording to the agent, and most of the water-soluble organic and inorganic metabolites are excreted by the kidneys. The signifi- cance of these findings for individual agents is discussed in the following section. Specific Agents Nitrous Oxide Nitrous oxide is a colorless, odorless, and nonflammable gas approximately 1.5 times as heavy as air. It exists as a gas at 38 Inhalation Anesthetics room temperature and atmospheric pressure, but it can be com- pressed into a liquid unless its temperature is above 36.5° C. Medical-grade nitrous oxide is stored in cylinders as a liquid at room temperature under a pressure of 750 pounds per square inch (psi), or 50 atmospheres. This agent is a weak anesthetic. Its MAC is 104%, a value that can be achieved only in the hyperbaric chamber. Normally no more than 70% nitrous oxide is administered in clinical practice, the other 30% being oxygen. After induction of an- esthesia with an intravenous agent, it is given with a narcotic analgesic and a muscle relaxant to maintain anesthesia in the so-called balanced anesthesia technique. It is also used in com- bination with the more potent volatile agents. Because the MACs of nitrous oxide and other inhalation agents are additive (see "Minimum Alveolar Concentration" above), the use of ni- trous oxide will reduce both the requirement of these agents and their side effects. In contradistinction to its weak anesthetic property, nitrous oxide is a good analgesic. Premixed 50% ni- trous oxide in oxygen (Entonox and Nitronox) is used for pain relief in obstetrics and in dental surgery. Few side effects are attributed to nitrous oxide. It does de- press respiratory and myocardial function, but the degree of depression is small. It is also a cerebral vasodilator, particularly when it is used in combination with other volatile agents. Ter- atogenic effects have been observed in rats, and an increased incidence of spontaneous abortion