Enzymes-Part I PDF
Document Details
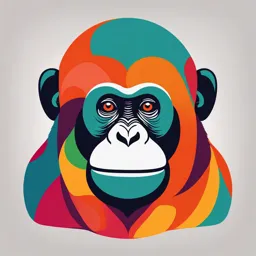
Uploaded by ForemostWendigo
VIT Vellore
A K A Mandal
Tags
Summary
This document provides an overview of enzymes, focusing on those involved in DNA manipulation. It covers different types of enzymes, their functions, and applications, offering a concise introduction to the topic.
Full Transcript
M1 Tools - The Enzymes A K A Mandal A K A Mandal 1 Introduction Pure DNA Cut Join Lengthen Shorten Modify Copy etc. All these operations make use of purified enzymes – Within the cell these enzymes participate in essent...
M1 Tools - The Enzymes A K A Mandal A K A Mandal 1 Introduction Pure DNA Cut Join Lengthen Shorten Modify Copy etc. All these operations make use of purified enzymes – Within the cell these enzymes participate in essential processes such as DNA replication and transcription, breakdown of unwanted or foreign DNA (e.g., invading virus DNA), repair of mutated DNA, and recombination between different DNA molecules – After purification from cell extracts, many of these enzymes can be persuaded to carry out their natural reactions, or something closely related to them, under artificial conditions 2 The range of DNA manipulative enzymes DNA manipulative enzymes can be grouped into four broad classes, depending on the type of reaction that they catalyze: – Nucleases - cut, shorten, or degrade nucleic acid molecules – Ligases - join nucleic acid molecules together – Polymerases - make copies of molecules – Modifying enzymes - remove or add chemical groups A K A Mandal 3 Before considering in detail each of these classes of enzyme, two points should be made: The first is that, although most enzymes can be assigned to a particular class, a few display multiple activities that span two or more classes. Most importantly, many polymerases combine their ability to make new DNA molecules with an associated DNA degradative (i.e., nuclease) activity Second, it should be appreciated that, as well as the DNA manipulative enzymes, many similar enzymes able to act on RNA are known. The ribonuclease used to remove contaminating RNA from DNA preparations is an example of such an enzyme. Although some RNA manipulative enzymes have applications in gene cloning we will in general restrict our thoughts to those enzymes that act on DNA A K A Mandal 4 Nucleases Nucleases degrade DNA molecules by breaking the phosphodiester bonds that link one nucleotide to the next in a DNA strand. There are two different kinds of nuclease: – Exonucleases - remove nucleotides one at a time from the end of a DNA molecule – Endonucleases - are able to break internal phosphodiester bonds within a DNA molecule A K A Mandal 5 The reactions catalyzed by the two different kinds of nuclease: (a) An exonuclease, which removes nucleotides from the end of a DNA molecule. (b) An endonuclease, which breaks internal phosphodiester bonds. A K A Mandal 6 Exonucleases The reactions catalyzed by different types of exonuclease. (a) Bal31, which removes nucleotides from both strands of a double-stranded molecule (b) Exonuclease III, which removes nucleotides only from the 3′ terminus 7 The main distinction between different exonucleases lies in the number of strands that are degraded when a double- stranded molecule is attacked. The enzyme called Bal31 (purified from the bacterium Alteromonas espejiana) is an example of an exonuclease that removes nucleotides from both strands of a double-stranded molecule. The greater the length of time that Bal31 is allowed to act on a group of DNA molecules, the shorter the resulting DNA fragments will be. In contrast, enzymes such as E. coli exonuclease III degrade just one strand of a double-stranded molecule, leaving single- stranded DNA as the product A K A Mandal 8 Endonucleases The same criterion can be used to classify endonucleases – S1 endonuclease (from the fungus Aspergillus oryzae) only cleaves single strands – Deoxyribonuclease I (DNase I), which is prepared from cow pancreas, cuts both single and double-stranded molecules. DNase I is non-specific in that it attacks DNA at any internal phosphodiester bond, so the end result of prolonged DNase I action is a mixture of mononucleotides and very short oligonucleotides – On the other hand, the special group of enzymes called restriction endonucleases (RE) cleave double stranded DNA only at a limited number of specific recognition sites A K A Mandal 9 The reactions catalyzed by different types of endonuclease. (a) S1 nuclease, which cleaves only single-stranded DNA, including single-stranded nicks in mainly double-stranded molecules. (b) DNase I, which cleaves both single- and double-stranded DNA. (c) A restriction endonuclease, which cleaves double-stranded DNA, but only at a limited number of sites A K A Mandal 10 Ligases In the cell the function of DNA ligase is to repair single-stranded breaks (“discontinuities”) that arise in double-stranded DNA molecules during, for example, DNA replication DNA ligases from most organisms can also join together two individual fragments of double- stranded DNA A K A Mandal 11 The two reactions catalyzed by DNA ligase (a) Repair of a discontinuity—a missing phosphodiester bond in one strand of a doublestranded Molecule (b) Joining two molecules together A K A Mandal 12 Polymerases Most polymerases can function only if the template possesses a double-stranded region that acts as a primer for initiation of polymerization A K A Mandal 13 Polymerases Four types of DNA polymerase are used routinely in genetic engineering. – DNA polymerase I - usually prepared from E. coli. This enzyme attaches to a short single-stranded region (or nick) in a mainly double-stranded DNA molecule, and then synthesizes a completely new strand, degrading the existing strand as it proceeds. DNA polymerase I is therefore an example of an enzyme with a dual activity—DNA polymerization and DNA degradation A K A Mandal 14 Polymerases – The polymerase and nuclease activities of DNA polymerase I are controlled by different parts of the enzyme molecule. The nuclease activity is contained in the first 323 amino acids of the polypeptide, so removal of this segment leaves a modified enzyme that retains the polymerase function but is unable to degrade DNA. This modified enzyme, called the Klenow fragment, can still synthesize a complementary DNA strand on a single-stranded template, but as it has no nuclease activity it cannot continue the synthesis once the nick is filled in. Several other enzymes—natural polymerases and modified versions—have similar properties to the Klenow fragment. The major application of these polymerases is in DNA sequencing. A K A Mandal 15 Polymerases – The Taq DNA polymerase used in the polymerase chain reaction (PCR) is the DNA polymerase I enzyme of the bacterium Thermus aquaticus. This organism lives in hot springs, and many of its enzymes, including the Taq DNA polymerase, are thermostable, meaning that they are resistant to denaturation by heat treatment. This is the special feature of Taq DNA polymerase that makes it suitable for PCR, because if it was not thermostable it would be inactivated when the temperature of the reaction is raised to 94°C to denature the DNA A K A Mandal 16 Polymerases – The final type of DNA polymerase that is important in genetic engineering is reverse transcriptase, an enzyme involved in the replication of several kinds of virus. Reverse transcriptase is unique in that it uses as a template not DNA but RNA – The ability of this enzyme to synthesize a DNA strand complementary to an RNA template is central to the technique called complementary DNA (cDNA) cloning A K A Mandal 17 The reactions catalyzed by DNA polymerases. (a) The basic reaction: a new DNA strand is synthesized in the 5′ to 3′ direction. (b) DNA polymerase I, which initially fills in nicks but then continues to synthesize a new strand, degrading the existing one as it proceeds. (c) The Klenow fragment, which only fills in nicks. (d) Reverse transcriptase, which uses a template of RNA. A K A Mandal 18 DNA modifying enzymes There are numerous enzymes that modify DNA molecules by addition or removal of specific chemical groups. The most important are as follows: – Alkaline phosphatase (from E. coli, calf intestinal tissue, or arctic shrimp), which removes the phosphate group present at the 5′ terminus of a DNA molecule A K A Mandal 19 DNA modifying enzymes – Polynucleotide kinase (from E. coli infected with T4 phage), which has the reverse effect to alkaline phosphatase, adding phosphate groups onto free 5′ termini A K A Mandal 20 DNA modifying enzymes – Terminal deoxynucleotidyl transferase (from calf thymus tissue), which adds one or more deoxyribonucleotides onto the 3′ terminus of a DNA molecule A K A Mandal 21 The reactions catalyzed by DNA modifying enzymes. (a) Alkaline hosphatase, which removes 5′- phosphate groups. (b) Polynucleotide kinase, which attaches 5′-phosphate groups. (c) Terminal deoxynucleotidyl transferase, which attaches deoxyribonucleotides to the 3′ termini of polynucleotides in either (i) single-stranded or (ii) double-stranded molecules. A K A Mandal 22 Restriction Endonuclease (RE) A K A Mandal 23 Enzymes for cutting DNA—Restriction Endonucleases Gene cloning requires that DNA molecules be cut in a very precise and reproducible fashion. This is illustrated by the way in which the vector is cut during construction of a recombinant DNA molecule. Each vector molecule must be cleaved at a single position, to open up the circle so that new DNA can be inserted: a molecule that is cut more than once will be broken into two or more separate fragments and will be of no use as a cloning vector. Furthermore, each vector molecule must be cut at exactly the same position on the circle, random cleavage is not satisfactory. It should be clear that a very special type of nuclease is needed to carry out this manipulation. A K A Mandal 24 Often it is also necessary to cleave the DNA that is to be cloned. There are two reasons for this First, if the aim is to clone a single gene, which may consist of only 2 or 3 kb of DNA, then that gene will have to be cut out of the large (often greater than 80 kb) DNA molecules produced by skilfull use of the preparative techniques Second, large DNA molecules may have to be broken down simply to produce fragments small enough to be carried by the vector. Most cloning vectors exhibit a preference for DNA fragments that fall into a particular size range: most plasmid- based vectors, for example, are very inefficient at cloning DNA molecules more than 8 kb in length A K A Mandal 25 Purified restriction endonucleases allow the molecular biologist to cut DNA molecules in the precise, reproducible manner required for gene cloning. The discovery of these enzymes, which led to Nobel Prizes for W. Arber, H. Smith, and D. Nathans in 1978, was one of the key breakthroughs in the development of genetic engineering A K A Mandal 26 The need for very precise cutting manipulations in a gene cloning experiment. A K A Mandal 27 The function of a restriction endonuclease in a bacterial cell: (a) phage DNA is cleaved, but (b) bacterial DNA is not A K A Mandal 28 These degradative enzymes are called restriction endonucleases and are synthesized by many, perhaps all, species of bacteria: over 2500 different ones have been isolated and more than 300 are available for use in the laboratory. A K A Mandal 29 Before 1970 there was no method for cleaving DNA at discrete points. All the available methods for fragmenting DNA were non- specific. The available endonucleases had little site specificity and chemical methods produced very small fragments of DNA. The only method where any degree of control could be exercised was the use of mechanical shearing. A K A Mandal 30 During the 1960s, phage biologists elucidated the biochemical basis of the phenomenon of host restriction and modification. The culmination of this work was the purification of the restriction endonuclease of Escherichia coli K12 by Meselson and Yuan (1968). Since this endonuclease cuts unmodified DNA into large discrete fragments, it was reasoned that it must recognize a target sequence. This in turn raised the prospect of controlled manipulation of DNA. Unfortunately, the K12 endonuclease turned out to be perverse in its properties. While the enzyme does bind to a defined recognition sequence, cleavage occurs at a “random” site several kilobases away. A K A Mandal 31 The much sought-after breakthrough finally came in 1970 with the discovery in Hemophilus influenzae (Kelly & Smith 1970, Smith & Wilcox 1970) of an enzyme that behaves more simply. That is, the enzyme recognizes a particular target sequence in a duplex DNA molecule and breaks the polynucleotide chain within that sequence to give rise to discrete fragments of defined length and sequence. The presence of restriction and modification systems is a double-edged sword. On the one hand, they provide a rich source of useful enzymes for DNA manipulation. On the other, these systems can significantly affect the recovery of recombinant DNA in cloning hosts. For this reason, some knowledge of restriction and modification is essential. A K A Mandal 32 Four different types of restriction and modification (R- M) system have been recognized but only one is widely used in gene manipulation At least four different kinds of R-M system are known: – type I – type II – type III – type IIs A K A Mandal 33 The type I systems The first to be characterized and a typical example is that from E. coli K12 The active enzyme consists of two restriction subunits, two modification (methylation) subunits, and one recognition subunit. These subunits are the products of the hsdR, hsdM, and hsdS genes The methylation and cutting reactions both require ATP and S- adenosylmethionine as cofactors The recognition sequences are quite long with no recognizable features such as symmetry The enzyme cuts unmodified DNA at some distance from the recognition sequence These features mean that type I systems are of little value for gene manipulation A K A Mandal 34 The type III systems Type III enzymes have symmetrical recognition sequences but otherwise resemble type I systems and are of little value A K A Mandal 35 The type II systems Most of the useful R-M systems are of type II. They have a number of advantages over type I and III systems First, restriction and modification are mediated by separate enzymes so it is possible to cleave DNA in the absence of modification Secondly, the restriction activities do not require cofactors such as ATP or S-adenosylmethionine, making them easier to use Most important of all, type II enzymes recognize a defined, usually symmetrical, sequence and cut within it Many of them also make a staggered break in the DNA and the usefulness of this will become apparent A K A Mandal 36 The type IIs systems Although type IIs systems have similar cofactors and macromolecular structure to those of type II systems, the fact that restriction occurs at a distance from the recognition site limits their usefulness A K A Mandal 37 The classification of R-M systems into types I to III is convenient but may require modification as new discoveries are made. For example, the Eco571 system comprises a single polypeptide which has both restriction and modification activities (Petrusyte et al. 1988). Other restriction systems are known which fall outside the above classification. Examples include the mcr and mrr systems and homing endonucleases. The latter are double-stranded deoxyribonucleases (DNases) derived from introns or inteins (Belfort & Roberts 1997). They have large, asymmetric recognition sequences and, unlike standard restriction endonucleases, tolerate some sequence degeneracy within their recognition sequence A K A Mandal 38 A K A Mandal 39 The naming of restriction endonucleases provides information about their source The discovery of a large number of restriction and modification systems called for a uniform system of nomenclature. A suitable system was proposed by Smith and Nathans (1973) and a simplified version of this is in use today. The key features are: – The species name of the host organism is identified by the first letter of the genus name and the first two letters of the specific epithet to generate a three-letter abbreviation. This abbreviation is always written in italics – Where a particular strain has been the source then this is identified – When a particular host strain has several different R-M systems, these are identified by roman numerals A K A Mandal 40 – Homing endonucleases are named in a similar fashion except that intron-encoded endonucleases are given the prefix “I-” (e.g. I-CeuI) and intein endonucleases have the prefix “PI-” (e.g. Pl-PspI) – Where it is necessary to distinguish between the restriction and methylating activities, they are given the prefixes “R” and “M”, respectively, e.g. R.SmaI and M.SmaI A K A Mandal 41 Examples of restriction endonuclease nomenclature A K A Mandal 42 Type II restriction endonucleases cut DNA at specific nucleotide sequences The central feature of type II restriction endonucleases (which will be referred to simply as “restriction endonucleases” from now on) is that each enzyme has a specific recognition sequence at which it cuts a DNA molecule. A particular enzyme cleaves DNA at the recognition sequence and nowhere else. For example, the restriction endonuclease called PvuI (isolated from Proteus vulgaris) cuts DNA only at the hexanucleotide CGATCG In contrast, a second enzyme from the same bacterium, called PvuII, cuts at a different hexanucleotide, in this case CAGCTG WHY? A K A Mandal 43 Many restriction endonucleases recognize hexanucleotide target sites, but others cut at four, five, eight, or even longer nucleotide sequences. Sau3A (from Staphylococcus aureus strain 3A) recognizes GATC, and AluI (Arthrobacter luteus) cuts at AGCT There are also examples of restriction endonucleases with degenerate recognition sequences, meaning that they cut DNA at any one of a family of related sites. HinfI (Haemophilus influenzae strain Rf), for instance, recognizes GANTC, so cuts at GAATC, GATTC, GAGTC, and GACTC A K A Mandal 44 The recognition sequences for some of the most frequently used restriction endonucleases A K A Mandal 45 Restriction enzymes cut DNA at sites of rotational symmetry and different enzymes recognize different sequences Most, but not all, type II restriction endonucleases recognize and cleave DNA within particular sequences of four to eight nucleotides which have a twofold axis of rotational symmetry. Such sequences are often referred to as palindromes because of their similarity to words that read the same backwards as forwards For example, the restriction and modification enzymes R.EcoRI and M.EcoRI recognize the sequence: 5′-G A A T T C-3′ 3′-C T T A A G-5′ Axis of symmetry A K A Mandal 46 The position at which the restricting enzyme cuts is usually shown by the symbol “/” and the nucleotides methylated by the modification enzyme are usually marked with an asterisk. For EcoRI these would be represented thus: 5′-G/AA*T T C-3′ 3′-C T T A*A/G-5′ For convenience it is usual practice to simplify the description of recognition sequences by showing only one strand of DNA, that which runs in the 5′ to 3′ direction. Thus the EcoRI recognition sequence would be shown as G/AATTC A K A Mandal 47 Not all type II enzymes cleave their target sites like EcoRI. Some, such as PstI (CTGCA/G), produce fragments bearing 3′ overhangs, while others, such as SmaI (CCC/GGG), produce blunt or flush ends A K A Mandal 48 Restriction enzymes with the same sequence specificity and cut site are known as isoschizomers. For example, SphI (CGTAC/G) and BbuI (CGTAC/G) are isoschizomers of each other. The first enzyme discovered which recognizes a given sequence is known as the prototype; all subsequently identified enzymes that recognize that sequence are isoschizomers. Isoschizomers are isolated from different strains of bacteria and therefore may require different reaction conditions https://www.neb.com/tools-and-resources/selection-charts/isoschizomers A K A Mandal 49 Enzymes that recognize the same sequence but cleave at different points, for example SmaI (CCC/GGG) and XmaI (C/CCGGG), are sometimes known as neoschizomers A K A Mandal 50 Under extreme conditions, such as elevated pH or low ionic strength, restriction endonucleases are capable of cleaving sequences which are similar but not identical to their defined recognition sequence. This altered specificity is known as star activity. The most common types of altered activity are acceptance of base substitutions and truncation of the number of bases in the recognition sequence. For example, EcoRI* (EcoRI star activity) cleaves the sequence N/AATTN, where N is any base, whereas EcoRI cleaves the sequence GAATTC A K A Mandal 51 A K A Mandal 52 For a comprehensive database of information on restriction endonucleases and their associated methylases, including cleavage sites, commercial availability, and literature references, the reader should consult the website maintained by New England Biolabs http://rebase.neb.com/rebase/rebase.html https://www.re3data.org/ A K A Mandal 53 Nature of cut - Blunt ends and Sticky ends The exact nature of the cut produced by a restriction endonuclease is of considerable importance in the design of a gene cloning experiment Blunt ends Many restriction endonucleases make a simple double-stranded cut in the middle of the recognition sequence, resulting in a blunt end or flush end. PvuII and AluI are examples of blunt end cutters A K A Mandal 54 Sticky ends Other restriction endonucleases cut DNA in a slightly different way. With these enzymes the two DNA strands are not cut at exactly the same position. Instead the cleavage is staggered, usually by two or four nucleotides, so that the resulting DNA fragments have short single-stranded overhangs at each end. These are called sticky or cohesive ends, as base pairing between them can stick the DNA molecule back together again A K A Mandal 55 One important feature of sticky end enzymes is that restriction endonucleases with different recognition sequences may produce the same sticky ends BamHI (recognition sequence GGATCC) and BglII (AGATCT) are examples— both produce GATC sticky ends. The same sticky end is also produced by Sau3A, which recognizes only the tetranucleotide GATC. Fragments of DNA produced by cleavage with either of these enzymes can be joined to each other, as each fragment carries a complementary sticky end A K A Mandal 56 The ends produced by cleavage of DNA with different restriction endonucleases (a) A blunt end produced by AluI. (b) A sticky end produced by EcoRI. (c) The same sticky ends produced by BamHI, BglII and Sau3A A K A Mandal 57 The frequency of recognition sequences in a DNA molecule The number of recognition sequences for a particular restriction endonuclease in a DNA molecule of known length can be calculated mathematically A tetranucleotide sequence (e.g., GATC) should occur once every 44 = 256 nucleotides, and a hexanucleotide (e.g., GGATCC) once every 46 = 4096 nucleotides. These calculations assume that the nucleotides are ordered in a random fashion and that the four different nucleotides are present in equal proportions (i.e., the GC content = 50%) A K A Mandal 58 The frequency of recognition sequences in a DNA molecule In practice, neither of these assumptions is entirely valid. For example, the DNA molecule, at 49 kb, should contain about 12 sites for a restriction endonuclease with a hexanucleotide recognition sequence. In fact, many of these recognition sites occur less frequently (e.g., six for BglII, five for BamHI, and only two for SalI), a reflection of the fact that the GC content for is rather less than 50% A K A Mandal 59 Furthermore, restriction sites are generally not evenly spaced out along a DNA molecule. If they were, then digestion with a particular restriction endonuclease would give fragments of roughly equal sizes. Figure b shows the fragments produced by cutting DNA with BglII, BamHI, and SalI. In each case there is a considerable spread of fragment sizes, indicating that in DNA the nucleotides are not randomly ordered A K A Mandal 60 The lesson to be learned from is that although mathematics may give an idea of how many restriction sites to expect in a given DNA molecule, only experimental analysis can provide the true picture A K A Mandal 61 Restriction of the λ DNA molecule. (a) The positions of the recognition sequences for BglII, BamHI, and SalI. (b) The fragments produced by cleavage with each of these restriction endonucleases. The numbers are the fragment sizes in base pairs A K A Mandal 62