ENS 413 Unit 1: Atmospheric Chemistry, Meteorology, and Climatology PDF
Document Details
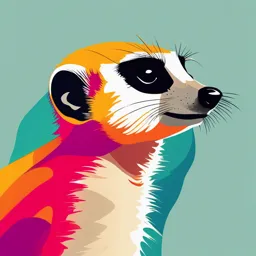
Uploaded by MindBlowingOctopus
Sree Vasavi Vidya Peetha
Dr. M T Das
Tags
Summary
This document provides a lecture outline on atmospheric chemistry, meteorology, and climatology, covering topics such as atmospheric composition, vertical variations, optical phenomena, and solar radiation. The document likely serves as a study guide for an undergraduate course.
Full Transcript
Lecture Outline-Dr. M T Das ENS 413- Atmospheric Chemistry, Meteorology and Climatology UNIT I Structure Composition and Radiation Physics of the Atmosphere Composition of the atmosphere (variable and stable components), Vertical variations in atmosphere (atm...
Lecture Outline-Dr. M T Das ENS 413- Atmospheric Chemistry, Meteorology and Climatology UNIT I Structure Composition and Radiation Physics of the Atmosphere Composition of the atmosphere (variable and stable components), Vertical variations in atmosphere (atmospheric layers, pressure change, temperature change, change in composition, Ionosphere); Optical phenomena of the atmosphere (Basic definitions of Scattering, Reflection, Refraction, Dispersion and Diffraction and their role in formation of Mirage, Rainbow, Halos, Sun Dogs, Solar Pillars, Corona, iridescent clouds and the Auroras) Solar radiation and earth’s energy budget (solar constant, albedo, earth’s effective black body temperature and actual surface temperature, net energy budget) 1. Composition of the atmosphere Our atmosphere is composed of several constituents which can be grouped in to two major categories. The variable components whose concentration in air is not constant, it varies from time to time and from place to place. Important examples include water vapour, aerosol, and ozone. If the variable components are removed from the atmosphere, the remaining air is clean dry and is composed of the stable components whose concentrations does not change within an altitude of 80 km (i.e. up to mesosphere). The clean and dry atmosphere mainly consists of nitrogen (78 %) and oxygen (21 %). The remaining one percent of air is mostly the inert gas argon (0.93 %), carbon dioxide (0.0391 %). All the constituents of the atmosphere have their own roles in sustaining the intricate balance of our planet. However, from the meteorological and climatological purposes, the trace gases (CO2, Water vapor, Ozone, Methane, CFCs, Nitrous oxide) play a major role. 2. Vertical variations in atmosphere The atmosphere is divided into four distinct layers based on their temperature profile: the troposphere, stratosphere, mesosphere, and thermosphere. The composition of gases in the atmosphere from the ground to the top of the mesosphere, are almost identical except for the variable components (i.e. water vapor, aerosols, and ozone). Therefore, the region below the mesosphere is also called the homosphere i.e. the zone of homogenous composition. In contrast, the atmosphere in the thermosphere region is not uniform. It has a heterogeneous composition; hence it is called the heterosphere. The pressure variation is more prominent in the lower 35 kilometers where atmospheric pressure decreases with altitude. Beyond 35km the decrease in pressure is negligible as the density of air becomes very low. Half of the mass of our atmosphere lies below and altitude of 5.6 km. 1 Troposphere: It is the lowest layer which extends up to an average height of 12 km. The depth of the troposphere varies with latitude and season (16 km in tropics and 9 km in poles). It accounts for about three quarters of the mass of the atmosphere and contains nearly all of the water associated with the atmosphere (vapor, clouds and precipitation). Therefore; all the weather phenomena occur in the troposphere and the air flow is highly turbulent. The temperature in the troposphere usually decreases with height at the average lapse rate of 6.5 °C per kilometer (environmental lapse rate). Tropospheric decrease in temperature is due to decrease in atmospheric pressure. Stratosphere: extends from 12 kilometers to 50 kilometers above ground. Within the lower part of stratosphere remains the tropopause (Starts at 12km up to 20 km) where temperature is almost constant. Above 20 kilometers the temperature increases with height at the average rate of 5 °C per kilometer. The increase in temperature is due to absorption of UV radiation by ozone. Most of the atmospheric ozone is concentrated in the stratosphere forming the ozone layer. Since almost no dust or water vapor from the land surface reaches the stratosphere, the air flow in this layer is steady. Mesosphere: Extends from 50kilometers to 80 kilometers above ground. Within the lower part of mesosphere remains the stratropause(Starts at 50km up to 55 km) where temperature is almost constant. Beyond the stratopause the temperature usually decreases with height up to the top of the mesosphere where the temperature can be as low as - 95 °C or even lower. It is the coldest region of the atmosphere. The decrease in temperature is due to drastic decrease in atmospheric pressure. Moreover there is no radiation absorbing gases like ozone in this layer. Mesosphere is the least explored layer as it cannot be reached by any research balloons or jet airplanes. Nor it is accessible to any lower orbiting satellite. Thermosphere: It is the region above the top of mesosphere where the temperature begins to rise again after a short mesopause (80-90 km). Thermosphere has no defined upper limit in altitude. The rise in temperature is due to the very high molecular speed of the gases. The air in the thermosphere is extremely thin thus the molecular mean free path becomes very large. Thermosphere is otherwise known as heterosphere. In heterosphere molecular diffusion becomes dominant over turbulent mixing. So under equilibrium conditions (steady state, no sources and sinks of constituents), each gas is arranged in an individual layer. This equilibrium distribution is called diffusive equilibrium. In diffusive equilibrium, heavy gases that lighter gases separate from each other with the lighter gases rising to the top. Thus in 2 thermosphere four distinct layers are found consisting of nitrogen (at the bottom of thermosphere), followed by oxygen, helium and hydrogen (at the top). In the lower thermosphere (80 – 400 Km) an electrically charged region is there which is known as ionosphere. Here, the fast moving gaseous molecules are ionized by high energy shortwave solar energy. In this process gaseous molecules/atoms loose one or more electron and become positively charged ions. The electrons are set free to travel as electric currents. Ionization also occurs in the upper part of mesosphere. But the ions reunite to form molecules as the air density is higher. The electrical property of ionosphere is very effective in reflecting radio waves. So, most of the artificial satellites revolve around earth in this layer. The electrical structure of ionosphere is not uniform. It consists of three layers called D, E F layers. 3. Optical phenomena of the atmosphere The Auroras An aurora is a natural light display in the sky, particularly in the Polar Regions, during the night. In the northern hemisphere the aurora is called aurora borealis and in the southern hemisphere it is called aurora australis. It is an optical phenomenon of the ionosphere. Sun emits enormous amount of fast movingcharged particles (protons and electrons) during the massive magnetic storms known as solar flares. These charged particles are directed by the earth's magnetic field towards the magnetic poles. As the charged particles enter the ionosphere they excite the nitrogen molecules and oxygen atoms and cause them to emit light. Why is the sky blue? Why are some clouds white and others dark? Nitrogen, the most abundant gas in the atmosphere, is a selective scatterer and scatters shorter wavelengths of visible light (violet, blue and green) more efficiently than longer wavelengths (yellow, orange and red). This scattering is called 'Rayleigh scattering'. Rayleigh scattering is applicable when the radius (r) of the scattering sphere is much smaller than the wavelength (λ) of the incident light. As per 'Rayleigh scattering' formula, the amount of scattering is inversely proportional the wavelength of the light to the 4th power. As the nitrogen and oxygen molecules in the atmosphere, follow Rayleigh scattering, violet-indigo-blue lights which have smaller wave length gets scattered to a much larger extent and as human eye is more sensitive to blue light, the sky looks blue. Hazy atmosphere occurs when small particles (dust and sea salt) are suspended in the atmosphere. These particles scatter all the wavelengths of light (Mie scattering) and we see a whitish or grayishshade instead of clear blue. The more particles that are suspended in the atmosphere, the whiter the sky appears. Clouds appear white because countless numbers of cloud droplets ice crystals scatter all the wavelengths of visible light in all directions (Mie scattering). Dark clouds are seen when the cloud grows thicker, larger and taller and very little light penetrates through to the base of the cloud. The raindrops at the cloud base also are so large that they cannot scatter efficiently but rather absorb the energy. 3 Why the sun looks red at the horizon? During sunrise or sunset, the sun’s rays strike the atmosphere at a lower angle than during the day. Thus the light penetrates a deeper layer of atmosphere and most of the shorter wavelength of visible light (violet, blue and green) are scattered away. Therefore, when the light finally reaches our eyes, we see the longer wavelengths (orange‐yellow). Bright yellow‐orange sunsets occur when the atmosphere is fairly clean. A more polluted atmosphere gives a red sunrise / sunset. Formation of Mirage Refraction plays a role in formation of mirages. As light travels from a less dense medium to a denser medium at an angle, the speed of light decreased and light is bent toward the normal. As light travels from a dense medium to a less dense medium at an angle, the speed of light increased and light is bent away from the normal. Inferior mirage: (Example: desert mirage) When the sun heats the earth’s surface the air closer to the ground is warmer and less dense than layers of air higher in the atmosphere. This leads the light to follow a concave path to reach our eye from an object. As our brain perceives light a following a straight path, the object appears below its original position and is often inverted. Superior mirage or looming: (Example: apparent displacement of sun’s position during sun rise)When the earth’s surface is cooler at the end of night, the air closer to the ground is cooler and more dense than layers of air higher in the atmosphere. This leads the light to follow a convex path along the curvature of earth to reach our eye from an object (rising sun). Thus apparent displacement of sun’s position occurs during sun rise, and it appears above the horizon even though actually it is still below the horizon. Formation of Rainbow 4 A rainbow is formed by dispersion of sunlight by water droplets due to a combination of refraction and total internal reflection. Three things must happen to see a rainbow'. First, the sun must be shining. Second, the sun must be behind the viewer, and third, there must be many water drops in the air in front of the viewer. Sunlight shines into the water drops, which act as tiny prisms that bend or "refract" the light and separate it into colors. If rainbow is formed after one internal reflection in the droplets it is called a primary rainbow. The primary rainbow is brighter and has a smaller curvature. The bright primary rainbows are formed when the water drops are large, usually right after a rain shower. The viewing angle of primary rainbow is 40.8° (Blue) -42.8(Red) Often a second faint rainbow is seen above the brighter one known as the secondary rainbow. In a secondary rainbow, the colour sequence appears in the opposite order. Secondary rainbow is formed after two internal reflections in the smaller droplets. It has a wider radius than the primary rainbow, with red on the bottom and indigo on the top. The viewing angle of secondary rainbow is 52° (Red) -54° (Blue) Formation of Halo: A halo is a dispersion phenomenon produced by ice crystals creating a single ring of color around the sun or moon after a combination of refraction and total internal reflection. It occurs more frequently than a rainbow. They are produced by the ice crystals in cirrus clouds high (5–10 km, or 3–6 miles) in the upper troposphere. The shape and orientation of the crystals is responsible for the type of halo observed.Six‐sided ice crystals that make up those clouds act as the prisms that separate the sun’s light into colours. In ancient times halos were used as an empirical means of weather forecasting before meteorology was developed. Formation of Sun dogs: Sun dogs or parhelia are optical phenomena that create bright spots of light in thesky, often on a luminous ring or halo on either side of the sun. When light refracts through flat horizontal ice crystals, bright spots of light are seen along the right and left sides of a halo. Sundogs are best seen and are most prominent when the sun is near the horizon. Formation of Corona:They are look similar to halos but are formed by diffraction from a cloud's (altostratus and altocumulus) water drops rather than dispersion from icecrystals. Light shines through cloud droplets, curves around the circular drops and gets diffracted. Moreover, halos are seen at a particular viewing angle (42° or 22°) where as corona are seen at variable viewing angles. When all the cloud droplets are about the same size, the diffracted light can make thecorona separate into colors. These colors may repeat themselves. Formation of iridescence Cloud:This phenomenon involves the occurrence of colours in a cloud. Iridescent clouds are a diffraction phenomenon. Small water droplets or even small ice crystals in clouds individually diffract light. It is a fairly common phenomenon and is usually observed in altocumulus, cirrocumulus and lenticular clouds. Iridescence is most frequently seen near to the sun with the sun's glare masking it. Large ice crystals produce halos, which are dispersion phenomena rather than diffraction. The cloud must be optically thin so that most rays encounter only a single droplet. Iridescence is therefore mostly seen at cloud edges or in semi‐transparent clouds. 5 Sun pillars A sun pillar is a vertical shaft of light extending upward or downward from the sun, typically seen during sunrise or sunset. Sun pillars form when sunlight (or another bright light source) reflects off the surfaces of millions of falling ice crystals associated with thin, high-level clouds, for example, cirrostratus clouds. The ice crystals have roughly horizontal faces. 4. Solar radiation, Earth’s effective black body temperature and earth’s energy budget Solar constant: It is the average amount of radiation received annually at a point that is located perpendicular to the sun's rays, at the outer atmosphere of earth at a mean distance from the sun. Solar constant varies slightly depending on the energy output of the sun and the distance of the earth relative to the sun. Currently it is equal to 1370 Watt/m2. Insolation: The amount of incoming solar radiation received at a particular time and location on the earth surface is called insolation. Insolation is governed by various factors including Solar constant, Transparency of the atmosphere, Daily sunlight duration and Angle at which the sun's rays strike the earth Albedo: The fraction (or percentage) of incoming solar energy that is reflected back to space is known as albedo. Different surfaces (water, snow, sand, etc.) have different albedo values. For the earth and atmosphere as a whole, the average albedo is 31% (0.31 fraction) for average conditions of cloudiness over the earth. This reflectivity is greatest in the visible range of wavelengths. Earth’s effective black body temperature From the above figure it is clear that the solar energy passing through the hoop with same radius as that of earth can only reach earth. The radiation that misses the hoop also misses the earth The average amount of radiation received annually at a point at the outer atmosphere of earth = S (solar constant) So the total amount of radiation that reaches earth = SπR2(Watts) The fraction of incoming solar energy that is reflected back to space i.e. albedo = α = 0.31 So the total amount of radiation reflected by earth = SπR2 α the total amount of radiation absorbed by earth = SπR2—SπR2 α = SπR2 (1—α)---1 If the earth's surface absorbs more energy than it radiates, it will heated up. If the earth's surface radiates more energy than it absorbs, it will cooled down. But this does not happen, i.e. earth's annual average temperature remains constant. This indicates that earth behaves more or less like a black body. That means the total amount of radiation absorbed by earth must be equal to the total amount of energy radiated back to space by it. As per Stefan-Boltzmann’s law- energy radiated by a black body is proportional to its surface area and to the fourth power of its absolute temperature. i.e. the total amount of energy radiated back to space by earth α Total surface area (4πR2) α fourth power of its absolute temperature (T e4) Thus, the total amount of energy radiated back to space by earth = σ 4πR2Te4 ------2 Where σ = Stefan-Boltzmann’s constant = 5.67 × 10-8 W/m2 K4; Te = Earth’s effective black body temperature 6 As earth behaves like a black body: amount of radiation absorbed by earth must be equal to the amount of energy radiated back to space So SπR2 (1—α) = σ 4πR2Te4 Solving for Te Te = [S(1—α)/4σ]1/4 Te = [{1370 W/m2 (1 – 0.31) /4} 5.67 × 10-8 W/m2 K4]¼ = 254 K = -19°C Thus, earth’s effective black body temperature is -19°C which is 34°C less than the actual surface temperature. The actual surface temperature of earth is 288 K = 15°C. The difference between the effective black body temperature and actual surface temperature is due to the greenhouse effect. Wien’s displacement law and black body spectrum The wavelength, at which a black body spectrum peaks, is inversely proportional to its black body temperature. i.e. mathematically λmax (µm) = 2898/T (K) The sun can be represented as a black body with temperature = 5800 K So the spectrum of sun peaks at 0.5µm [i.e. the visible wavelength range (0.38 to 0.78 μm) of the electromagnetic spectrum]. Solar radiations of wavelengths longer than 3 μm are strongly absorbed by water vapor and carbon dioxide in the atmosphere. Radiation of wavelengths less than 0.29 μm is absorbed high in the atmosphere by oxygen, ozone. Therefore, solar radiation striking the earth (troposphere) generally has a short wavelength between 0.29 and 3 μm. The spectra of sun and earth is shown in following figure. Our earth can be represented as a black body with temperature = 288 K So the spectrum of earth peaks at 10.1µm. Therefore, terrestrial radiation outgoing from the surface of earth generally has a longer wavelength i.e. these radiations behave as thermal radiations. Most of the longer wavelength radiations emitted by earth is absorbed by different green house gases (H 2O, CO2, CH4, N2O). Only some of the radiations of wavelength 7-9.5 µm and 10.6-12µm are not absorbed by any green house gases and they directly escape in to the space. This is referred as atmospheric radiative window. Global Net Energy Budget (Balance) or RadiationBudget (Balance) In order to balance the incoming solar radiation and outgoing terrestrial radiation, green house effect is to be considered. The balanced state is represented mathematically in the following equations: The total amount of radiation that reaches earth = SπR2 The total amount of radiation that reaches per unit surface area of earth = SπR2/4πR2= S/4 = 1370/4 =342 W/m2 7 The total amount of radiation reflectedper unit surface area of earth = 342 × 0.31 = 106 W/m2 The total amount of radiation absorbed per unit surface area of earth= 342 -106 =236 W/m2 Out of this 236 W/m2 absorbed radiations 68 W/m2 is absorbed by the atmospheric gases and the remaining i.e. 168 W/m2 is absorbed by surface. Now let us check the outgoing terrestrial radiation: The total amount of energy radiated back to space by earth = σ 4πR2Ts4 Where Ts = actual surface temperature of earth The total amount of energy radiated back to space per unit surface area of earth = σ 4πR2Ts4/4πR2 = σTs4 = 5.67 × 10-8 (288)4 = 390 W/m2 Out of this 390 W/m2 terrestrial radiations only 40 W/m2 passes directly through the atmospheric radiative window (i.e. radiations that are not absorbed by any green house gases). The remaining 350 W/m 2 is absorbed by green house gases. The atmosphere then radiates 324 W/m2 back to the surface. Convective heat transfer from the earth surface to the atmosphere = 24 W/m2 Latent heat release into the atmosphere during condensation = 78 W/m2 All the above discussions are shown graphically in the following figure. If this global energy balance model is internally self-consistent, then the total amount of energy gain must be equal to the total amount energy loss in three regions: space, atmosphere and earth surface. The necessary checks are represented in following table: Region Amount energy gain Amount energy loss Remark Earth’s surface 168 +324 390 +24 +78 (Checks) Atmosphere 68 + 78 + 24 + 350 196 + 324 (Checks) Space 106 + 196 + 40 342 (Checks) Thus the global energy budget model shows necessary balance. 8