Energy and Environment 25 PDF
Document Details
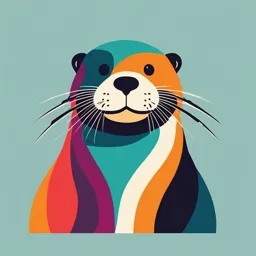
Uploaded by WorthPiccoloTrumpet
Department of Mechanical Engineering
Steve Tsike
Tags
Summary
This document explores the relationship between energy consumption and environmental impact, discussing various energy sources and the thermodynamic principles behind energy conversion. It also looks at the impact of energy use on ecosystems and human health, touching on related international conventions.
Full Transcript
STEVE TSIKE ENERGY AND THE ENVIRONMENT INTRODUCTION Modern societies of today consumption substantial amount of fossil fuels, and to some extent nuclear energy in order to meet the growing demand for energy. This enormous amount of energy use and its concentration in mostly the urban areas of bo...
STEVE TSIKE ENERGY AND THE ENVIRONMENT INTRODUCTION Modern societies of today consumption substantial amount of fossil fuels, and to some extent nuclear energy in order to meet the growing demand for energy. This enormous amount of energy use and its concentration in mostly the urban areas of both industrialized and developing nations has caused tremendous adverse impact on the biophysical environmental such as air and water pollution, and land degradation. This has affected many land-dependent ecosystems on a local and regional scale, as well as adverse health effects in human populations. Today, we are approaching the limit of the amount of land for agricultural purposes, only more intensive use of land can provide food for current and the increasing future world population. The international community is doing all it can to limit the damage to human health and natural ecosystems that attend these current problems and to forestall the development of even more severe ones in the future. Energy, Uses and Sources In modern industrial societies energy is consumed in providing food, clothing, shelter, transportation, communication, lighting, materials, and numerous services for the entire population. The first law of thermodynamics in physics states that energy cannot be destroyed, but can be transformed from one form to another. Chemical energy is released when fuel burns in the air causes the rearrangement of fuel and oxygen atoms to form combustion products, and this energy is transferred to the hot combustion product molecules. STEVE TSIKE ENERGY AND THE ENVIRONMENT Economic sectors and Energy Use It is customary to divide energy usage among four sectors of economic activity: 1. Industrial (manufacturing, material production, agriculture, resource recovery), 2. Transportation (cars, trucks, trains, airplanes, pipelines and ships), 3. Commercial (services) 4. Residential (homes). Except for renewable energy sources (including hydropower), the main global sources of energy are fossil and nuclear fuels. Ghana’s electricity generation had over the years been dominated by hydro assets; however, in recent years hydro has been complemented by fossil fuel generation to meet rapidly growing demand. Total installed capacity to date is about 2,703.5MW. 50 percent of installed capacity comes from hydropower installations, with the rest coming from thermal plants burning Natural Gas (NG), Light Crude Oil (LCO), or diesel. The generating units are owned and operated primarily by the Volta River Authority (VRA); however, some Independent Power producers (IPPs) such as Sunon Asogli. Aboadze T2 (TICo) and OSONOR (CENIT) have entered the market Environment The Oxford dictionary: defines environment as “the set of circumstances or conditions in which a person or community lives, works, develops, etc, or a thing exists or operates; the external conditions affecting the life of a plant or animal”. The Merriam Webster Collegiate dictionary defines the environment as “The complex of physical, chemical, and biotic factors (as climate, soil, and living things) that act upon an organism or an ecological community and ultimately determine its form and survival The adverse effects of human activities on the natural would include air and water pollution, land degradation by the by-products of industrial activity, permanent loss of natural species of plants and animals by changes in land and water usage and human predation, and, more recently, growing indications that the global climate was changing because of the anthropogenic emissions of so-called greenhouse gases. The degradation of the environment from human activities results from effects such as photochemical smog, acid rain, acidification of forest soils, contamination of marine sediments with municipal waste sludge, and poisoning of aquifers with drainage from toxic waste dump STEVE TSIKE ENERGY AND THE ENVIRONMENT Some United Nations Protocols , Treaties and Conventions on the Environment 1) Convention on long-range transboundary air pollution. Geneva, 13 November 1979 2) Vienna Convention for the Protection of the Ozone Layer. Vienna, 22 March 1985 3) Basel Convention on the Control of Transboundary Movements of Hazardous Wastes and their Disposal. Basel, 22 March 1989 4) Convention on Environmental Impact Assessment in a Transboundary Context. Espoo, Finland, 25 February 1991 5) Convention on the Protection and Use of Transboundary Watercourses and International Lakes. Helsinki, 17 March 1992 Convention on the Transboundary Effects of Industrial Accidents. Helsinki, 17 March 1992 6) United Nations Framework Convention on Climate Change. New York, 9 May 1992, 7) Kyoto Protocol to the United Nations Framework Convention on Climate Change. Kyoto, 11 December 1997 8) Convention on Biological Diversity. Rio de Janeiro, 5 June 1992, 9) United Nations Convention to Combat Desertification in those Countries Experiencing Serious Drought and/or Desertification, particularly in Africa. Paris, 14 October 1994, 10) Rotterdam Convention on the Prior Informed Consent Procedure for Certain Hazardous Chemicals and Pesticides in International Trade. Rotterdam, 10 September 1998 11) Stockholm Convention on Persistent Organic Pollutants. Stockholm, 22 May 2001 12) The Montreal Protocol on Substances That Deplete the Ozone Layer, 1988 STEVE TSIKE ENERGY AND THE ENVIRONMENT Thermodynamic Principles Of Energy Conversion Thermodynamics, as a subject, is central to the study of energy conversion, utilization, and the development of related technologies. The subject of thermodynamics provides an essential foundation for dealing with energy conversion processes and systems, as it introduces the thermodynamic properties of matter used to characterize energy systems, as well as helping in the development of a theoretical framework for predicting an energy system’s performance. The study of thermodynamics deals with how energy is transferred in natural processes, that is, it deals with the rate of flow of and the transformation of energy from one form or quality to another. Organisms are highly organized both structurally and metabolically, thus, constant care and maintenance is required to keep up this organization, and constant supply of energy is required to maintain these processes. Every time some energy is used by cells to do work, some of that energy is dissipated or lost as heat. If cellular energy supply are interrupted or depleted the result-sooner or later- is death. 2.1. Energy Sources and Conversion STEVE TSIKE ENERGY AND THE ENVIRONMENT Figure 2.1 Energy sources and conversion 2.1.1. Laws of Thermodynamics 2.1.1.1. Zeroth Law of Thermodynamics The Zeroth Law of Thermodynamics: If two systems are in thermal equilibrium with a third, then they are in thermal equilibrium with each other. This law is the basis of temperature measurement. The law is important for the mathematical formulation of thermodynamics or more precisely for stating the mathematical definition of temperature. This law is mostly used to compare temperatures of different objects. If we want to measure the accurate temperature, a reference body is required and a certain characteristic of that body which changes with temperature. The change in that characteristic may be taken as an indication of a change of temperature. That selected characteristic is known as thermodynamic property. The most common application of the zeroth law of thermodynamics can be seen in thermometers STEVE TSIKE ENERGY AND THE ENVIRONMENT 2.1.1.2. First of Thermodynamics Law The change in internal energy of a closed system is equal to the heat added to the system (or absorbed from the environment) minus the work done by the system (or on the environment). This law is a consequence of conservation of energy. While attempting to transform heat into work with full efficacy, we quickly learned that always some heat would escape into the surrounding environment as wasted energy (recall that energy cannot be destroyed). This wasted energy can never be fully converted into anything useful. In any process, “the total change in energy of a system, ∆E, is equal to the sum of the heat absorbed (q), and the work, (w) done on the system” or ∆E = q + w (1) The first law of thermodynamics asserts that energy is conserved and that heat flow is a form of energy transfer. For homogeneous systems, with a well-defined temperature and pressure, a commonly used corollary of the first law is that, for a system subject only to pressure forces and heat transfer (e.g., a cylinder-full of gas), the differential change in the internal energy of the system (with a gain in energy signified by a positive quantity) is given as: (2) where T = temperature, S = Entropy, P = pressure, and V = Volume 2.1.1.3. Second Law of Thermodynamics It is impossible to construct an engine which, operating in a cycle, will produce no other effect than the extraction of heat from a single heat reservoir and the performance of an equal amount of work. It imposes a limitation on energy transformations other than that imposed by the first law. [The second law of thermodynamics states that with each successive energy transfer or transformation in a system, less energy is available to do work] STEVE TSIKE ENERGY AND THE ENVIRONMENT 2.1.2. Entropy and Second Law of Thermodynamics The second law of thermodynamics expresses the relationship between entropy change and spontaneity. The law states that, “in a spontaneous process, there is a net increase in entropy, taking into accounts both system and its surroundings” [Entropy of a system is a measure of the randomness that exists in the system]. This 2nd Law means that heat cannot be converted 100% to work. When heat is converted to work, there will always be losses. ∆Suniverse = (∆Ssystem + ∆Ssurrounding)> 0 spontaneous process (3) “The second law states that “heat flow is naturally from regions of higher temperature to regions of lower temperature, but that it will not flow naturally the other way”. Heat can be made to flow from a colder region to a hotter region, which is exactly what happens in an air conditioner, but heat only does this when it is forced. On the other hand, heat flows from hot to cold spontaneously. Entropy is an indicator of the temperature of energy. A given amount of thermal energy has low entropy when it is at high temperature, and the same amount of energy has higher entropy when it is at lower temperature. Heat is energy, and with energy, size matters, with temperature, it does not. The radiant energy that arrives at Earth from the Sun at a temperature of 6000 K is a very low-entropy form of heat. ηthermal=1−Tc/T. H (4) ELECTRIC ENERGY PRODUCTION Most of world’s electric power is generated in large power plants where fossil or nuclear fuel provides the heat needed to generate mechanical energy in a steam turbine, which is then converted to electrical power in the electric generator coupled to it, or alternator, as 60-cycle synchronous alternating current (AC) electricity. In transmitting the power to distant consumers, the voltage is increased by electrical transformers and the power is merged into a network of high-voltage transmission lines that joins the outputs of many power plants to supply the myriads STEVE TSIKE ENERGY AND THE ENVIRONMENT of end-users who are connected to the transmissions lines by a network of distribution lines at lower voltage More recently, electric power is being generated in smaller plants, often employing as turbine engines, either alone or in combination with steam turbines the mechanical power source. 3.1. Sources of Electric Power Thermal and non-thermal sources of energy. Thermal Sources: the sun (solar thermal), burning fossil fuels like coal, oil, and natural gas, geothermal heat from the Earth's core, heat from a fire, a hot cup of coffee, an operating oven, and energy from biomass (organic waste). Non-thernal Sources: light energy, sound energy, electrical energy, mechanical energy (like movement), gravitational energy, magnetic fields, and radiation like X-rays or radio waves; essentially, any form of energy that isn't directly related to heat or temperature changes. hydropower, wave energy, solar, wind, Transmission of Electric Power.A gas or steam turbine powers an electric generator supplying alternating current to a transformer that steps up the line voltage to a high value in the transmission line, usually 60–500 kilovolts. After transmission from the power plant to the vicinity of load centers, a step-down transformer reduces the voltage to a lower value, 12–35 kilovolts, for the distribution system that delivers power to the end-users. For residential use, a further reduction to 120–240 volts is required Electric power is transmitted via electric cable consisting of two wires that conduct electrical current at different electric potentials. In a direct current (DC) system the electric current in each wire flows in one direction only. On the other hand, in an alternating current (AC) system, where the potential difference and current are sinusoidal functions of time, the currents in each wire reverse direction every half-ycle STEVE TSIKE ENERGY AND THE ENVIRONMENT Turbine Generator Step-up Step Down Load Transformer Transformer Power plant Transmission Line Distribution 3.3. Storage of Electric Power The electric power must be supplied at the same rate that it is being utilized by the utility’s customers. Although there is some energy temporarily stored (and removed) each halfcycle in the transmission and distribution lines and transformers, it is not available for supplying power when demand exceeds supply during a sustained period Ways of storing electric power include: 1) Electrostatic energy storage: Uses capacitors for storage 2) Magnetic energy storage: Uses magnetic inductors to store electric power 3) Electrochemical energy storage: Using battery for electric power storage 4) Mechanical energy storage: The common form of storing energy for use in electric utility systems is that of pumped hydropower. It consists of a normal hydroelectric plant that is supplied with water impounded behind a dam at high elevation and discharging to a body of water at a lower elevation through a turbine that drives an electric generator 3.4. FOSSIL-FUEL POWER PLANT In this chapter we describe the workings of fossil fueled power plants and their components: fuel storage and preparation, burner, boiler, turbine, condenser, and generator. We focus in particular on emission control technologies that power plants are required by law in most countries to install in order to safeguard public health and the environment 3.4.1. Fossil-Fueled Power Plant Components In a fossil-fueled power plant the chemical energy inherent in the fossil fuel is converted first to raise the enthalpy of the combustion gases; that enthalpy is transferred by convection and radiation to a working fluid (usually, water/steam); the enthalpy of the working fluid is converted to mechanical energy in a turbine; and finally the mechanical energy of the turbine shaft is converted to electrical energy in a generator. STEVE TSIKE ENERGY AND THE ENVIRONMENT The major components of a fossil fueled power plant are as follows: Fuel storage and preparation Burner Boiler Steam turbine Gas turbine Condenser Cooling tower Generator Emission control 3.4.1.1. Mechanism of Converting Coal to Electricity Coal is first milled to a fine powder, which increases the surface area and allows it to burn more quickly. The pulverized or powdered coal is blown into the combustion chamber of a boiler where it is burnt at high temperature (see diagram below). The hot gases and heat energy produced converts water in tubes or pipes lining the boiler into steam. STEVE TSIKE ENERGY AND THE ENVIRONMENT Figure 3.1 Typical Coal fuel power plant Source: Google images The high pressure steam is passed into a turbine containing thousands of propeller-like blades. The steam pushes these blades causing the turbine shaft to rotate at high speed. A generator is mounted at one end of the turbine shaft and consists of carefully wound wire coils. Electricity is generated when these are rapidly rotated in a strong magnetic field. After passing through the turbine, the steam is condensed and returned to the boiler to be heated once again. The electricity generated is transformed into the higher voltages (up to 400,000 volts) used for economic, efficient transmission via power line grids. When it nears the point of consumption, such as our homes the electricity is transformed down to the safer 100-250 voltage systems used in the domestic market. 3.4.1.2. Fuels Used in Thermal Plant STEVE TSIKE ENERGY AND THE ENVIRONMENT Most modern steam power plants are fire by pulverized coal. The raw coal from the stockpile is delivered on a conveyor belt directly to 1. a Rotating Ring mill 2. a Rotating Hammer mill. 3. a Rotating ball mill to pulverize the coal into fine powder, less than 1mm in size. The pulverized coal is stored in large vertical silos from where it is blown pneumatically into the burners at a rate specify by the load of the plant. In oil-fired power plants, oil is stored in large tanks (the“tankfarm”), to which oil is delivered either by pipeline, by railroad tankers, or by tanker ship or barge if the plant is located near navigable waters. The oil purchased from refineries is combusted in the burners, with specified sulfur, nitrogen, and ash content as well as other properties, such as viscosity and vapor pressure. In natural gas-fired power plants, gas is delivered to the power plant by pipeline at high pressure (compressed natural gas, CNG). Some gas-fired power plants use liquified natural gas (LNG). Liquified gas is often delivered by refrigerated tankers at about −164◦C, which is then stored in refrigerated tanks until used. 3.4.1.3. Role of Burners The burner provides a thorough mixing of the fuel and air completely combustion of fuel. a Pulverized coal particle or atomized oil droplet burns in a fraction of a second in the combustion chamber. After combustion, the coal particle or oil droplet leaves behind incombustible mineral matter called ash. In modern pulverized coal fired power plants, more than 90% of the mineral matter forms fly ash, which is blown out of the boiler and later captured in particle collectors. About 10% of the mineral matter falls to the bottom of the boiler as bottom ash. When the bottom of the boiler is filled with water, the bottom ash forms a wet sludge, which is sluiced away into an impoundment. Some of the fly ash, however, is deposited on the water pipes lining the boiler, forming a slag which impedes heat transfer. The slag needs to be removed from time to time by blowing steam jets against it or by mechanical scraping. STEVE TSIKE ENERGY AND THE ENVIRONMENT Pulverized coal requires 15–20% excess air; oil and gas 5–10%, as coal requires excess air for complete combustion. 3.4.1.3.1. Boiler Types The boiler is the central component of a fossil-fueled steam power plant. Most modern boilers are of the water wall type, in which the boiler walls are almost entirely constructed of vertical tubes that either carry feed water into the boiler or carry steam out of the boiler 3.4.1.3.1.1. Steam Turbine The antecedent of the steam turbine is the water wheel. Just as water pushes the blades of a water wheel, steam pushes the blades of a steam turbine. Considering the high pressure and temperature of the steam, that the turbine must be leak proof, the enormous centrifugal stresses on the shaft, and the fact that steam condenses to water while expanding in the turbine, thereby creating a two phase fluid flow, one gets an idea of the technological problems facing the designer and builder of steam turbines. 3.4.1.4. Types of Steam Turbines Three of the most common types of turbines are: i. Impulse turbine ii. Reaction turbine iii. Gas turbines 3.4.1.4.1. Impulse Turbine In an impulse turbine a jet of steam impinges on the blades of a turbine. The blades are symmetrical and have equal entrance and exit angles, usually 20◦. Steam coming from the super heater is then used to turn the rotor blades 3.4.1.4.2. Reaction Turbine STEVE TSIKE ENERGY AND THE ENVIRONMENT A reaction turbine consists of rows of fixed (stator) and moving (rotor) blades. The blades are shaped to form a converging nozzle [see Figure 5.3(b)]. Within the converging blades the steam pressure, density and temperature decline while converting its enthalpy to kinetic energy 3.4.1.4.3. Gas Turbine In a gas turbine plant where oil, natural gas, or synthesis gas may be used as a fuel, the hot combustion gases are directly used to drive a gas turbine, rather than transferring heat to steam and driving a steam turbine. This requires a different turbine, appropriate for the much higher temperature of the combustion gases and their different thermodynamic properties compared to steam 3.4.1.5. The role of the Condenser Steam turbines, after performing useful work as in all heat engine, the working fluid must eliminate heat to a cold body. Thermal plants reject a significant amount of heat into the environment. Between 1.5 and 3 times as much heat is rejected as the plant produces work in the form of electricity. For example, a 1000-MW electric power plant working at 25% efficiency rejects 3000 MW of heat to the environment, whereas one working at 40% efficiency rejects 1500 MW. Some of that heat is added to the environment by the condensing system in a steam cycle, and the rest is added by the discharge to the atmosphere of the hot flue gas vented through the smoke stack 3.4.1.5.1. Types of Condensers There are two types of condenser: direct contact and surface contact condensers. 3.4.1.5.1.1. Direct Contact Condenser The turbine exhaust passes an array of spray nozzles through which cooling water is sprayed, condensing the steam by direct contact. The warm condensate is pumped into a cooling tower where updrafting air cools the condensate that flows in tubes. 3.4.1.5.1.2. Surface Contact Condenser STEVE TSIKE ENERGY AND THE ENVIRONMENT.Surface contact condenser is essentially a shell-and-tube type heat exchanger. The turbine exhaust passes an array of tubes in which the cooling water flows. Because for large power plants very large volumes of steam need to be condensed, the contact surface area can reach 100,000 m2 for a 1000-MW plant 3.4.2. SOLAR ENERGY Solar energy is an important, clean, cheap and abundantly available renewable energy. It is received on Earth in cyclic, intermittent and dilute form with very low power density 0 to 1 kW/m2.Solar energy received on the ground level is affected by atmospheric clarity, degree of latitude, etc. For design purpose, the variation of available solar power, the optimum tilt angle of solar flat plate collectors, the location and orientation of the heliostats should be calculated. 3.4.2.1. Units of Solar Power and Solar Energy: In SI units, energy is expressed in Joule For solar energy calculations, the energy is measured as an hourly or monthly or yearly average and is expressed in terms of kJ/m2/day or kJ/m2/hour. Solar power is expressed in terms of W/m2 or kW/m2. 3.4.2.1.1. Essential subsystems in a Solar Energy Plant 3.4.2.1.1.1. Solar collector or Concentrator It receives solar rays and collects the energy. It may be of following types: a) Flat plate type without focusing b) Parabolic trough type with line focusing c) Paraboloid dish with central focusing d) Fresnel lens with centre focusing e) Heliostats with centre receiver focusing STEVE TSIKE ENERGY AND THE ENVIRONMENT 3.4.2.2. Energy Transport Medium: Substances such as water/ steam, liquid metal or gas are used to transport the thermal energy from the collector to the heat exchanger or thermal storage. In solar PV systems energy transport occurs in electrical form. 3.4.2.3. Energy Storage: Solar energy is not available continuously. So we need an energy storage medium for maintaining power supply during nights or cloudy periods. There are three major types of energy storage: a) Thermal energy storage; b) Battery storage; c) Pumped storage hydro-electric plant. 3.4.2.4. Energy conversion plant Thermal energy collected by solar collectors is used for producing steam, hot water, etc. Solar energy converted to thermal energy is fed to steam thermal or gas-thermal power plant. 3.4.2.5. Power conditioning, control and protection system Load requirements of electrical energy vary with time. The energy supply has certain specifications like voltage, current, frequency, power etc. The power conditioning unit performs several functions such as control, regulation, conditioning, protection, automation, etc 3.4.2.6. Alternative or standby power supply The backup may be obtained as power from electrical network or standby diesel generator. 3.4.2.7. Energy from the Sun The sun radiates about 3.8 x 1026 W of power in all the directions. Out of this about 1.7 x 1017 W is received by earth. The average solar radiation outside the earth’s atmosphere is 1.35 kW/m2 varying from 1.43 kW/m2 (in January) to 1.33 kW/m2 (in July). Solar thermal energy (STE) is a STEVE TSIKE ENERGY AND THE ENVIRONMENT form of energy and a technology for harnessing solar energy to generate thermal energy or electrical energy for use in industry, and in the residential and commercial sectors. The first installation of solar thermal energy equipment occurred in the Sahara Desert approximately in 1910 when a steam engine was run on steam produced by sunlight. Because liquid fuel engines were developed and found more convenient, the Sahara project was abandoned, only to be revisited several decades late. Solar thermal collectors are classified as low-, medium-, or high-temperature collectors. 1. Low-temperature collectors are flat plates generally used to heat swimming pools. 2. Medium-temperature collectors are also usually flat plates but are used for heating water or air for residential and commercial use. 3. High-temperature collectors concentrate sunlight using mirrors or lenses and are generally used for fulfilling heat requirements up to 300 deg C / 20 bar pressure in industries, and for electric power production. However, there is a term that used for both the applications. Concentrated Solar Thermal (CST) for fulfilling heat requirements in industries and Concentrated Solar Power (CSP) when the heat collected is used for power generation. CST and CSP are not replaceable in terms of application. 3.4.2.8 Solar Thermal Energy A solar thermal collector system gathers the heat from the solar radiation and gives it to the heat transport fluid. The heat-transport fluid receives the heat from the collector and delivers it to the thermal storage tank, boiler steam generator, heat exchanger etc. Thermal storage system stores heat for a few hours. The heat is released during cloudy hours and at night. Thermal-electric conversion system receives thermal energy and drives steam turbine generator or gas turbine generator. The electrical energy is supplied to the electrical load or to the AC grid. Applications of solar thermal energy systems range from simple solar cooker of 1 kW rating to complex solar central receiver thermal power plant of 200 MW rating Solar thermal energy is the most readily available source of energy. The Solar energy is most important kind of non-conventional source of energy which has been used since ancient times, but in a most primitive manner. The abundant solar energy available is suitable for harnessing for a number of applications. The application of solar thermal energy system ranges from solar STEVE TSIKE ENERGY AND THE ENVIRONMENT cooker of 1 kw to power plant of 200MW. These systems are grouped into low temperature ( 4 μm). The higher absorption of solar energy increase the temperature of absorber plate and working fluid. The top losses reduce and the efficiency of the collector increases. The selective surface should be able to withstand high temperature of 300-400oC, cost less, should not oxidize and be corrosive resistant. The property of material should not change with time (f) Inlet Temperature: With increase in inlet temperature of working fluid the losses increase to ambient. The high temperature fluid absorbed the less heat from absorber plate because of low temperature difference and increases the top loss coefficient. Therefore the efficiency of collector get reduced with rise in inlet temperature. (g) Dust on cover Plate: The efficiency of collector decreases with dust particles on the cover plate because the transmission radiation decreases by 1%. Frequent cleaning is required to get the maximum efficiency of collector. 3.4.2.12. Concentrating Collectors Concentrating collector is a device to collect solar energy with high intensity of solar radiation on the energy absorbing surface. Such collectors use optical system in the form of reflectors or refractors. These collectors are used for medium (100-300o C) and high-temperature (above 300oC) applications such as steam production for the generation of electricity. The high temperature is achieved at absorber because of reflecting arrangement provided for concentrating the radiation at required location using mirrors and lenses. These collectors are best suited to places having more number of clear days in a year. The area of the absorber is kept less than the aperture through which the radiation passes, to concentrate the solar flux. These collectors require tracking to follow the sun because of optical system. The tracking rate depends on the degree of concentration ratio and needs frequent adjustment for system having high concentration ratio. The efficiency of these collectors lies between 50-70%. The collectors need more maintenance than FPC because of its optical system. STEVE TSIKE ENERGY AND THE ENVIRONMENT The concentrating collectors are classified on the basis of reflector used; concentration ratio and tracking method adopted. 3.4.2.12,1, Types of Concentrating Collectors There are a number of different types of concentrating collectors available with varied degrees of efficiency. 3.4.2.12,1.1. Flat Plate collector (FPC) with Reflectors The mirrors are placed as reflecting surface to concentrate more radiations on FPC absorber. The fluid temperature is higher by 30oC than achieved in FPC. These collections utilize direct and diffuse radiation. 3.4.2.12,1.2. Lens Focusing Type The lenses are used to concentrate the radiation at its focus. To achieve high efficiency, the lower side of lenses is grooved so that radiation concentrates on a focus line. 3.4.2.12,1.3. Compound Parabolic Collectors Compound Parabolic Collectors are line focusing collectors which have two parabolic surfaces to concentrate the solar radiation on the absorber placed at the bottom. These collectors is tracking in nature with have high concentration ratio, constantly moving to track the sun. 3.4.2.12,1.4. Cylindrical Parabolic Collectors The troughs concentrate sunlight onto a receiver tube, placed along the focal line of the trough. The temperature at the absorber tube is obtained at nearly 400o C. The absorber in these collectors is moving to receive the reflected radiations by reflector, while the concentrators (trough) remains fixed. Because of its parabolic shape, it can focus the sun at 30 to 100 times its normal intensity (concentration ratio) on a receiver. The heat transfer medium carries the heat at one central place for further utilization. 3.4.2.12,1.5. Parabolic Dish Collector The collectors have mirror-like reflectors and an absorber at the focal point. These collectors are point focusing type. The concentrating ratio of these collectors is 100 and temperature of the receiver can reach up to 2000o C. These collectors have higher efficiency for converting solar STEVE TSIKE ENERGY AND THE ENVIRONMENT energy to electricity in the small-power plant. In some systems, a heat engine, such as a Stirling engine, is connected to the receiver to generate electricity. 3.4.2.12.2. Center Receiver Type (Solar Power Tower) These collectors are used to collect the large solar energy at one point, using between 100-10000 of flat tracking mirror scaled heliostats to reflect the solar energy to central receiver mounted on tower. The energy can be concentrated as much as 1,500 times the energy coming in from the sun. The concentrated energy is used to heat a fluid to produce steam. 3.4.2.12.2.1. Advantages of concentrating collector over flat collector 1. The size of the absorber can be reduced that gives high concentration ratio. 2. Thermal losses are less than FPC. However small losses occur in the concentrating collector because of its optical system as well as by reflection, absorption by mirrors and lenses. 3. The efficiency increases at high temperatures. 4. In these collectors the area intercepting the solar radiation is greater than the absorber area. 5. These collectors are used for high-temperature applications. 6. Reflectors can cost less per unit area than flat plate collectors 7. Focusing or concentrating systems can be used for electric power generation when not used for heating or cooling 8. Little or no anti freeze is required to protect the absorber in a concentrator system whereas the entire solar energy collection surface requires anti freeze protection in a flat plate collector 3.4.2.12.2.2. Disadvantages 1) Out of the beam and diffuse solar radiation components, only beam component is collected in case of focusing collectors because diffuse component cannot be reflected and is thus lost. 2) In some stationary reflecting systems it is necessary to have a small absorber to track the sun image; in others the reflector may have to be adjustable more than one position if year round operation is desired; in other words costly orienting systems have to be used to track the sun. STEVE TSIKE ENERGY AND THE ENVIRONMENT 3) Additional requirements of maintenance particular to retain the quality of reflecting surface against dirt, weather, oxidation etc. 4) Non –uniform flux on the absorber whereas flux in flat-plate collectors in uniform. 5) Additional optical losses such as reflectance loss and the intercept loss, so they introduce additional factors in energy balances. 6) High initial cost. 3.4.2.12.3. Solar Air Heaters Air stream is heated by the back side of the collector plate in flat plate collector. Fins attached to the plate increase the contact surface. The back side of the collector is heavily insulated with mineral wool or some other material. If the size of collector is large, a blower is used to draw air into the collector and transmit the hot air to dryer. 3.4.2.12.3.1. Applications of Solar air heaters 1. Heating buildings. 2. Drying agricultural produce and lumber. 3. Heating green houses. 4. For Air conditioning buildings 5. Heat sources for a heat engine such as a Stirling cycle. 3.4.2.12.3.2. Disadvantages: 1. Need of handling larger volumes of air than liquids due to low density of air as working substance. 2. Thermal capacity of the air is low. 3. They have relatively high fluid circulation costs (especially if the rock heat storage unit is not carefully designed) 4. They have relatively large volumes of storage (roughly three times as much volume as for water heat-storage) 5. They have a higher noise level. STEVE TSIKE ENERGY AND THE ENVIRONMENT 6. The system has difficulty of adding conventional absorption air-conditioners to air systems 7. The space is required for ducting. 3.4.2.12.4. Flat Plate Collector (FPC) Flat plate collector absorbs both beam and diffuse components of radiant energy. The absorber plate is a specially treated blackened metal surface. Sun rays striking the absorber plate are absorbed causing rise of temperature of transport fluid. Thermal insulation behind the absorber plate and transparent cover sheets (glass or plastic) prevent loss of heat to surroundings. 3.4.2.12.4.1. Applications of flat plate collector 1. Solar water heating systems for residence, hotels, industry. 2. Desalination plant for obtaining drinking water from sea water. 3. Solar cookers for domestic cooking 4. Drying applications. 5. Residence heating. 3.4.2.12.5. Paraboloid dish collectors Parabolic trough with line focusing reflecting surface provides concentration ratios from 30 to 50. Hence, temperature as high as 300oC can be attained. Light is focused on a central line of the parabolic trough. The pipe located along the centre line absorbs the heat and the working fluid is circulated trough the pipe. 3.4.2.12.6. Paraboloid Dish Collectors The beam radiation is reflected by paraboloid dish surface. The point focus is obtained with CR (above 1000) and temperatures around 1000oC. Low temperature collector 1. Medium temperature collector 2. High temperature collector STEVE TSIKE ENERGY AND THE ENVIRONMENT 3. Low temperature collector: 3.4.2.12.7. Low temperature collector Glazed solar collectors are designed primarily for space heating. They are building air through a solar air panel where the air is heated and then directed back into the building. These solar space building and only perform when the air in the solar collector is warmer than the building room temperature. Most glazed collectors are used in the residential sector. 3.4.2.12.8. Solar thermal collector Glazed solar collectors are designed primarily for space heating. They re-circulate building air through a solar air panel where the air is heated and then directed back into the building. These solar space heating systems require at least two penetrations into the building and only perform when the air in the solar collector is warmer than the building lazed collectors are used in the residential sector. 3.4.2.12.9. Unglazed, "transpired" air collector Unglazed solar collectors are primarily used to pre-heat make-up ventilation air in commercial, industrial and institutional buildings with a high ventilation load. They turn building walls or sections of walls into low cost, high performance, unglazed solar collectors. Heat conducts from the absorber surface to the thermal boundary layer of air 1 mm thick on the outside of the absorber and to air that passes behind the absorber. The boundary layer of air is drawn into a nearby perforation before the heat can escape by convection to the outside air. The heated air is then drawn from behind the absorber plate into the building's ventilation system. 3.4.2.12.10. A Trombe Wall A Trombe wall is a passive solar heating and ventilation system consisting of an air channel sandwiched between a window and a sun-facing thermal mass. During the ventilation cycle, sunlight stores heat in the thermal mass and warms the air channel causing circulation through vents at the top and bottom of the wall. During the heating cycle the Trombe wall radiates stored heat. 3.4.2.12.11. Solar Roof Ponds Solar roof ponds are unique solar heating and cooling systems consisting of a roof-mounted water bladder with a movable insulating cover. This system can control heat exchange between interior and exterior environments by covering and uncovering the bladder between night and day. When heating is a concern the bladder is uncovered during the day allowing sunlight to STEVE TSIKE ENERGY AND THE ENVIRONMENT warm the water bladder and store heat for evening use. When cooling is a concern the covered bladder draws heat from the building's interior during the day and is uncovered at night to radiate heat to the cooler atmosphere. The two main types of solar air panels are glazed and unglazed. 3.4.2.12.12. Medium temperature collector: 3.4.2.12.12.1. Solar drying Solar thermal energy can be useful for drying wood for construction and wood fuels such as wood chips for combustion. Solar is also used for food products such as fruits, grains, and fish. Crop drying by solar means is environmentally friendly as well as cost effective while improving the quality. The less money it takes to make a product, the less it can be sold for, pleasing both the buyers and the sellers. Technologies in solar drying include ultra low cost pumped transpired plate air collectors based on black fabrics. Solar thermal energy is helpful in the process of drying products such as wood chips and other forms of biomass by raising the temperature while allowing air to pass through and get rid of the moisture. 3.4.2.12.12.2. Solar Cookers Solar cookers use sunlight for cooking, drying and pasteurization. Solar cooking offsets fuel costs, reduces demand for fuel or firewood, and improves air quality by reducing or removing a source of smoke. 3.4.2.12.13. High Temperature Collector Where temperatures below about 95 °C are sufficient, as for space heating, flat-plate collectors of the non-concentrating type are generally used. Because of the relatively high heat losses through the glazing, flat plate collectors will not reach temperatures much above 200 °C even when the heat transfer fluid is stagnant. Such temperatures are too low for efficient conversion to electricity. The efficiency of heat engines increases with the temperature of the heat source. To achieve this in solar thermal energy plants, solar radiation is concentrated by mirrors or lenses to obtain higher temperatures – a technique called Concentrated Solar Power (CSP). The practical effect of high efficiency is to reduce the plant's collector size and total land use per unit power generated, reducing the environmental impacts of a power plant as well as its expense. STEVE TSIKE ENERGY AND THE ENVIRONMENT BIOMASS ENERGY ESOURCES Introduction Biomass is the stored energy in plant and animal tissues that can be used as fuel. It is considered to be among the most vital resources on the earth. Biomass, in addition to providing sustenance for plants and animals, is also used in the majority of human-made materials, including fabrics, medicines, chemicals, and construction materials. The use of biomass as a source of energy dates back to the discovery of fire, when wood was combusted for heat. This chapter will review biomass fuels and technologies that provide us with energy used in various forms, including for the fueling of cars, generating power, and constructing computer components. 4.1. Origins and Chemical Composition of Biomass Biomass is biological material derived from living, or recently living organisms. It most often refers to plants or plant-based materials which are specifically called lignocellulosic biomass. As an energy source, biomass can either be used directly via combustion to produce heat, or indirectly after converting it to various forms of biofuel. Conversion of biomass to biofuel can be achieved by different methods which are broadly classified into: thermal, chemical, and biochemical methods. Wood remains the largest biomass energy source to date; examples include forest residues (such as dead trees, branches and tree stumps), yard clippings, wood chips and even municipal solid waste. In the second sense, biomass includes plant or animal matter that can be converted into fibres or other industrial chemicals, including biofuels. Industrial biomass can be grown from numerous types of plants, including miscanthus, switchgrass, hemp, corn, poplar, willow, sorghum, sugarcane, bamboo, and a variety of tree species, ranging from eucalyptus to oil palm (palm oil). The chemical composition of biomass depends on the various types of tissues found among plant and animal species. In general, plant structure consists of about 25 percent lignin and 75 percent carbohydrates or sugars. The carbohydrates in plants consist of many sugar molecules that are linked together in long chains called polymers. Some of the carbohydrates contain significant amounts of cellulose and hemicellulose. Lignin is a non- sugar polymer that acts as the mortar in the building blocks of plants. It gives a plant its strength while acting as the “glue” that holds the cellulose fibers together. STEVE TSIKE ENERGY AND THE ENVIRONMENT 4.2. Energy Value of Biomass as a Potential Alternative Energy Source Biomass fuels, when processed, offer a wide diversity of fuel supply. These fuels can be produced and used to generate electricity through direct combustion in modern devices or be processed to produce a wide variety of liquid fuels such as ethanol, biodiesel, and other alcohol- containing fuels used in motor vehicles. Biomass energy undoubtedly can increase global economic development without contributing to the greenhouse effect because biomass does not contribute to the inversion layer in global warming. This is so because the net amount of CO2 produced from burning is recycled and absorbed by plants, therefore leading to biomass being considered a sustainable-energy resource. The potential for bioenergy extraction from biomass is enormous. With advances in applications of the technology, it is now possible to convert raw biomass into various forms of energy, including electricity, liquid or gaseous fuels, and processed solid fuels. This could result in significant social and economic benefits to the world. It is a well-known that the quality of life for very large majorities of the world’s population is directly related to the availability of one or another form of energy. It has been proven that improvements in infrastructure, health, social advancement, and jobs depend on the availability of dispersible energy. 4.3. Fuel Ethanol Production Ethanol is an alcohol produced by yeast from sugars. It is the same alcohol produced by yeast in beer, wine, and spirits. Fuel ethanol is ethanol that has been highly concentrated to remove water and blended with other compounds to render the alcohol undrinkable. Fuel ethanol can be used alone as a fuel, such as in Indy Racing League cars, or can be blended with gasoline and used as fuel. All cars and trucks on the road today can use gasoline/ethanol blends of up to 10% ethanol (90% gasoline), also called “E10.” Blends of up to 85% ethanol, also known as “E85,” can be used as transportation fuel by cars and trucks with slight modifications 4.3.1. Role of Yeast’s in Ethanol Production All ethanol production is based upon the activity of yeast (Saccharomyces cerevisiae), an important microorganism to humans. Through a process called “fermentation,” yeast eats simple sugars and produce carbon dioxide (CO2) and ethanol as waste products. For each pound of simple sugars, yeast can produce approximately ½ pound (0.15 gallons) of ethanol and an STEVE TSIKE ENERGY AND THE ENVIRONMENT equivalent amount of carbon dioxide. The value of corn as a feedstock for ethanol production is due to the large amount of carbohydrates, specifically starch, present in corn (Table 1). Starch can be rather easily processed to break it down into simple sugars, which can then be fed to yeast to produce ethanol. Modern ethanol production can produce approximately 2.7 gallons of fuel ethanol per bushel of corn. 4.3.2. Ethanol Production Using Corn as Feedstock The major steps in this process are: Milling Slurry and Liquefaction. Saccharification.... Fermentation.... Distillation.... Dehydration.... Ethanol Loadout.... Co-Products. 4.3.2.1. Milling The feedstock (corn) passes through a hammer mill, which grinds it into a fine powder called meal and then is conveyed into the plant. 4.3.2.2. Slurry and Liquefaction The corn meal is mixed with process water and alpha-amylase forming mash and then passed through a jet cooker where the starch is liquefied and sheared. Heat is applied at this stage to reduce bacteria levels in the mash as well as allow the alpha-amylase to enable liquefaction where the starch is broken down into complex sugars. 4.3.2.3. Saccharification. The mash from liquefaction is cooled through plate and frame exchangers and the secondary enzyme gluco-amylase is added to convert the complex sugars into fermentable sugars (simple sugars). STEVE TSIKE ENERGY AND THE ENVIRONMENT 4.3.2.4. Fermentation Yeast is added to the mash to ferment the simple sugars into ethanol, heat and carbon dioxide. In our batch process, the mash stays in one fermenter for about 50-60 hours before the distillation process is started. 4.3.2.5. Distillation. The fermented mash, now called beer, contains about 14% ethanol, plus all the non-fermentable solids from the corn meal and yeast cells. The mash is pumped to our continuous flow, multi- column distillation system where the ethanol is removed from the solids and the water. The ethanol leaves the top of the final column at about 190 proof, which is the azeotropic point of water (point where mixed liquids being distillated have constant boiling point and composition) and ethanol. The residual mash, called stillage, is transferred from the base of the column to the co-product processing area. 4.3.2.6. Dehydration. The ethanol from the top of the column passes through a dehydration system where the remaining water will be removed. Our ethanol plant uses a molecular sieve to capture the last bit of water in the ethanol. The alcohol product at this stage is called anhydrous ethanol (pure, without water) and is approximately 200 proof. The 200 proof ethanol is then pumped into storage tanks to be loaded out. The corn is grounded and cooked, liquefied and then saccharified. Saccharification is the hydrolysis of polysaccharides to soluble sugars. Malt made from barley is used as a source of β- amylase to break down starch into the disaccharide maltose, which can be used by yeast to produce beer. Other amylase enzymes may convert starch to glucose or to oligosaccharides STEVE TSIKE ENERGY AND THE ENVIRONMENT Commercial production of fuel ethanol involves breaking down the starch present in corn into simple sugars (glucose), feeding these sugars to yeast (fermentation), and then recovering the main product (ethanol) and byproducts (e.g., animal feed). Two major industrial methods for producing fuel ethanol are in use: wet milling and dry grind. \ 4.3.2.1. Wet Grinding Wet milling is called “wet” because the first step in the process involves soaking the grain in water (steeping) to soften the grain and make it easier to separate (fractionate) the various components of the corn kernel. Fractionation separates the starch, fiber, and germ, to allow these various components to be processed separately to make a variety of products such as fuel ethanol, high fructose corn syrup (HFCS), biodegradable plastics, food additives such as citric acid, corn oil (cooking oil), and livestock feed. STEVE TSIKE ENERGY AND THE ENVIRONMENT 4.3.2.2.2. Dry-Grind Ethanol Process In the dry-grind ethanol process, the whole grain is processed without first steeping, and the residual components are separated at the end of the process. There are five major steps in the dry-grind method of ethanol production. 4.3.2.2.3. Dry-Grind ethanol Processing steps 1. Milling 2. Liquefaction 3. Saccharification 4. Fermentation 5. Distillation and recovery 4.3.2.2.4. Milling Milling involves processing corn through a hammer mill (with screens between 3.2 to 4.0 mm) to produce a corn flour. This whole corn flour is slurried with water, and heat-stable enzyme (ά- amylase) is added 4.3.2.2.5. Liquefaction This slurry is cooked, also known as “liquefaction.” Liquefaction is accomplished using jet- cookers that inject steam into the corn flour slurry to cook it at temperatures above 100°C (212°F). The heat and mechanical shear of the cooking process break apart the starch granules present in the kernel endosperm, and the enzymes break down the starch polymer into small fragments. The cooked corn mash is then allowed to cool to 80-90°C (175-195°F), additional enzyme (a-amylase) is added, and the slurry is allowed to continue liquefying for at least 30 minutes. 4.3.2.2.6. Saccharification After liquefaction, the slurry, now called “corn mash,” is cooled to approximately 30°C (86°F), and a second enzyme (gluco-amylase) is added. Gluco-amylase completes the breakdown of the starch into simple sugar (glucose). This step, called “Saccharification,” often occurs while the STEVE TSIKE ENERGY AND THE ENVIRONMENT mash is filling the fermentor in preparation for the next step (fermentation) and continues throughout the next step. 4.3.2.2.7. Fermentation In the fermentation step, yeast grown in seed tanks are added to the corn mash to begin the process of converting the simple sugars to ethanol. The other components of the corn kernel (protein, oil, etc.) remain largely unchanged during the fermentation process. In most dry-grind ethanol plants, the fermentation process occurs in batches. A fermentation tank is filled, and the batch ferments completed before the tank is drained and refilled with a new batch. The up-stream processes (grinding, liquefaction, and saccharification) and downstream processes (distillation and recovery) occur continuously (grain is continuously processed through the equipment). Carbon dioxide is also produced during fermentation. Usually, the carbon dioxide is not recovered and is released from the fermenters to the atmosphere. If recovered, this carbon dioxide can be compressed and sold for carbonation of soft drinks or frozen into dry ice for cold product storage and transportation. After the fermentation is complete, the fermented corn mash is emptied from the fermentor into a beer well. The beer well stores the fermented beer between batches and supplies a continuous stream of material to the ethanol recovery steps, including distillation 3.3.2.2.3. Distillation and Recovery After fermentation, the liquid portion of the slurry has 8-12% ethanol by weight. Because ethanol boils at a lower temperature than water (780C) does, the ethanol can be separated by a process called “distillation.” Conventional distillation/rectification systems can produce ethanol at 92- 95% purity. The residual water is then removed using molecular sieves that selectively adsorb the water from an ethanol/water vapor mixture, resulting in nearly pure ethanol (>99%) The residual water and corn solids that remain after the distillation process are called “stillage.” This whole stillage is then centrifuged to separate the liquid (thin stillage) from the solid fragments of the kernel (wet cake or distillers’ grains). Some of the thin stillage (backset) is recycled to the beginning of the dry-grind process to conserve the water used by the facility. The remaining thin stillage passes through evaporators to remove a significant portion of the water to produce thickened syrup.. STEVE TSIKE ENERGY AND THE ENVIRONMENT 4.4. Bioenergy Conversion Technologies There are five fundamental forms of biomass energy use. 1. The "traditional domestic" use in developing countries (fuelwood, charcoal and agricultural residues) for household cooking (e.g. the "three stone fire"), lighting and space- heating (5% and 15%. efficient) 2. The "traditional industrial" use of biomass for cottage industrial processing e.g. bricks & tiles, etc, up to about 15% efficiency. 3. The "Modern industrial." use of biomass with technologically advanced thermal conversion technologies with conversion efficiency between 30 and 55%. 4. Newer "chemical conversion" technologies ("fuel cell") which are capable of by-passing the entropy-dictated Carnot limit which describes the maximum theoretical conversion efficiencies of thermal units. 5. The"biological conversion" techniques, including anaerobic digestion for biogas production and fermentation for alcohol. In general, biomass-to-energy conversion technologies have to deal with a feedstock which can be highly variable in mass and energy density, size, moisture content, and intermittent supply. 4.5. Direct Combustion Processes. Feedstocks used are often residues such as woodchips, sawdust, bark, hogfuel, black liquor, bagasse, straw, municipal solid waste (MSW), and wastes from the food industry. Direct combustion furnaces can be divided into two broad categories and are used for producing either direct heat or steam. Dutch ovens, spreader-stoker and fuel cell furnaces employ two-stages. The first stage is for drying and possible partial gasification, and the second for complete combustion. More advanced versions of these systems use rotating or vibrating grates to facilitate ash removal, with some requiring water cooling. The second group, include suspension and fluidised bed furnaces which are generally used with fine particle biomass feedstocks and liquids. In suspension furnaces the particles are burnt whilst being kept in suspension by the injection of turbulent preheated air which may already have the biomass particles mixed in it. In fluidised bed combustors, a boiling bed of pre-heated sand (at temperatures of 500 to 900°C) provides the combustion medium, into which the biomass fuel is either dropped (if it is dense enough to sink STEVE TSIKE ENERGY AND THE ENVIRONMENT into the boiling sand) or injected if particulate or fluid. These systems obviate the need for grates, but require methods of preheating the air or sand, and may require water cooled injection systems for less bulky biomass feedstocks and liquids. 4.5.1. Thermal Co-firing. A modern practice which has allowed biomass feedstocks an early and cheap entry point into the energy market is the practice of co-firing a fossil-fuel (usually coal) with a biomass feedstock. Co-firing has a number of advantages, especially where electricity production is an output. Firstly, where the conversion facility is situated near an agro-industrial or forestry product processing plant, large quantities of low cost biomass residues are available. These residues can represent a low cost fuel feedstock although there may be other opportunity costs. Secondly, it is now widely accepted that fossil-fuel power plants are usually highly polluting in terms of sulphur, CO2 and other GHGs. Using the existing equipment, perhaps with some modifications, and co- firing with biomass may represent a cost-effective means for meeting more stringent emissions targets. Biomass fuel's low sulphur and nitrogen (relative to coal) content and nearly zero net CO2 emission levels allows biomass to offset the higher sulphur and carbon contents of the fossil fuel. Thirdly, if an agro-industrial or forestry processing plant wishes to make more efficient use of the residues generated by co-producing electricity, but has a highly seasonal component to its operating schedule, co-firing with a fossil fuel may allow the economic generation of electricity all year round. Agro-industrial processors such as the sugarcane sugar industry can produce large amounts of electricity during the harvesting and processing season, however, during the off- season the plant will remain idle. 4.5.1.1. Drawbacks of Co-Firing This has two drawbacks, firstly, it is an inefficient use of equipment which has a limited life-time, and secondly, electrical distribution utilities will not pay the full premium for electrical supplies which can't be relied on for year round production. In other words the distribution utility needs to guarantee year round supply and may therefore, have to invest in its own production capacity to cover the off-season gap in supply with associated costs in equipment and fuel. STEVE TSIKE ENERGY AND THE ENVIRONMENT 4.6. Thermochemical Processes Pyrolysis. Carbonisation. Gasification. Catalytic Liquefaction. These processes do not necessarily produce useful energy directly, but under controlled temperature and oxygen conditions are used to convert the original biomass feedstock into more convenient forms of energy carriers, such as producer gas, oils or methanol. These carriers are either more energy dense and therefore reduce transport costs, or have more predictable and convenient combustion characteristics allowing them to be used in internal combustion engines and gas turbines. 4.6.1. Pyrolysis The biomass feedstock is subjected to high temperatures at low oxygen levels, thus inhibiting complete combustion, and may be carried out under pressure. Biomass is degraded to single carbon molecules (CH4 and CO) and H2 producing a gaseous mixture called "producer gas." Carbon dioxide may be produced as well, but under the pyrolytic conditions of the reactor it is reduced back to CO and H2O; this water further aids the reaction. Liquid phase products result from temperatures which are too low to crack all the long chain carbon molecules so resulting in the production of tars, oils, methanol, acetone, etc. Once all the volatiles have been driven off, the residual biomass is in the form of char which is virtually pure carbon. Pyrolysis has received attention recently for the production of liquid fuels from cellulosic feedstocks by "fast" and "flash" pyrolysis in which the biomass has a short residence time in the reactor. A more detailed understanding of the physical and chemical properties governing the pyrolytic reactions has allowed the optimisation of reactor conditions necessary for these types of pyrolysis. Further work is now concentrating on the use of high pressure reactor conditions to produce hydrogen and on low pressure catalytic techniques (requiring zeolites) for alcohol production from the pyrolytic oil. STEVE TSIKE ENERGY AND THE ENVIRONMENT 4.6.2. Gasification. Gasification is a thermochemical process that converts any carbonaceous (carbon-based) raw material such as coal into fuel gas, also known as synthesis gas (syngas). Gasification occurs in a high temperature/pressure vessel called gasifier, where low levels of oxygen (or air) and steam are directly contacted with the coal or other feed material causing a series of chemical reactions to occur that convert the feed to syngas and ash/slag (mineral residues). Syngas is an intermediate product in the production of synthetic natural gas. Syngas is composed primarily of the colorless, odorless, highly flammable gases carbon monoxide (CO) and hydrogen (H2), syngas has a variety of uses. The syngas can be further converted (or shifted) to nothing but hydrogen and carbon dioxide (CO2) by adding steam and reacting over a catalyst in a water-gas-shift reactor. When hydrogen is burned, it creates nothing but heat and water, resulting in the ability to create electricity with no carbon dioxide in the exhaust gases. Furthermore, hydrogen made from coal or other solid fuels can be used to refine oil, or to make products such as ammonia and fertilizer. More importantly, hydrogen enriched syngas can be used to make gasoline and diesel fuel. Poly-generation plants that produce multiple products are uniquely possible with gasification technologies. Carbon dioxide can be efficiently captured from syngas, preventing its greenhouse gas emission to the atmosphere and enabling its utilization (such as for Enhanced Oil Recovery) or safe storage 4.6.2.1. Effect of High Temperature and a controlled Environment High temperatures and a controlled environment leads to virtually all the raw material being converted to gas. This takes place in two stages. In the first stage, the biomass is partially STEVE TSIKE ENERGY AND THE ENVIRONMENT combusted to form producer gas and charcoal. In the second stage, the C02 and H2O produced in the first stage is chemically reduced by the charcoal, forming CO and H2. The composition of the gas is 18 to 20% H2, an equal portion of CO, 2 to 3% CH4, 8 to 10% CO2, and the rest nitrogen. These stages are spatially separated in the gasifier, with gasifier design very much dependant on the feedstock characteristics. Gasification requires temperatures of about 800°C and is carried out in closed top or open top gasifiers. These gasifiers can be operated at atmospheric pressure or higher. The energy density of the gas is generally less than 5.6 MJ/m3, which is low in comparison to natural gas at 38 MJ/m3 , providing only 60% the power rating of diesel when used in a modified diesel engine. A major future role is envisaged for electricity production from biomass plantations and agricultural residues using large scale gasifiers with direct coupling to gas turbines. Modern coal electrical plants have efficiencies of about 35% or less. Combined Heat and Power systems could eventually provide energy at efficiencies of between 50% to 80%. The use of low-grade feedstocks combined with high conversion efficiencies makes these systems economically competitive with cheap coal-based plants and energetically competitive with natural gas-based plants. 4.6.3. Carbonisation. This is the traditional methods of charcoal production which centers on the use of earth mounds or covered pits into which the wood is piled. The conversion efficiency of these traditional technique is very low. On a weight basis, it is estimated that the wood to charcoal conversion rate for this method ranges from 6 to 12 tonnes of wood per tonne of charcoal. During carbonisation most of the volatile components of the wood such as hydrogen and oxygen are eliminated allowing carbon to accumulate. The wood undergoes a number of physico- chemical changes as the temperature rises. Between 100 and 170°C most of the water is evaporated; between 170°C and 270°C gases develop containing condensible vapours, CO and CO2. These condensible vapours (long chain carbon molecules) form pyrolysis oil, which can then be used for the production of chemicals or as a fuel after cooling and scrubbing. Between 270°C and 280°C an exothermic reaction develops which can be detected by the spontaneous generation of heat. STEVE TSIKE ENERGY AND THE ENVIRONMENT 4.6.3.1. Types of Carbonisation ( charcoal making) Methods There are three basic types of charcoal-making: a) internally heated (by controlled combustion of the raw material), b) externally heated (using fuelwood or fossil fuels), and c) hot circulating gas (retort or converter gas, used for the production of chemicals). Internally heated charcoal kilns are the most common form of charcoal kiln. It is estimated that 10 to 20% of the wood (by weight) is sacrificed, a further 60% (by weight) is lost through the conversion to, and release of, gases to the atmosphere from these kilns. Externally heated reactors allow oxygen to be completely excluded, and thus provide better quality charcoal on a larger scale. They do, however, require the use of an external fuel source, which may be provided from the "producer gas" once pyrolysis is initiated. Recirculating heated gas systems offer the potential to generate large quantities of charcoal and associated by- products, but are presently limited by high investment costs for large scale plant. 4.6.4. Hydrothermal (Catalytic) Liquefaction (HTL) Hydrothermal liquefaction (HTL) is a thermal depolymerization (break up of polymers) process used to convert wet biomass into crude-like oil (bio-oil or biocrude), under moderate temperature and high pressure. The bio-oil has high energy density with a lower heating value of 33.8-36.9 MJ/kg and 5-20 wt % oxygen and renewable chemicals. A homogeneous and/or heterogeneous catalysts is required to improve the quality of products and yields. Carbon and hydrogen of an organic material, such as biomass, peat or low-ranked coals (lignite) are thermo-chemically converted into hydrophobic compounds with low viscosity and high solubility. Depending on the processing conditions, the fuel can be used as produced for heavy engines, including marine and rail or upgraded to transportation fuels, such as diesel, gasoline or jet-fuels. The usual methods for direct liquefaction processing are pyrolysis, solvent extraction or hydrogenation. These processes can all be enhanced by the use of catalysts (for example, catalytic pyrolysis or catalytic hydropyrolysis. Catalysis is necessary because there are many STEVE TSIKE ENERGY AND THE ENVIRONMENT reactions possible at the conditions used for liquefaction and only certain ones are desirable, such as bond cleavage and chain termination. This technology has the potential to produce higher quality products of greater energy density. Most catalytic liquefaction processes for producing low-sulphur liquid fuel from coal use large amounts of hydrogen which will have to be produced at high cost. 4.7. Biochemical Processes. Anaerobic Fermentation. Methane Production in Landfills. Ethanol Fermentation. Biodiesel. The use of micro-organisms for the production of ethanol is an ancient art. However, in more recent times such organisms have become regarded as biochemical "factories" for the treatment and conversion of most forms of human generated organic waste. Microbial engineering has encouraged the use of fermentation technologies (aerobic and anaerobic) for use in the production of energy (biogas) and fertiliser, and for the use in the removal of unwanted products from water and waste streams. 4.7.1. Anaerobic Fermentation. Anaerobic reactors are generally used for the production of methane rich biogas from manure (human and animal) and crop residues. They utilise mixed methanogenic bacterial cultures which are characterised by defined optimal temperature ranges for growth. These mixed cultures allow digesters to be operated over a wide temperature range i.e. above 0°C up to 60°C. When STEVE TSIKE ENERGY AND THE ENVIRONMENT functioning well, the bacteria convert about 90% of the feedstock energy content into biogas (containing about 55% methane), which is a readily useable energy source for cooking and lighting. The sludge produced after the manure has passed through the digester is non-toxic and odourless. Also, it has lost relatively little of its nitrogen or other nutrients during the digestion process thus, making a good fertiliser. In fact, compared to cattle manure left to dry in the field the digester sludge has a higher nitrogen content; many of the nitrogen compounds in fresh manure become volatised whilst drying in the sun. On the other hand, in the digested sludge little of the nitrogen is volatised, and some of the nitrogen is converted into urea. Urea is more readily accessible by plants than many of the nitrogen compounds found in dung, and thus the fertiliser value of the sludge may actually be higher than that of fresh dung. Anaerobic digestion produces energy (biogas) and fertiliser as well as improved health benefits. Health benefits primarily arise from the cleaner combustion products of biogas as opposed to other biomass or fossil fuels which may be used in the domestic environment. Reliability problems have arisen from a number of problems i.e. construction defects, the mixed nature of the bacterial population, the digesters requirements for water and the maintenance of the optimum nitrogen ratio of the medium. Another problem is the digester's demand for dung, which may have alternative uses. Modern designs have answered many of these problems and digesters are again becoming useful, especially with regard to the potential of digesters to remove toxic nutrients such as nitrates from water supplies; levels of which are now much more stringently controlled in many industrialised countries. The combination of energy production with the ability to enhance crop yields make biogas technology a good candidate for more widespread use now that reliable operation can be demonstrated. 4.7.2. Methane Production in Landfills. Anaerobic digestion in landfills is brought about by the microbial decomposition of the organic matter in refuse. The levels of organic matter produced per capita vary considerably from developed to developing countries e.g. the percentage of Municipal Solid Waste (MSW) which is putrescible in Burkina Faso is about 90%, compared to about 60% for US MSW. The reduced levels of putrescibles in MSW are a result of the increased proportions of plastics, metals and glass, mostly from packaging. Landfill-generated gas is on average half methane (CH4) and half carbon dioxide (CO2) with an energy content of 18 to 19 MJ/m3. Its production does not occur STEVE TSIKE ENERGY AND THE ENVIRONMENT under pressure, and thus recovery processes must be active. Commercial production of landfill- gas are affected by leaching problems associated with landfill sites. There is the potential for heavy metals pollution and nutrients leaching into aquifers. However, landfill processing reduces the volume of sludge to be disposed of, and the nutrient content, thus facilitating proper disposal. Methane is a powerful greenhouse gas, with substantial amounts being derived from unutilized methane production from landfill sites. Its recovery therefore, not only results in the stabilisation of the landfill site, allowing faster reuse of the land, but also serves to lessen the impact of biospheric methane emissions on global warming. 4.7.3. Ethanol Fermentation. Ethanol is mainly used as a substitute for imported oil in order to reduce their dependence on imported energy supplies. The substantial gains made in fermentation technologies now make the production of ethanol for use as a petroleum substitute and fuel enhancer, both economically competitive (given certain assumptions) and environmentally beneficial. For example, subsidies for alcohol production in Brazil are now becoming regarded as detrimental to the stability of the ethanol market, and thus obsolete, In Zimbabwe, foreign exchange savings are seen as a major bonus, which along with the employment and environmental benefits have made the long term future and expansion of the this programme a priority for the Zimbabwean government. The most commonly used feedstock in developing countries is sugarcane, due to its high productivity when supplied with sufficient water. Where water availability is limited, sweet sorghum or cassava may become the preferred feedstocks. Other advantages of sugarcane feedstock include the high residue energy potential and modern management practices which make sustainable and environmentally benign production possible whilst at the same time allowing continued production of sugar. Other feedstocks include saccharide-rich sugar beet, and carbohydrate rich STEVE TSIKE ENERGY AND THE ENVIRONMENT potatoes, wheat and maize. One of the most promising fermentation technologies to be identified recently is the "Biostil" process which uses centrifugal yeast reclamation, and continuous evaporative removal of the ethanol. This allows the fermentation medium to be continuously sterilised and minimises water use. The Biostil process markedly lowers the production of stillage, whilst the non-stop nature of the fermentation process allows substrate concentrations to be constantly kept at optimal levels and therefore fermentation efficiency is maximised. Improved varieties of yeast, produced through clonal selection techniques have also raised the tolerance levels of the yeast to alcohol concentrations, again improving efficiency. Recent advances in the use of cellulosic feedstock, may allow the competitive production of alcohol from woody agricultural residues and trees to become economically competitive in the medium term. 4.7.4. Biodiesel Production “Biodiesel is a liquid biofuel obtained by chemical processes from vegetable oils or animal fats and an alcohol that can be used in diesel engines, alone or blended with diesel oil”. Whilst it is feasible to run diesel engines on raw vegetable oils, in general the oils must first be chemically transformed to resemble petroleum-based diesel more closely. The raw oil can be obtained from a variety of annual and perennial plant species. Perennials include, oil palms, coconut palms,and Chinese Tallow Tree. Annuals include, sunflower, groundnut, soybean and rapeseed. Many of these plants can produce high yields of oil, with positive energy and carbon balances. It is necessary to trnsform the raw oil before use in order to avoid problems associated with variations in feedstock. The oil can undergo thermal or catalytic cracking, Kolbe electrolysis, or transesterification processes in order to obtain better characteristics. Problems with Untreated Oil 1. Untreated oil causes problems through incomplete combustion, resulting in the build up of sooty residues, waxes, gums etc. STEVE TSIKE ENERGY AND THE ENVIRONMENT 2. Incorrect viscosities can result in poor atomization of the oil resulting in poor combustion. 3. Oil polymerisation can lead to deposition on the cylinder walls. 4.7.4. 1. Advantages of the Use of Biodiesel Some of the advantages of using biodiesel as a replacement for diesel fuel are : Renewable fuel, obtained from vegetable oils or animal fats. Low toxicity, in comparison with diesel fuel. Degrades more rapidly than diesel fuel, minimizing the environmental consequences of biofuel spills. Lower emissions of contaminants: carbon monoxide, particulate matter, polycyclic aromatic hydrocarbons, aldehydes. Lower health risk, due to reduced emissions of carcinogenic substances. No sulfur dioxide (SO2) emissions. Higher flash point (100C minimum). May be blended with diesel fuel at any proportion; both fuels may be mixed during the fuel supply to vehicles. Excellent properties as a lubricant. It is the only alternative fuel that can be used in a conventional diesel engine, without modifications. Used cooking oils and fat residues from meat processing may be used as raw materials 4.7.4. 2. Disadvantages of the Use of Biodiesel There are certain disadvantages of using biodiesel as a replacement for diesel fuel that must be taken into consideration: It has slightly higher fuel consumption due to the lower calorific value of biodiesel. Slightly higher nitrous oxide (NOx) emissions than diesel fuel. Higher freezing point than diesel fuel. This may be inconvenient in cold climates It is less stable than diesel fuel, and therefore long-term storage (more than six months) of biodiesel is not recommended. STEVE TSIKE ENERGY AND THE ENVIRONMENT It may degrade plastic and natural rubber gaskets and hoses when used in pure form, in which case replacement with Teflon components is recommended. It dissolves the deposits of sediments and other contaminants from diesel fuel in storage tanks and fuel lines, which then are flushed away by the biofuel into the engine, where they can cause problems in the valves and injection systems. In consequence, the cleaning of tanks prior to filling with biodiesel is recommended 4.7.5. Raw Materials for Biodiesel Production The raw materials for biodiesel production are i. Vegetable oils, (rapeseed, soybean, palm oil, sunflower oil), non- edible oil such as castor oil, jatropha , tung, cotton, jojoba ii. Animal fats: pork, poultry, fish iii. Short chain alcohols (Methanol [(most commonly used) or ethanol] iv. Catalyst [(base, e.g., KOH, NaOH), (Acid, H2SO4, HCl)] 4.7.6. Characteristics of Oils and Fats Used in Biodiesel Production Fat = Ester of Fatty acids, (or a mixture of such compounds), --Fats and oils are organic compounds that, like carbohydrates, are composed of the elements carbon (C), hydrogen (H), and oxygen (O), arranged to form molecules. --Fat often refers specifically to triglycerides (triple esters of glycerol), that are the main components of vegetable oils and of fatty tissue in animals; or, even more narrowly, to triglycerides that are solid or semisolid at room temperature, thus excluding oils. Lipids: any of a class of organic compounds that are fatty acids or their derivatives and are insoluble in water (polar solvent) but soluble in organic solvents (non-polar or hydrophobic). They include many natural oils, waxes, and steroids. STEVE TSIKE ENERGY AND THE ENVIRONMENT 4.7.6.1. Triglycerides Triglycerides are usually made up of three (tri) fatty acids and one molecule of glycerol (glycerin is another name) as shown below. (The mono and diglycerides that are used as emulsifiers have one and two fatty acids respectively.) STEVE TSIKE ENERGY AND THE ENVIRONMENT Lipids differ in their physical states at room temperature. From a chemical viewpoint, lipids are fatty glycerol esters known as triglycerides. Fatty acids may be saturated fatty acids (SFA) or non-saturated fatty acids (NSFA). The most frequent fatty acids in oils are lauric, palmitic, stearic, linoleic and linolenic, although others may also be present. It is important to note that vegetable oils differ in their content of fatty acids. A perfect biodiesel should only comprise of mono-unsaturated fatty acids. 4.7.6.2. Fatty Acids Each kind of fat or oil has a different combination of fatty acids. The nature of the fatty acid determines the consistency of the fat or oil. For example, stearic acid is the major fatty acid in beef fat, and linoleic acid is dominant in seed oils. Fatty acids are defined as short, medium, or long chain, depending on the number of atoms in the molecule. The reason that some fat melts gradually is that as the temperature rises, each fatty acid will, in turn, soften, as its melting point is reached. Fats that melt all of a sudden mean that the fatty acids are of the same or similar type and have melting points within a narrow range. An example of such a fat is coconut fat: one second it is solid, the next, liquid STEVE TSIKE ENERGY AND THE ENVIRONMENT 4.7.6.3. Saturated/Unsaturated Saturated and unsaturated refer to the extent to which the carbon atoms in the molecule of fatty acid are linked or bonded (saturated) to hydrogen atoms. One system of fatty acid classification is based on the number of double bonds. Zero (0) double bonds: saturated fatty acids. Stearic acid is a typical long-chain saturated fatty acid One (1) double bond: monounsaturated fatty acids. Oleic acid is a typical monounsaturated fatty acid STEVE TSIKE ENERGY AND THE ENVIRONMENT 7.7.6.3.1. Types of Saturated Fats Saturated fat is found in many foods such as: Animal foods (like beef, chicken, lamb, pork, and veal) Coconut, palm, and palm kernel oils Dairy products (like butter, cheese, and whole milk) Lard Shortening STEVE TSIKE ENERGY AND THE ENVIRONMENT 7.7.6.3.2. Types of Saturated Fats There are two main types of unsaturated fats: Monounsaturated fat, which can be found in: 1. Avocados 2. Nuts and seeds (like cashews, pecans, almonds, and peanuts) 3. Vegetable oils (like canola, olive, peanut, safflower, sesame, and sunflower) Polyunsaturated fat, which can be found in: 1. Fatty fish (like herring, mackerel, salmon, trout and smelt) 2. Fish oils 3. Nuts and seeds (like cashews, pecans, almonds and peanuts) 4. Vegetable oils (like canola, corn, flaxseed, soybean and sunflower) 4.7.7. Characteristics of Alcohols Used in Biodiesel Production Alcohols that can be used in biodiesel production are those with short chains, including methanol, ethanol, butanol, and amyl-alcohol. The most widely used alcohols are methanol (CH3OH) and ethanol (C2H5OH) because of their low cost and properties. Methanol is often preferred to ethanol in spite of its high toxicity because its use in biodiesel production requires simpler technology; excess alcohol may be recovered at a low cost and higher reaction speeds are reached STEVE TSIKE ENERGY AND THE ENVIRONMENT 4.7.8. Biodiesel Production Process (Transesterification Reaction) Biodiesel is produced from vegetable oils or animal fats and an alcohol, through a transesterification reaction. This chemical reaction converts an ester (vegetable oil or animal fat) into a mixture of esters of the fatty acids that makes up the oil (or fat). Biodiesel is obtained from the purification of the mixture of fatty acid methyl esters (FAME). A catalyst is used to accelerate the reaction. According to the catalyst used, transesterification can be basic, acidic or enzymatic, the former being the most frequently used. Basic. Most frequently used at all production scales. Acid. Less frequent in industrial production, sometimes used a first stage with highly acidic raw materials. Enzymatic. Less used; the enzymes are usually lipases A generic transesterification reaction is presented below: RCOOR1 indicates an ester, R2OH an alcohol, R1OH another alcohol (glycerol), RCOOR2 an ester mixture and cat a catalyst: RCOOR1 + R2OH cat R1OH + RCOOR2 When methanol is the alcohol used in the transesterification process, the product of the reaction is a mixture of methyl esters; similarly, if ethanol were used, the reaction product would be a mixture of ethyl esters. In both cases, glycerin will be the co-product of the reaction. STEVE TSIKE ENERGY AND THE ENVIRONMENT Although transesterification is the most important step in biodiesel production (since it originates the mixture of esters), additional steps are necessary to obtain a product that complies with international standards. As a result of that, once the chemical reaction is completed and the two phases (mix of esters and glycerin) are separated, the mix of methyl esters must be purified to reduce the concentration of contaminants to acceptable levels. These include: remnants of catalyst water and methanol; The methanol is usually mixed in excess proportion with the raw materials in order to achieve higher conversion efficiency in the transesterification reaction 4.8. Stages of Biodiesel Production Process 1. Treatment of raw materials 2. Alcohol-catalyst mixing 3. Chemical reaction 4. Separation of the reaction products 5. Purification of the reaction products STEVE TSIKE ENERGY AND THE ENVIRONMENT 4.8.1. Treatment of Raw Materials The content of free fatty acids, water and non-saponificable substances are key parameters to achieve high conversion efficiency in the transesterification reaction. The use of basic catalysts in triglycerides with high content of free fatty acids is not advisable, since part of the latter reacts with the catalyst to form soaps. The consequence of that is that part of the catalyst is spent, and it is no longer available for transesterification. In summary the efficiency of the reaction diminishes with the increase of the acidity of the oil; basic transesterification is viable if the content of free fatty acids (FFAs) is less than 2%. In the case of highly acidic raw materials (animal fats from cattle, poultry, pork; vegetable oils from cotton, coconut, most used oils, etc.) an acid transesterification is necessary as a preliminary stage, to reduce the level of FFAs to the above-mentioned value Recommended oils for biodiesel production usually have: -Acidity level < 0.1 mg KOH/g -Humidity < 500 ppm -Peroxide index < 10 meq/kg -Non-saponificable substances < 1%. Base catalyzed transesterification reaction STEVE TSIKE ENERGY AND THE ENVIRONMENT 4.8.1.1. Saponification Reaction Under Base-catalyzed Transesterification Reaction In biodiesel production, soap formation creates phase separation problem during purification process resulting in low the biodiesel yield and ester content. Water can react with the triglyceride to make free fatty acids and a diglyceride. It can also dissociate the sodium or potassium from the hydroxide, and the ions Na+ and K+ can react with the free fatty acid to form soap as shown below 4.8.2. Alcohol-Catalyst Mixing The alcohol used for biodiesel production must be mixed with the catalyst before adding the oil. The mixture is stirred until the catalyst is completely dissolved in the alcohol. It must be noted that the alcohol must be water-free (anhydrous). Sodium and potassium hydroxides are among the most widely used basic catalysts. For production on an industrial scale, sodium or potassium methoxides or methylates are commercially available. The alcohol-to-oil volume ratio, R, is another key variable of the transesterification process. The stoichiometric ratio (1:3) requires 1 mol of oil to react with 3 mol of alcohol, to obtain 3 mol of Fatty Acids Methyl Esters (FAME) and 1 mol of glycerin. However, since the reaction is reversible, excess alcohol as a reactant will shift the equilibrium to the right side of the equation, increasing the amount of products (as it may be inferred from Le Chatelier’s principle). STEVE TSIKE ENERGY AND THE ENVIRONMENT Although a high alcohol-to-oil ratio does not alter the properties of FAME, it will make the separation of biodiesel from glycerin more difficult, since it will increase the solubility of the former in the latter. Usually, a 100% alcohol excess is used in practice, that is, 6 mol of alcohol per mole of oil. 4.8.3. Chemical Reaction The chemical reaction takes place when the oil is mixed with the alkoxide (alcohol–catalyst mix) The required certain conditions are: Time, Temperature Stirring. Since alcohols and oils do not mix at room temperature, the chemical reaction is usually carried out at a higher temperature and under continuous stirring, to increase the mass transfer between the phases. The transesterification process may be carried out at different temperatures. For the same reaction time, the conversion is greater at higher temperatures. Since the boiling point of methanol is approximately 68C (341 K), the temperature for transesterification at atmospheric pressure is usually in the range between 50 and 60OC 4.8.4. Catalysts in Reaction ++The catalysts used for the transesterification of triglycerides may be classified as basic, acid or enzymatic. ++Basic catalysts include sodium hydroxide (NaOH), potassium hydroxide (KOH), carbonates and their corresponding alcoxides (for instance, sodium methoxide or ethoxide). ++Acid catalysts include sulfuric acid, sulfonic acids and hydrochloric acid; their use has been less studied. ++Heterogeneous catalysts that have been considered for biodiesel production include enzymes, titanium silicates, and compounds from alkaline earth metals, anion exchange resins and STEVE TSIKE ENERGY AND THE ENVIRONMENT guanidines in organic polymers. Lipases are the most frequently used enzymes for biodiesel production 4.8.5. Separation of the Reaction Products The separation of reaction products takes place by decantation: the mixture of fatty acids methyl esters (FAME) separates from glycerin forming two phases, since they have different densities; the two phases begin to form immediately after the stirring of the mixture is stopped. Due to their different chemical affinities, most of the catalyst and excess alcohol will concentrate in the lower phase (glycerin), while most of the mono-, di-, and triglycerides will concentrate in the upper phase (FAME). Once the interphase is clearly and completely defined, the two phases may be physically separated. Centrifugation is a faster, albeit more expensive alternative. 4.8.6. Purification of the Reaction Products The mixture of fatty acids methyl esters (FAME) obtained from the transesterification reaction must be purified in order to comply with established quality standards for biodiesel. Therefore, FAME must be washed, neutralized and dried Successive washing steps with water remove the remains of methanol, catalyst and glycerin, since these contaminants are water-soluble. Care must be taken to avoid the formation of emulsions during the washing steps, since they would reduce the efficiency of the process. The first washing step is carried out with acidified water, to neutralize the mixture of esters. Then, two additional washing steps are made with water only. Finally the traces of water must be eliminated by a drying step. After drying, the purified product is ready for characterization as biodiesel according to international standards. An alternative to the above purification process is the use of ion exchange resins or silicates. STEVE TSIKE ENERGY AND THE ENVIRONMENT STEVE TSIKE ENERGY AND THE ENVIRONMENT