Endocrine System Patho PDF
Document Details
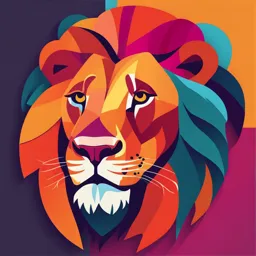
Uploaded by AstonishedWilliamsite2489
Tags
Related
- The Endocrine System and Hormones PDF
- Anatomy & Physiology II - Endocrine System PDF
- General Physiology Lecture - Endocrine System PDF
- Vander's Human Physiology - The Endocrine System PDF
- Physiology Endocrine System Self-Assessment PDF
- Behavioral Biology Lecture 10: The Endocrine System (Part 2) PDF
Summary
This document provides an introduction to the endocrine system, covering its functions, hormone characteristics, types of hormones, mechanisms of action, effects, hormones' synthesis, and elimination. It also explains the various types of hormone receptors and their role in regulating cellular responses.
Full Transcript
Endocrine System Introduction to the Endocrine System The endocrine system plays a critical role in integrating essential life functions, including growth, sex differentiation, metabolism, and adaptation to changing environmental conditions. This system uses hormones to regulate and coordinate body...
Endocrine System Introduction to the Endocrine System The endocrine system plays a critical role in integrating essential life functions, including growth, sex differentiation, metabolism, and adaptation to changing environmental conditions. This system uses hormones to regulate and coordinate body functions. It is closely associated with the nervous and immune systems, leading to terms like "neuroendocrine" or "neuroendocrine-immune" to describe their interactions. Functions of the Endocrine System The endocrine system utilizes chemical messengers called hormones to regulate and integrate various bodily processes. These include digestion, the usage and storage of nutrients, growth and development, electrolyte and water balance, and reproductive functions. The endocrine system does not function in isolation but works in close connection with the central and peripheral nervous systems and the immune system. Hormones Definition Hormones are chemical messengers transported through body fluids. These are specialized organic molecules produced by endocrine organs to act on specific target cells. Hormones do not start reactions but modulate cellular and systemic responses. Sources Hormones are released from several sites, including the endocrine glands, the brain, and other organs such as the heart, liver, and adipose tissue. Characteristics 1. Hormones are present in body fluids at all times, with their levels fluctuating according to the body's needs. 2. A single hormone can produce different effects in different tissues. 3. A single physiological function can be regulated by multiple hormones. Examples The heart produces atrial natriuretic peptide (ANP), which induces natriuresis in the kidneys. Erythropoietin, produced by the kidneys, stimulates the production of red blood cells in the bone marrow. Lipolysis, the release of free fatty acids from adipose tissue, is regulated by hormones such as catecholamines, insulin, and glucagon, as well as cytokines like tumor necrosis factor-α. Hormone Effects and Actions Hormones elicit effects based on their site of action relative to their site of release. These effects are classified as follows: 1. Endocrine Effect: Hormones are released into the bloodstream and act on distant target cells. 2. Paracrine Effect: Hormones act locally on nearby cells without entering the bloodstream, such as the action of sex steroids on the ovary. 3. Autocrine Effect: Hormones act on the same cell that produced them, such as insulin inhibiting its further release from pancreatic beta cells. 4. Intracrine Effect: Hormones are synthesized and act within the same cell. Structural Classification of Hormones Hormones are classified based on their structure into three main categories: 1. Amines and Amino Acids: These include hormones derived from single amino acids, such as norepinephrine, epinephrine, and thyroid hormones. 2. Peptides and Proteins: This is the largest category, ranging from small molecules like thyrotropin-releasing hormone (TRH, with three amino acids) to large proteins like growth hormone (GH, with approximately 200 amino acids). Glycoproteins, such as follicle-stimulating hormone (FSH), are peptide hormones combined with carbohydrates. 3. Steroids: These are derived from cholesterol and include hormones such as cortisol, estrogen, and testosterone. Synthesis, Release, Transport, and Elimination of Hormones Synthesis and Release of Hormones 1. Mechanisms of Synthesis and Release: o Hormone synthesis and release vary based on the hormone's structure, with some aspects still not fully understood. o Protein hormones are synthesized and stored in vesicles in the cytoplasm until secretion is required. o Steroid hormones are synthesized and secreted immediately upon synthesis. 2. Protein Hormones: o Protein hormones are the most prominent class of hormones using vesicle-mediated synthesis and release. o Typically, a precursor hormone is produced, which is modified by the addition of peptides or sugar units to ensure proper folding and linkage formation. o If the precursor hormone contains extra amino acids, it is termed a prohormone (e.g., insulin). 3. Release Mechanism: o Endocrine cell stimulation causes vesicles to move to the cell membrane, releasing their hormones. o The vesicle-mediated pathway is also used for some nonpolypeptide hormones and neurotransmitters, such as catecholamines (dopamine, epinephrine, norepinephrine). 4. Non-Vesicle-Mediated Hormones: o Includes glucocorticoids, androgens, estrogens, and mineralocorticoids (steroid hormones derived from cholesterol). o These hormones are synthesized in the smooth endoplasmic reticulum, with steroid-secreting cells identifiable by their abundance of smooth endoplasmic reticulum. o Certain steroids, like progesterone, serve as precursors for other hormones (e.g., aldosterone, cortisol, and androgens in the adrenal cortex). Transport of Hormones 1. Circulation Forms: o Hormones circulate in the bloodstream as either: Free or unbound molecules (e.g., peptide and protein hormones). Bound to transport carriers (e.g., steroid hormones and thyroid hormone). 2. Carrier Proteins: o Specific carrier proteins, synthesized in the liver, transport steroid hormones and thyroid hormone. o The extent of carrier binding affects: The rate at which hormones leave the blood and enter cells. The hormone's half-life (time required to reduce its concentration by half). 3. Half-Life Examples: o Thyroxine (99% protein-bound): Half-life of 6 days. o Aldosterone (15% protein-bound): Half-life of 25 minutes. 4. Drugs and Carrier Binding: o Certain drugs (e.g., aspirin) compete with hormones for binding to transport carriers. o This competition increases the availability of free, active hormone, enhancing hormone action. o Example: In thyroid crisis, aspirin competes with thyroid hormone, potentially causing serious effects due to increased levels of free hormone. Metabolism and Elimination of Hormones 1. Continuous Inactivation: o Hormones must be continuously inactivated to prevent accumulation. o Intracellular and extracellular mechanisms ensure the termination of hormone function. 2. Peptide Hormones and Catecholamines: o These hormones are water-soluble and circulate freely in the blood. o They are degraded by enzymes in the blood or tissues and excreted by the kidneys and liver. o Peptide hormones typically have a short lifespan in circulation and are degraded by: Binding to cell-surface receptors. Uptake and degradation by peptide-splitting enzymes in the cell membrane or inside the cell. 3. Steroid Hormones: o These hormones are transported bound to protein carriers and remain inactive in the bound state. o Their activity depends on the availability of transport carriers. Mechanisms of Hormone Action General Mechanism of Action 1. Hormones interact with high-affinity receptors linked to effector systems within the cell. 2. These mechanisms influence various metabolic activities, such as: o Ion transport at the cell surface. o Stimulation of nuclear transcription for complex molecule synthesis. 3. Reaction time depends on the mechanism: o Neurotransmitters: Milliseconds (e.g., controlling ion channel opening). o Thyroid hormone: Days (e.g., regulating cell metabolism and intracellular signaling molecules). Receptors 1. Function: o Recognize specific hormones. o Translate hormonal signals into cellular responses. 2. Specificity: o Receptors are specific to certain hormones. o Examples: Thyroid receptors respond to thyroid-stimulating hormone (TSH). Gonadal receptors respond to gonadotropic hormones. 3. Response Variability: o Depends on: Number of receptors present. Receptor affinity for hormone binding. o Factors influencing receptor number and affinity: Antibodies can destroy/block receptor proteins. Hormone levels can regulate receptor synthesis via gene activity. 4. Regulation: o Up-regulation: Decreased hormone levels increase receptor numbers. Increases sensitivity to existing hormone levels. o Down-regulation: Sustained excess hormone levels decrease receptor numbers. Reduces hormone sensitivity. o Receptor regulation occurs by altering receptor gene transcription. 5. Quantity: o Cells have 2000 to 100,000 receptor molecules per cell. Types of Hormone Receptors 1. Cell-Surface Receptors: o Used by peptide hormones and catecholamines. o Peptide hormones and catecholamines cannot cross the lipid cell membrane due to low solubility. o Mechanism: Hormone (first messenger) binds to surface receptors. This generates an intracellular signal (second messenger). Example: Glucagon (first messenger) binds to liver cell receptors. This triggers glycogen breakdown via the second- messenger system. 2. Intracellular Receptors: o Used by steroid and thyroid hormones. o These hormones are lipid-soluble and can cross the cell membrane. o Mechanism: Hormone binds to an intracellular receptor. The hormone–receptor complex travels to the nucleus. Binds to hormone response elements (HREs) on DNA. Activates/suppresses gene activity, leading to: Messenger RNA (mRNA) production or inhibition. Protein synthesis or suppression. Hormone Receptors Overview of Hormone Receptors Hormones exert effects by binding to specific cell receptors. Two general types: 1. Cell-Surface Receptors: Use second-messenger systems to transmit signals. 2. Intracellular Nuclear Receptors: Regulate gene expression by interacting with DNA. 1. Cell-Surface Receptors Target Hormones: Water-soluble hormones (e.g., parathyroid hormone [PTH], glucagon). Mechanism: o Hormones bind to receptor sites on the cell membrane's surface. o This triggers a structural change in the receptor, activating an intracellular signaling system. o These receptors allow cells to: Sense extracellular events. Transmit information to the intracellular environment. Types of Cell-Surface Receptors: o G-Protein–Coupled Receptors: Mediate actions of hormones like catecholamines, prostaglandins, and thyroid-stimulating hormone (TSH). Hormone binding activates a G protein. The G protein activates an effector enzyme (e.g., adenyl cyclase). The effector generates a second messenger (e.g., cyclic adenosine monophosphate [cAMP]). The second messenger: Activates intracellular enzymes. Leads to cellular responses (e.g., secretion, gene activation). 2. Nuclear Receptors Target Hormones: Lipid-soluble hormones (e.g., steroid hormones, vitamin D, thyroid hormones). Mechanism: o Hormones diffuse through the cell membrane into the cytoplasm. o Bind to an intracellular receptor, activating the receptor-hormone complex. o The activated complex moves to the nucleus. o It binds to: Hormone Response Elements (HREs) in the promoter regions of target genes. Or other transcription factors. o This leads to: Transcription of specific mRNA. The mRNA travels to the cytoplasm, where ribosomes translate it into proteins. o Resulting proteins: Induce specific cellular responses. Sometimes form structural proteins exported from the cell. Control of Hormone Levels Hormone Secretion Patterns 1. Hormone secretion varies significantly over a 24-hour period: o Diurnal fluctuations: E.g., growth hormone (GH) and adrenocorticotropic hormone (ACTH) follow the sleep-wake cycle. o Cyclic secretion: E.g., female sex hormones. o Feedback regulation: E.g., insulin (regulated by glucose levels) and antidiuretic hormone (ADH, regulated by water levels). 2. The hypothalamic–pituitary-target cell system plays a key role in regulating many hormone levels. Hypothalamic–Pituitary Regulation 1. Hypothalamus and Pituitary: o Form a control unit for various endocrine and physiological functions. o Connected by: Hypophysial portal system (blood flow from hypothalamus to anterior pituitary). Nerve axons linking the hypothalamus to the posterior pituitary (supraoptic and paraventricular nuclei). o Pituitary gland is enclosed in the sella turcica and protected by the diaphragma sellae. 2. Hypothalamic Hormones: o Regulate anterior pituitary hormone secretion via releasing and inhibiting hormones. o Synthesized hormones for posterior pituitary (ADH and oxytocin) are made in hypothalamic neurons. o Key hypothalamic hormones: GH-releasing hormone (GHRH), somatostatin, dopamine, thyrotropin-releasing hormone (TRH), corticotropin-releasing hormone (CRH), gonadotropin-releasing hormone (GnRH). 3. Neuronal and Hormonal Regulation: o Hypothalamus responds to: Negative feedback signals from hormones. Neuronal inputs via neurotransmitters (e.g., acetylcholine, dopamine, norepinephrine, serotonin, GABA). Cytokines involved in immune and inflammatory responses. Pituitary Hormones 1. Known as the master gland: o Anterior pituitary hormones regulate: Body growth and metabolism: GH. Thyroid gland function: TSH. Glucocorticoid levels: ACTH. Gonadal function: FSH, LH. Breast growth and milk production: Prolactin. 2. Hormones from the posterior pituitary: o ADH and oxytocin are synthesized in the hypothalamus and released by the posterior pituitary. Feedback Regulation 1. Negative Feedback: o Most hormone levels are regulated by negative feedback mechanisms. o Mechanism: Sensors detect changes in hormone levels. Low hormone levels: Stimulate increased production. High hormone levels: Suppress production. o Example: High thyroid hormone levels suppress TSH secretion from the anterior pituitary, reducing thyroid hormone output. 2. Positive Feedback: o Less common and not as well understood. o Rising levels of one hormone stimulate another hormone that amplifies the first. o Example: Estradiol during the follicular phase of the menstrual cycle increases FSH production, leading to further estradiol production until the follicle is destroyed. Diagnostic Tests in Endocrinology Overview Endocrinology is highly dependent on laboratory testing, but clinical examination and medical history remain vital. A comprehensive approach considers the patient as a whole, accounting for various complex factors. Strict adherence to testing protocols (preparation, timing, collection, and storage) is crucial for reliable results. Errors can occur; the quality of results depends on the quality of the specimen. Techniques for Assessing Endocrine Function 1. Direct Measurement of Hormones: o Measures the hormone levels in blood or other samples. o Most commonly used method. 2. Indirect Methods: o Assess the physiological effects of hormones (e.g., blood glucose as a marker for insulin availability). Blood Tests for Endocrine Disorders 1. General Characteristics: o Blood tests provide a snapshot of hormone levels at a specific time. o Can measure hormone levels directly or use physiologic indicators. o Example: Measuring insulin levels alongside blood glucose after a glucose challenge to assess insulin response. 2. Radioimmunoassay (RIA): o Measures plasma hormone levels through competitive binding. o Methodology: Uses a radiolabeled hormone and an antibody prepared with purified hormone. The unlabeled hormone in the sample competes with the radiolabeled hormone for antibody binding. The level of radiolabeled hormone-antibody complex is inversely related to the hormone concentration in the sample. o Limitations: Cross-reactivity may reduce specificity. Radiolabeled hormones have a limited shelf life. Disposal of radioactive waste is costly. 3. Immunoradiometric Assay (IRMA): o A refined version of RIA that uses two antibodies: Directed at different parts of the hormone molecule for better specificity. o Offers higher specificity than traditional RIA. 4. Autoantibody Testing: o Detects autoimmune endocrine disorders. o Common tests include: Anti-thyroid peroxidase (anti-TPO) antibodies for Hashimoto thyroiditis. Autoantibody testing for conditions like: Type 1 diabetes Graves disease Autoimmune hypoparathyroidism Autoimmune Addison disease Diagnostic Tests in Endocrinology (Continued) Urine Tests Purpose: Measures hormone or hormone metabolite excretion over 24 hours. Advantages: o Provides a better measure of hormone levels over time than a single blood sample. o Non-invasive and easy to collect. Disadvantages: o Timed urine collections can be unreliable (e.g., accidental discarding or improper preservation of samples). o Relies on adequate renal function. o Hormone metabolism interference (by drugs or diseases) may affect results. Requirement: Complete health history (including medication use) and strict adherence to procedures. Stimulation and Suppression Tests 1. Stimulation Tests: o Used to evaluate suspected hypofunction of an endocrine organ. o Mechanism: Administer a tropic/stimulating hormone to assess the gland's capacity to produce hormones. o Example: TRH stimulation test: Measures TSH response. Lack of TSH increase suggests pituitary dysfunction. 2. Suppression Tests: o Used to diagnose suspected hyperfunction of an endocrine organ. o Mechanism: Tests if the organ can respond to negative feedback and reduce hormone production. o Example: GH response to glucose: Normally, glucose suppresses GH levels. In acromegaly, GH levels are not suppressed and may paradoxically increase. Genetic Tests Purpose: Analyze DNA to identify genetic causes of endocrine diseases. Advances: o Human genome sequencing (23,000 genes identified). o Proteomics (study of proteins) complements genomics (DNA) and transcriptomics (mRNA). o Proteins produced by normal/abnormal endocrine cells provide insights into pathophysiology. Applications: o Development of targeted therapies. o Research on endocrine diseases like type 2 diabetes, metabolic syndrome, and obesity. o Exploration of genetic risk factors considering race, ethnicity, socioeconomic conditions, and evolution. Future Prospects: o Expanded genetic testing for diagnosis, risk assessment, and treatment of endocrine conditions. Imaging Studies 1. Isotopic Imaging: o Uses radioactive substances for scanning. o Examples: Radioiodine scanning: Thyroid imaging. Sestamibi scanning: Parathyroid imaging. 2. Nonisotopic Imaging: o MRI: Preferred for pituitary and hypothalamic imaging. o CT scans: Best for adrenal and abdominal endocrine lesions. o Ultrasound: Excellent for thyroid and parathyroid anatomy. Useful for thyroid nodule visualization and biopsy guidance (fine-needle aspiration). Not considered definitive for diagnosis. o DEXA (Dual-energy X-ray absorptiometry): Standard for diagnosing osteoporosis and metabolic bone diseases. o PET Scans: Increasingly used for evaluating endocrine tumors. PET/CT imaging: Combines PET and CT for enhanced detail and identification of complex structures. Feedback Regulation of Hormone Levels Overview Hormone levels in the endocrine system are regulated by feedback mechanisms. Feedback can be negative or positive, with most hormonal regulation involving negative feedback. Some mechanisms involve simple loops, while others involve complex loops (e.g., hypothalamic-pituitary-target cell regulation). 1. Negative Feedback Definition: The most common regulation mechanism where the hormone or its effect inhibits further secretion to maintain balance. Mechanism: o The hormone level or its physiologic effect regulates the endocrine gland's response. o Example: Insulin and Blood Glucose Regulation: 1. After a meal, blood glucose rises. 2. The pancreas secretes insulin. 3. Insulin prompts glucose uptake by target cells, lowering blood glucose. 4. Lower glucose levels suppress further insulin release. 5. This cycle maintains glucose levels within an optimal range. 2. Hypothalamic-Pituitary Target Cell Feedback Involves complex loops regulating hormones of the thyroid, adrenal cortex, and gonads. Mechanism: 1. The hypothalamus secretes a releasing hormone. 2. The anterior pituitary produces a tropic hormone in response. 3. The tropic hormone stimulates the peripheral target gland to secrete its hormone. 4. The target gland hormone acts on target cells, generating a physiologic response. 5. Increased levels of the target gland hormone provide feedback to the hypothalamus and anterior pituitary to reduce tropic hormone secretion. 6. This cycle ensures hormone levels remain within a narrow, optimal range. 3. Positive Feedback Definition: A less common mechanism where a hormone stimulates continued secretion until a specific event occurs or levels are appropriate. Mechanism: o Example: Preovulatory LH Surge: 1. Rising estrogen levels during the menstrual cycle stimulate the anterior pituitary. 2. The anterior pituitary secretes more luteinizing hormone (LH). 3. This positive feedback loop continues until LH levels trigger ovulation. 4. The cycle ceases once ovulation occurs. Hypofunction and Hyperfunction of the Endocrine System 1. Categories of Endocrine Disturbances Hypofunction Definition: A decrease in endocrine gland activity resulting in reduced hormone production. Causes: o Developmental Issues: Absence or impaired development of glands. o Enzyme Deficiencies: Impair hormone synthesis. o Destruction of Glands: Disrupted blood flow. Infection, inflammation, autoimmune responses, or tumors. o Aging or Atrophy: Drug therapy or unknown causes. o Receptor Defects: Missing or defective hormone receptors. Impaired cellular response to hormones. o Other Mechanisms: Biologically inactive hormones. Destruction of active hormones by antibodies. Hyperfunction Definition: Excessive hormone production due to overstimulation or abnormal gland activity. Causes: o Hyperplasia of Glands. o Hormone-Producing Tumors: Can cause ectopic hormone production (e.g., bronchogenic tumors secreting ACTH or ADH). o Clinical Example: A woman with vaginal small cell carcinoma developing Cushing syndrome due to ACTH secretion by the tumor. 2. Types of Endocrine Disorders Primary Disorders Originates in the target gland responsible for hormone production. Example: Thyroidectomy leading to primary thyroid hormone deficiency. Secondary Disorders Target gland is normal, but pituitary hormones are deficient. Example: Pituitary damage causing lack of ACTH and subsequent adrenal insufficiency. Tertiary Disorders Caused by hypothalamic dysfunction, leading to inadequate stimulation of the pituitary and target glands. Example: Cerebral irradiation or craniopharyngiomas. 3. Pituitary and Growth Disorders Anatomy of the Pituitary Gland Location: Base of the brain in the sella turcica. Structure: o Anterior Lobe (Adenohypophysis): Glandular tissue secreting hormones (e.g., ACTH, TSH, GH, LH, FSH, prolactin). o Posterior Lobe (Neurohypophysis): Neural tissue. Hormone Functions ACTH: Stimulates cortisol secretion from the adrenal gland. TSH: Regulates thyroid hormone production. LH and FSH: Control sex hormones and fertility. GH: Regulates growth and metabolism. 4. Assessment of Hypothalamic-Pituitary Function Baseline Hormonal Measurements (ideally before 8:00 AM): o Cortisol, prolactin, thyroxine (TSH), sex hormones (testosterone/estrogen), LH, FSH, GH, and IGF-1. o Plasma and urine osmolality. Imaging Studies: o MRI of the hypothalamus and pituitary. Dynamic Testing: o Hormone stimulation (e.g., ACTH stimulation test). o Hormone suppression (e.g., GH suppression test). 5. Pituitary Tumors Classification Primary Tumors: o Functional: Secrete hormones. o Nonfunctional: Do not secrete hormones. o Size: Microadenomas (10 mm). Secondary Tumors: Metastatic lesions. Pathophysiology Functional Adenomas: o Most are benign and hormone-secreting. Nonfunctional Adenomas: o Often discovered incidentally. Carcinomas: o Rare compared to adenomas. 6. Hypopituitarism Definition Decreased secretion of pituitary hormones affecting multiple endocrine systems. Causes Tumors, radiation, surgery, infiltrative diseases, infarction, genetic defects, and trauma. Clinical Manifestations Gradual symptoms: Weakness, fatigue, appetite loss, sexual dysfunction, and cold intolerance. ACTH Deficiency (most critical): Causes weakness, nausea, hypotension, and adrenal insufficiency. Sequence of Hormone Loss (Mnemonic: "Go Look For The Adenoma"): 1. GH: Growth and metabolism issues. 2. LH: Sex hormone deficiency. 3. FSH: Infertility. 4. TSH: Hypothyroidism. 5. ACTH: Adrenal insufficiency. Treatment Hormone Replacement: o Cortisol for ACTH deficiency. o Thyroid hormones for TSH deficiency. o Sex hormones for LH and FSH deficiency. o GH for pediatric and adult deficiencies. Underlying Causes: Tumor removal, radiation, or medication adjustments. Hormones Essential for Growth and Maturation 1. Growth Hormone (GH): Promotes bone and cartilage growth and regulates metabolic processes. 2. Insulin: Supports growth through its role in carbohydrate and fat metabolism; deficiency in children (e.g., diabetes) can impair growth. 3. Thyroid Hormones: Necessary for bone growth and epiphyseal closure; low levels delay these processes. 4. Androgens: Stimulate protein synthesis, contributing to growth. 5. Glucocorticoids: Excessive levels inhibit growth by opposing GH secretion. Growth Hormone (GH): Nature and Production: GH is a 191-amino-acid polypeptide secreted by somatotropes in the anterior pituitary. It plays a vital role in growth and metabolic regulation across all ages, not just during childhood. Functions: o Stimulates linear bone growth at epiphyseal plates and increases periosteal growth (bone width). o Enhances growth of visceral organs, muscles, skin, and connective tissues. o Promotes protein synthesis, mobilizes fatty acids for fuel, and maintains blood glucose by inhibiting its uptake in peripheral tissues. o Induces insulin-like growth factors (IGFs), especially IGF-1, which mediate bone and cartilage growth. Regulation: GH secretion is modulated by: o Stimulatory factors: GH-releasing hormone (GHRH), hypoglycemia, fasting, stress, and exercise. o Inhibitory factors: Somatostatin, high glucose levels, and obesity. o Ghrelin: Stimulates appetite, GH release, and fat accumulation. Secretion Pattern: Peaks during deep sleep (stages 3 and 4), with a half-life of 20–50 minutes. Short Stature in Children Definition: Attained height below the third percentile or slower-than- expected linear growth for age and sex. Causes: 1. Variants of Normal: o Genetic or familial short stature. o Constitutional short stature (e.g., delayed growth and maturation in otherwise healthy children). 2. Endocrine Disorders: o GH deficiency: Primary (idiopathic, pituitary agenesis) or secondary (panhypopituitarism). o Hypothyroidism and inadequate IGF-1 production. o Glucocorticoid excess (e.g., Cushing syndrome, prolonged steroid use). 3. Chronic Illness and Malnutrition: o Conditions like chronic kidney disease, diabetes, or malabsorption syndromes. o Nutritional deprivation and malabsorption (e.g., celiac disease). 4. Psychosocial Factors: o Emotional deprivation causing functional endocrine disturbances (psychosocial dwarfism). 5. Chromosomal and Skeletal Abnormalities: o Turner syndrome, achondroplasia. Diagnosis of Short Stature 1. Growth Monitoring: o Sequential height measurements and growth velocity studies. o Comparison with parental height and growth charts. 2. Hormonal Testing: o GH and IGF-1 levels. o Stimulated tests using insulin, GHRH, or arginine. o GH reserve evaluation through exercise or pharmacological agents. 3. Radiologic Assessment: o Bone age determination. o MRI of hypothalamic-pituitary area if a lesion is suspected. Management Address Underlying Causes: o Hormonal deficiencies (e.g., GH replacement for GH deficiency, thyroid hormone for hypothyroidism). o Correction of chronic illnesses and malnutrition. o Psychosocial intervention for neglected children. Catch-Up Growth: o Rapid growth occurs once treatment begins, especially in endocrine or nutritional deficiencies. Specific Conditions 1. Genetic/Constitutional Short Stature: Normal variation; children achieve a height proportional to parental stature. 2. Psychosocial Dwarfism: o Associated with severe emotional deprivation. o Growth normalizes after improving the child's environment. Growth Hormone (GH) Deficiency and Excess Growth Hormone (GH) Deficiency in Children Forms and Causes 1. Idiopathic GH Deficiency: Lack of hypothalamic GH-releasing hormone (GHRH) despite normal somatotropes in the anterior pituitary. 2. Pituitary Tumors or Agenesis: Absence of somatotropes causing GH deficiency. 3. Panhypopituitarism: Deficiency of all anterior pituitary hormones. 4. Laron-type Dwarfism: Normal/elevated GH levels with hereditary defect in IGF production. Treated with IGF-1 replacement therapy. Clinical Features Congenital Deficiency: o Decreased birth length. o Short stature becomes noticeable by 1–2 years. o Normal intelligence, immature facial features, delayed skeletal maturation, and delayed puberty. o Severe hypoglycemia and seizures in neonates. o Microphallus in males (if GnRH deficiency is present). Acquired Deficiency: Develops in later childhood due to tumors or other causes. Treatment GH Replacement Therapy: Human recombinant GH via daily subcutaneous injections during active growth. Other Uses: Effective in Turner syndrome, chronic renal insufficiency, and certain cases of idiopathic short stature. Ethical Concerns: Misuse of GH for cosmetic or non-medical height enhancement. Growth Hormone (GH) Deficiency in Adults Types 1. Childhood-Onset: Persistent GH deficiency into adulthood. 2. Adult-Onset: Often secondary to hypopituitarism from pituitary tumors or treatments. Clinical Impact Cardiovascular risks: Atherosclerosis, central adiposity, insulin resistance, and dyslipidemia. Increased high-sensitivity C-reactive protein (hsCRP) indicating systemic inflammation. Diagnosis Low GH responses to stimulation tests (e.g., insulin-induced hypoglycemia). Low IGF-1 levels in known pituitary disease. Tests include arginine plus GHRH, glucagon, or l-dopa. Treatment GH replacement improves lean body mass, bone density, lipid profiles, and well-being. Side effects: Peripheral edema, joint and muscle pain, carpal tunnel syndrome, and glucose intolerance. Growth Hormone (GH) Excess Excess in Children: Gigantism: Caused by somatotrope adenomas before epiphyseal plate closure, leading to excessive skeletal growth. High levels of IGF-1 drive abnormal height and bone elongation. Early recognition and treatment of adenomas are critical to prevent complications. Excess in Adults: Not detailed in the text provided. Tall Stature in Children Normal Variants: Genetic tall stature or constitutional tall stature (taller than peers but within normal growth velocity). Pathological Causes: o Genetic disorders (e.g., Marfan syndrome, XYY syndrome). Management: o Sex Hormone Therapy: Administer estrogens (girls) or testosterone (boys) to promote early epiphyseal plate closure. o Initiated 3–4 years before expected epiphyseal fusion after careful risk assessment. Growth Hormone Excess in Adults (Acromegaly) Definition and Incidence Acromegaly is a condition caused by excessive secretion of growth hormone (GH) in adulthood, after the long bone epiphyses have fused. It has an annual incidence of 3-4 cases per million, typically diagnosed at 40–45 years of age. Etiology and Pathogenesis Most cases (95%) are caused by somatotrope adenomas (tumors in the pituitary gland). Other rare causes include: o Hypothalamic tumors producing excess GHRH. o Ectopic GHRH secretion by tumors (e.g., carcinoid tumors, small cell lung cancers). o Ectopic GH secretion by nonendocrine tumors. Excess GH stimulates hepatic secretion of insulin-like growth factor 1 (IGF- 1), leading to clinical manifestations. Clinical Manifestations 1. Physical Changes: o Enlarged hands, feet, and membranous facial bones (broad nose, protruding jaw, slanting forehead). o Splayed teeth, disturbed bite, difficulty chewing. o Enlarged laryngeal structures causing a deepened voice and bronchitis. o Vertebral changes causing kyphosis (hunchback). o Arthralgias and degenerative arthritis in major joints. 2. Metabolic Effects: o Increased free fatty acids, ketone formation, and preference for fat metabolism over carbohydrates and proteins. oInsulin resistance, glucose intolerance, and eventual beta-cell "burnout," leading to diabetes in some cases. 3. Organ and System Involvement: o Enlargement of internal organs, including the heart, leading to cardiomyopathy and accelerated atherosclerosis. o Symptoms like oily skin, heat intolerance, weight gain, muscle weakness, menstrual irregularities, and decreased libido. o Sleep apnea (90% prevalence) due to increased pharyngeal soft tissue. o Increased risk of colonic polyps and colorectal cancer. 4. Neurological Effects: o Headaches, visual field defects (bitemporal hemianopia), and cranial nerve palsies due to tumor pressure. o Secondary hormone deficiencies (e.g., hypothyroidism, hypogonadism, adrenal insufficiency). Diagnosis Key features: Enlarged hands, feet, and coarsened facial features. Laboratory tests: Elevated GH levels not suppressed by glucose load; increased IGF-1 levels. Imaging: MRI to detect pituitary lesions. Treatment 1. Goals: o Normalize IGF-1 levels. o Remove or reduce tumor mass. o Relieve central pressure effects. o Address metabolic and clinical abnormalities. 2. Methods: o Surgery: Transsphenoidal removal of pituitary tumors. o Medical Therapy: Somatostatin analogs to inhibit GH release. Dopamine agonists to reduce GH levels. GH receptor antagonists to block GH action. o Adjunctive Treatment: Radiotherapy in some cases. Isosexual Precocious Puberty Definition Early activation of the hypothalamic–pituitary–gonadal axis resulting in sexual development before: o 7 years in white girls, 6 years in African American girls. o 9 years in boys. Etiology May be idiopathic or caused by gonadal, adrenal, or hypothalamic disease. CNS tumors (benign/malignant) may remove inhibitory influences on the hypothalamus. Clinical Manifestations Girls: Early breast development (thelarche), augmented adrenal androgen production (adrenarche), early menstruation (menarche). Boys: Early genital enlargement. Advanced bone age; initially tall stature but short final height due to early epiphyseal closure. Diagnosis Physical examination to confirm secondary sexual characteristics. Imaging (MRI/CT) to exclude intracranial lesions. Advanced bone age noted radiologically. Treatment Surgical: Removal of tumors if present. Medical: o Long-acting GnRH agonists to reduce gonadotropin secretion by desensitizing GnRH receptors. No treatment may be required in benign cases. Control of Thyroid Function The thyroid gland, located below the larynx in the anterior middle neck, is a shield-shaped structure composed of numerous tiny follicles. These follicles, lined with epithelial cells, store a colloid substance that primarily contains thyroglobulin, a glycoprotein–iodine complex. Thyroglobulin includes 140 tyrosine amino acids, essential for thyroid hormone synthesis, where iodine molecules attach to tyrosine. Iodine and Thyroid Hormone Synthesis A daily intake of 50 mg iodine (~1 mg/week) is necessary for normal thyroid hormone production. Iodine is efficiently absorbed and stored in the thyroid against a concentration gradient using: Na+/I− Symporter (NIS): Transports iodide into thyroid cells using energy from Na+/K+-ATPase. Pendrin: Transfers iodide into the colloid for hormone formation. Mutations in the PDS gene (Pendred syndrome) are associated with goiter and congenital deafness. In the colloid, thyroid peroxidase (TPO) oxidizes iodide, which binds to tyrosine to form: Monoiodotyrosine (MIT) Diiodotyrosine (DIT) Hormone synthesis occurs by coupling: Two DITs → Thyroxine (T4) One MIT + One DIT → Triiodothyronine (T3) Only T4 (90%) and T3 (10%) enter circulation, with T3 being the active form after T4 converts to T3. Hormone Transport Thyroid hormones are primarily transported in the blood bound to proteins: Thyroxine-binding globulin (TBG): Binds 70% of T4 and T3. Transthyretin (TBPA): Binds ~10% of T4 and smaller T3 amounts. Albumin: Binds ~15% of T4 and T3. Only unbound (free) hormones are biologically active. Binding protein levels can be influenced by conditions like malnutrition, nephrotic syndrome, or cirrhosis, and by drugs like phenytoin and salicylates. Regulation via Feedback The hypothalamic–pituitary–thyroid axis controls thyroid hormone secretion: The hypothalamus releases thyrotropin-releasing hormone (TRH). TRH stimulates the anterior pituitary to release thyroid-stimulating hormone (TSH). TSH enhances thyroid activity by: o Increasing thyroglobulin breakdown. o Activating the iodide pump. o Promoting iodide oxidation and its coupling with tyrosine. o Enlarging follicular cells. High thyroid hormone levels inhibit TRH and TSH secretion, forming a negative feedback loop. Additionally, excessive iodide temporarily inhibits thyroid activity. Cold exposure strongly stimulates thyroid hormone production via hypothalamic TRH release, while emotional stress can alter TRH and TSH secretion. Actions of Thyroid Hormone Thyroid hormones, primarily T3, impact all major organs by increasing metabolism, promoting protein synthesis, and supporting growth and development in children, including mental and sexual maturation. T3 binds to nuclear receptors in cells, initiating gene transcription for thyroid hormone response. 1. Metabolic Effects Increased Basal Metabolic Rate (BMR): Thyroid hormone raises BMR by 60–100%, affecting all tissues except the retinas, spleen, testes, and lungs. Energy Utilization: o Enhances glucose, fat, and protein metabolism. o Mobilizes lipids from adipose tissue. o Increases cholesterol catabolism in the liver. o Hypothyroidism: Elevated blood cholesterol. o Hyperthyroidism: Decreased blood cholesterol and muscle protein catabolism, causing muscle fatigue. Glucose Absorption: Increases absorption in the gastrointestinal tract. 2. Cardiovascular and Respiratory Effects Increased Metabolism: Drives higher oxygen consumption and metabolic byproducts, leading to vasodilation. Enhanced Circulation: o Increased blood flow, particularly to the skin, dissipating heat. o Higher blood volume, cardiac output, and ventilation maintain oxygen delivery. Heart Rate and Contractility: Elevated to support cardiac output. Blood Pressure: Minimal change due to balanced vasodilation and cardiac output. 3. Gastrointestinal Effects Enhanced Motility and Secretions: Increased motility and secretion can lead to diarrhea. Increased Appetite: Driven by a higher metabolic rate, though weight loss occurs due to calorie expenditure. 4. Neuromuscular Effects Muscle Response: o Slight hormone elevation → Faster muscle response. o Low hormone levels → Sluggish muscle reaction. o Hyperthyroidism: Fine muscle tremors, likely due to heightened spinal cord synapse sensitivity. Brain Development: Essential in infants for normal neural growth. Cerebration: o Hyperthyroidism causes extreme nervousness, anxiety, and insomnia. 5. Interaction with the Sympathetic Nervous System (SNS) Thyroid hormones enhance SNS activity, producing symptoms like: o Tachycardia (rapid heartbeat), palpitations, sweating, tremors, and restlessness. o Anxiety and diarrhea may reflect autonomic imbalance. Sympathetic Blockers: Effective in alleviating symptoms of hyperthyroidism by reducing SNS overactivity. Tests of Thyroid Function Several tests help diagnose thyroid disorders: 1. T3, T4, and TSH Measurement o Free T4 Test: Measures unbound T4, which is free to enter cells and exert its effects. This is typically the first laboratory test performed. o TSH Levels: Used to differentiate between primary (thyroid-related) and secondary (pituitary-related) thyroid disorders. In primary hypothyroidism, T3, T4, and free T4 are low, while TSH is elevated. o Thyroid Autoantibodies: Important for diagnosing autoimmune thyroid disorders like Hashimoto thyroiditis. 2. Radioiodine Uptake Test o Measures the thyroid gland's ability to concentrate and retain iodine, aiding in the diagnosis of thyroid function. 3. Thyroid Scans o Using 123I or 99mTc-pertechnetate, these scans help detect thyroid nodules and evaluate the gland's functional activity. 4. Ultrasonography o Differentiates between cystic and solid thyroid lesions. 5. CT and MRI Scans o Used to assess the impact of thyroid enlargement on neighboring structures, such as tracheal compression. 6. Fine-Needle Aspiration Biopsy o Considered the best method to distinguish benign from malignant thyroid disease in thyroid nodules. Alterations in Thyroid Function Thyroid dysfunction can be either hypofunctional (underactive) or hyperfunctional (overactive). Common alterations include: 1. Goiter o Enlargement of the thyroid gland. o Types of Goiter: Diffuse Goiter: Entire thyroid gland enlarges without nodules. Nodular Goiter: Presence of thyroid nodules. Toxic Goiter: Associated with hyperthyroidism or thyrotoxicosis (excessive thyroid hormone). Nontoxic Goiter: Result of thyroid hormone deficiency. o Compensatory Hypertrophy and Hyperplasia: Seen in conditions where the thyroid's hormone output is impaired. o Symptoms of Large Goiters: Compression of the esophagus and trachea, leading to difficulty swallowing, choking sensation, and inspiratory stridor. If the goiter compresses the superior vena cava, symptoms can include neck and upper extremity vein distension, eyelid and conjunctival edema, and syncope with coughing. Hypothyroidism Hypothyroidism can be classified into congenital and acquired types: 1. Congenital Hypothyroidism Definition: A condition present at birth due to either the complete absence of the thyroid gland or a defect in the thyroid hormone synthesis or secretion of TSH (thyroid-stimulating hormone). Prevalence: It affects approximately 1 in 4,000 infants. Causes: o Complete absence of the thyroid gland: The infant appears normal at birth due to maternal hormone supply in utero. o Abnormal thyroid hormone synthesis or deficient TSH secretion. Impacts: o Thyroid hormone is essential for normal growth and brain development, particularly in the first 6 months of life. If untreated, it leads to mental retardation and impaired physical growth. o The untreated condition is referred to as cretinism, but this term is avoided if the infant receives thyroid hormone replacement soon after birth. Neonatal Screening: o Screening is usually done in the hospital nursery by taking a blood sample from the infant's heel to measure T4 and TSH levels. Transient Hypothyroidism: o Transient hypothyroidism, which can occur due to maternal or infant exposure to iodine or substances like povidone-iodine, is increasingly recognized due to neonatal screening. o Maternal antithyroid drugs such as propylthiouracil and methimazole can also block fetal thyroid function. Treatment: o The main treatment for congenital hypothyroidism is hormone replacement therapy. Early and adequate treatment ensures that the child’s development is almost normal, with very little risk of mental retardation. o Treatment involves adjusting thyroid hormone (T4) dosage as the child grows. Acquired Hypothyroidism and Myxedema Acquired hypothyroidism refers to hypothyroidism that develops after birth and is commonly caused by primary dysfunction of the thyroid gland, but it can also result from issues in the pituitary or hypothalamus. 1. Etiology and Pathogenesis: Primary Hypothyroidism: The most common type, often caused by: o Thyroidectomy (surgical removal of the thyroid) or radiation therapy that destroys thyroid tissue. o Certain goitrogenic agents (e.g., lithium carbonate, antithyroid drugs like propylthiouracil, and methimazole) that inhibit thyroid hormone synthesis. o Excess iodine intake (e.g., from kelp tablets, iodine-containing cough syrups, or medications like amiodarone). o Hashimoto thyroiditis: An autoimmune disorder where the immune system attacks the thyroid gland, leading to hypothyroidism. It is the most common cause of hypothyroidism in both children and adults. Secondary and Tertiary Hypothyroidism: Result from pituitary or hypothalamic dysfunction, respectively. 2. Clinical Manifestations: Hypothyroidism leads to a hypometabolic state, and its symptoms may include: Fatigue and weakness Weight gain despite a loss of appetite Cold intolerance Dry, rough skin and coarse, brittle hair Puffy face with edematous eyelids and thinning of the outer third of the eyebrows Constipation, abdominal distention, and flatulence due to reduced gastrointestinal motility Bradycardia and delayed relaxation of deep tendon reflexes Mental dullness, lethargy, and impaired memory Myxedema: Characterized by non-pitting edema due to the accumulation of mucopolysaccharides in the connective tissues, which can affect various organs and tissues. The tongue may become enlarged, and the voice may be hoarse. Carpal tunnel syndrome and other entrapment syndromes are common. Impaired muscle function with stiffness, cramps, and pain. Cardiac dilation, bradycardia, and other signs of altered cardiac function due to mucopolysaccharide deposits. 3. Diagnosis: Serum T4 levels are low, and TSH levels are elevated in primary hypothyroidism. Antithyroid antibodies (especially anti-TPO) are tested when Hashimoto thyroiditis is suspected. 4. Treatment: Thyroid hormone replacement (usually T4) is the primary treatment. o TSH levels guide the adjustment of T4 dosage. o A "go low and go slow" approach is recommended, especially for elderly individuals due to the risk of triggering acute coronary syndromes. o Consistency with generic or brand-name T4 is crucial for maintaining accurate treatment and monitoring. 5. Myxedematous Coma: Myxedematous coma is a severe, life-threatening complication of hypothyroidism, presenting with: o Coma, hypothermia, cardiovascular collapse, and hypoventilation. o Metabolic disorders like hyponatremia, hypoglycemia, and lactic acidosis. Treatment includes: o Managing underlying precipitating factors (e.g., infections or medications). o Supportive care for cardiorespiratory function and fluid/electrolyte balance. o Thyroid hormone replacement to restore thyroid function. o Active rewarming is avoided in cases of hypothermia, as it may lead to vascular collapse. 6. Prevention: Prevention is the best strategy for individuals at risk, such as those with a history of Hashimoto thyroiditis. Awareness of hypothyroid symptoms and early treatment can prevent severe complications. Hyperthyroidism Hyperthyroidism (also known as thyrotoxicosis) occurs when the body is exposed to high levels of circulating thyroid hormones. It is primarily caused by overactivity of the thyroid gland. 1. Etiology and Pathogenesis: Graves' Disease is the most common cause, characterized by hyperthyroidism, a diffuse goiter, and ophthalmopathy (eye involvement). Other causes include multinodular goiter, thyroid adenomas, and thyroiditis. Iodine-containing agents can induce both hyperthyroidism and hypothyroidism. Thyroid Crisis (Thyroid Storm): An acute, life-threatening exacerbation of thyrotoxicosis that can occur under extreme conditions. 2. Clinical Manifestations: Hypermetabolic state and increased sympathetic nervous system activity lead to: o Nervousness, irritability, and fatigability. o Weight loss despite increased appetite. o Tachycardia, palpitations, and shortness of breath. o Excessive sweating, muscle cramps, and heat intolerance. o Fine muscle tremors, restlessness, and staring appearance (due to infrequent blinking or eyelid retraction). o Thin, silky hair and skin. Older adults may present with new-onset atrial fibrillation. 3. Diagnosis and Treatment: The goal of treatment is to reduce thyroid hormone levels. o Radioactive iodine therapy (common treatment) or surgery (thyroidectomy) to remove part or all of the thyroid gland. o Antithyroid drugs (e.g., propylthiouracil and methimazole) inhibit hormone synthesis. o Beta-blockers (e.g., propranolol, metoprolol) are used to manage the effects of increased sympathetic activity (e.g., tachycardia, tremors). 4. Graves' Disease: An autoimmune disorder that causes the thyroid to be abnormally stimulated by TSH receptor antibodies. Often associated with other autoimmune diseases like myasthenia gravis. Ophthalmopathy, affecting up to one-third of individuals with Graves' disease, is due to accumulation of T lymphocytes in the orbital area. This can result in exophthalmos (bulging eyes) and other serious eye complications like diplopia, corneal ulceration, and optic nerve involvement. Smoking aggravates ophthalmopathy, so cessation is strongly advised. 5. Thyroid Storm (Thyrotoxic Crisis): A severe, life-threatening exacerbation of hyperthyroidism, usually triggered by stress, infection, surgery, or untreated hyperthyroidism. Symptoms include: o Very high fever, extreme tachycardia, and cardiovascular collapse (e.g., congestive heart failure, angina). o Severe CNS effects, including agitation, delirium, and restlessness. Treatment involves: o Hemodynamic stabilization (fluids, glucose, and electrolytes). o Beta-blockers to control cardiovascular symptoms. o Glucocorticoids to correct adrenal insufficiency and inhibit T4 to T3 conversion. o Antithyroid drugs (e.g., propylthiouracil, methimazole) to block thyroid hormone synthesis. o Plasmapheresis, dialysis, or hemoperfusion may be used to remove thyroid hormones. o Cooling measures are essential to manage hyperthermia. Disorders of Adrenal Cortical Function Control of Adrenal Cortical Function The adrenal glands are small, bilateral structures located above the kidneys. The adrenal medulla (inner portion) secretes epinephrine and norepinephrine, part of the sympathetic nervous system, while the adrenal cortex (outer 80%) secretes glucocorticoids, mineralocorticoids, and adrenal androgens. Adrenal cortical function is essential for life, as total loss can be fatal without treatment. This section discusses the biosynthesis, metabolism, and functions of these hormones, as well as the effects of insufficiency and excess. Biosynthesis, Transport, and Metabolism The adrenal cortex produces more than 30 hormones, including aldosterone (mineralocorticoid), cortisol (glucocorticoid), and adrenal androgens. These steroid hormones are synthesized from cholesterol and acetate. Hormones are carried in an unbound state and bind to plasma proteins (e.g., corticosteroid- binding globulin for cortisol). The liver is the main site for metabolizing these hormones, which are then eliminated in the urine or bile. Adrenal Androgens Adrenal androgens, mainly dehydroepiandrosterone (DHEA) and its sulfate (DHEAS), are synthesized by the zona fasciculata and zona reticularis. These hormones have little impact on sexual function but may contribute to pubertal hair growth, especially in women. DHEAS is used for treating Addison's disease. DHEAS levels decrease with age (adrenopause), and its replacement therapy is unproven for aging. Mineralocorticoids Aldosterone, the primary mineralocorticoid, is produced in the zona glomerulosa and plays a crucial role in regulating sodium, potassium, and water balance. It is primarily regulated by the renin-angiotensin system and blood potassium levels. Aldosterone causes sodium retention and potassium excretion in the kidneys, affecting blood pressure and fluid volume. Excess aldosterone leads to low potassium and muscle weakness, while deficiency can cause high potassium and cardiac toxicity. Glucocorticoids Cortisol, the primary glucocorticoid, is produced in the zona fasciculata and regulated by the hypothalamic-pituitary-adrenal (HPA) axis. Cortisol is essential for responding to stress and regulating metabolism, inflammation, and immune function. It stimulates glucose production, breaks down proteins, and mobilizes fatty acids for energy. Glucocorticoids also affect emotional behavior and have significant immunosuppressive and anti-inflammatory effects. These actions are enhanced with pharmacologic doses of cortisol. Pharmacologic Suppression of Adrenal Function Long-term use of glucocorticoid drugs can suppress the HPA system, leading to adrenal atrophy and acute adrenal insufficiency upon abrupt withdrawal. Recovery of normal adrenal function can take up to 12 months or more. Tests of Adrenal Function Several tests assess adrenal cortical function, including: Blood tests for cortisol, aldosterone, and ACTH levels. 24-hour urine tests for cortisol metabolites. Cushing syndrome tests (e.g., late-night cortisol, dexamethasone suppression test). ACTH stimulation test to assess adrenal response. Dexamethasone suppression test to evaluate the HPA feedback system. CRH tests for diagnosing pituitary tumors (Cushing disease). Corticotropin (cosyntropin) stimulation tests for HPA axis responsiveness. These tests help diagnose conditions like Cushing syndrome, adrenal tumors, and pituitary ACTH-secreting tumors. Congenital Adrenal Hyperplasia (CAH) Definition: Congenital adrenal hyperplasia (CAH), also known as adrenogenital syndrome, is a congenital disorder caused by an autosomal recessive trait. It involves a deficiency in any of the enzymes necessary for cortisol synthesis. Causes and Pathophysiology: The primary issue in CAH is a defect in cortisol synthesis, leading to increased ACTH levels. This overstimulates adrenal androgen production, resulting in adrenal hyperplasia. Depending on the enzyme deficiency, mineralocorticoids may be produced in excessive or insufficient amounts. Clinical Manifestations: Male infants: Rarely diagnosed at birth unless there is genital enlargement or signs of salt loss or adrenal crisis. Female infants: Present with ambiguous genitalia, including an enlarged clitoris, fused labia, and urogenital sinus due to excess androgen levels. Secondary sex characteristics typically develop normally, and fertility is usually unaffected with appropriate treatment. Types: 21-Hydroxylase Deficiency: o Ranges from simple virilizing CAH to a salt-losing enzyme deficiency. o In simple virilizing CAH, cortisol synthesis is impaired, and steroid synthesis shifts to androgen production. o In salt-losing CAH, aldosterone production is deficient, leading to fluid and electrolyte imbalances (hyponatremia, dehydration, shock). 11-β-Hydroxylase Deficiency: o Rare and leads to excessive androgen production and impaired conversion of 11-deoxycorticosterone to corticosterone. o The overproduction of 11-deoxycorticosterone results in hypertension due to its mineralocorticoid activity. Diagnosis: Diagnosis is based on biochemical evaluations of cortisol pathway metabolites and genetic testing. However, correlation between phenotype and genotype can be complex. Treatment: Glucocorticoid replacement (oral or parenteral) is standard. Fludrocortisone acetate may be given for salt-losing cases. Surgical intervention may be needed in the first two years of life to reconstruct genitalia in female infants with virilization. Adrenal Cortical Insufficiency Adrenal insufficiency can be classified as primary (Addison's disease) or secondary (due to HPA dysfunction). Primary Adrenal Cortical Insufficiency (Addison's Disease) Definition: Addison's disease is a primary adrenal insufficiency where adrenal cortical hormones are deficient and ACTH levels are elevated due to lack of feedback inhibition. Etiology and Pathogenesis: Autoimmune destruction is the most common cause in the U.S. Tuberculosis, metastatic carcinoma, fungal infections (e.g., histoplasmosis), cytomegalovirus infections, and rare conditions like amyloid disease can also contribute. Bilateral adrenal hemorrhage may occur in cases of trauma or anticoagulant therapy. Clinical Manifestations: Symptoms appear after about 90% of the adrenal cortex is destroyed. Mineralocorticoid deficiency: Causes sodium loss, water loss, and potassium retention. Leads to hyponatremia, dehydration, shock, and orthostatic hypotension. Glucocorticoid deficiency: Results in hypoglycemia, weakness, fatigue, nausea, and vomiting. Hyperpigmentation: Due to elevated ACTH levels, resulting in a bronzed or suntanned appearance on skin and mucous membranes (a distinguishing feature of primary adrenal insufficiency). Treatment: Lifelong hormone replacement therapy is required, typically with hydrocortisone (glucocorticoid) and fludrocortisone (mineralocorticoid) for primary insufficiency. Regular meals and exercise schedules are important to manage hyponatremia and hypoglycemia. Stress management is crucial due to poor stress tolerance. Medical alert bracelet: Recommended for individuals with Addison’s disease for emergency recognition and treatment. Secondary Adrenal Cortical Insufficiency Secondary adrenal insufficiency can occur due to hypopituitarism or the surgical removal of the pituitary gland. Tertiary adrenal insufficiency results from a defect in the hypothalamus. However, a common cause is the rapid withdrawal of glucocorticoids used therapeutically for conditions such as asthma or multiple sclerosis, leading to adrenal atrophy and a loss of cortisol production. This suppression of the hypothalamic-pituitary-adrenal (HPA) system can continue even after discontinuing glucocorticoids, and can be critical during periods of stress or surgery. Acute Adrenal Crisis Acute adrenal crisis is a life-threatening emergency, often triggered by minor illness or stress. It can cause symptoms such as nausea, vomiting, muscle weakness, hypotension, dehydration, and vascular collapse. In cases of congenital adrenal hyperplasia (CAH) with salt-losing forms, adrenal crisis may develop rapidly. Adrenal hemorrhage, caused by conditions such as meningococcal septicemia or anticoagulant therapy, can also result in acute adrenal insufficiency. Management of acute adrenal crisis includes: Salt replacement Sugar (dextrose) replacement Steroid replacement Support of physiological function Identification and treatment of the underlying cause Hydrocortisone or dexamethasone is administered intravenously, and saline and dextrose are used for fluid resuscitation. Mineralocorticoid therapy is added as hydrocortisone is tapered. Glucocorticoid Hormone Excess (Cushing Syndrome) Cushing syndrome results from excessive cortisol production due to various causes: 1. Pituitary adenoma (Cushing disease) 2. Adrenal tumor 3. Ectopic Cushing syndrome from nonpituitary ACTH-secreting tumors 4. Iatrogenic Cushing syndrome due to long-term use of glucocorticoids Clinical Manifestations The major signs of Cushing syndrome include: Fat deposition: moon face, buffalo hump, and abdominal obesity Muscle weakness and wasting Thin skin, purple striae (stretch marks), and osteoporosis Hypertension and hypokalemia due to mineralocorticoid effects Increased susceptibility to infections and gastrointestinal issues (e.g., gastric ulcers) Hirsutism, acne, and menstrual irregularities in women Emotional disturbances, from euphoria to psychotic behavior Diagnosis and Treatment Diagnosis involves testing cortisol secretion, typically by measuring 24-hour urinary cortisol or conducting a dexamethasone suppression test. ACTH levels help differentiate between ACTH-dependent and non-ACTH-dependent causes. Imaging techniques such as MRI or CT scans help locate tumors. Treatment depends on the underlying cause, aiming to remove or treat the source of excess cortisol: Pituitary adenomas are treated with transsphenoidal surgery. Adrenal adenomas may require unilateral or bilateral adrenalectomy. Ectopic ACTH tumors are surgically removed when possible. Pharmacologic treatments (mitotane, ketoconazole, metyrapone) are used for inoperable tumors. Incidental Adrenal Mass (Incidentaloma) An incidentaloma refers to an adrenal mass found unexpectedly during imaging procedures. The key considerations are whether the mass is malignant or hormonally active. Although primary adrenal carcinoma is rare, metastatic cancers, particularly from the lung, can spread to the adrenal glands. Screening tests are conducted to rule out conditions like pheochromocytoma, Cushing syndrome, and Conn syndrome (mineralocorticoid excess). Hormonal Control of Glucose, Fat, and Protein Metabolism The body uses glucose, fatty acids, and other substrates for energy. The liver, in collaboration with hormones from the endocrine pancreas, is responsible for regulating the fuel supply. Glucose Metabolism Glucose is a primary fuel for the body, especially for the brain and nervous system, which rely almost entirely on it. The brain cannot synthesize or store more than a few minutes' worth of glucose, making a continuous blood supply crucial. Severe hypoglycemia can lead to brain death or dysfunction. In healthy individuals, fasting blood glucose levels are tightly regulated between 70-100 mg/dL. After eating, blood glucose rises, triggering insulin secretion. About two-thirds of the glucose is stored as glycogen in the liver, with the remaining converted to fat if the glycogen stores are full. Between meals, the liver releases glucose into the bloodstream to maintain normal levels. When blood glucose drops, glycogen is broken down via glycogenolysis to release glucose. The liver also synthesizes glucose from amino acids, glycerol, and lactic acid through gluconeogenesis, ensuring a steady supply when dietary intake is insufficient. Fat Metabolism Fat is an efficient energy source, providing 9 kcal/g, compared to 4 kcal/g from carbohydrates and proteins. Most dietary carbohydrates are converted to triglycerides and stored in adipose tissue. Triglycerides are made of three fatty acids and glycerol, and are broken down by lipases into these components. The glycerol can enter the glycolytic pathway, while fatty acids are transported to tissues for energy use. Most cells, except those in the brain, nervous tissue, and red blood cells, can use fatty acids for energy. However, fatty acids cannot be converted into glucose, which is required by the brain. The liver plays a significant role in fatty acid degradation, converting them into ketones when large amounts of fatty acids are used. During states like diabetes or fasting, excess ketones are released into the blood, leading to ketoacidosis if present in high amounts. Protein Metabolism Proteins are crucial for building body structures, including genes, enzymes, muscles, bones, and hemoglobin in red blood cells. Amino acids, the building blocks of proteins, are not stored in large quantities as glucose and fat are. Excess amino acids are converted into fatty acids, ketones, or glucose, and stored or used for energy. During periods of low food intake or increased metabolic demands, the body breaks down proteins to provide amino acids for gluconeogenesis (glucose production), as fatty acids cannot be converted into glucose. Glucose-Regulating Hormones The pancreas plays a central role in blood glucose regulation, containing two major tissue types: acini (which secrete digestive juices into the duodenum) and the islets of Langerhans (which secrete hormones into the blood). The islets contain beta cells (which secrete insulin and amylin), alpha cells (which secrete glucagon), delta cells (which secrete somatostatin), and PP cells (which secrete pancreatic polypeptide, involved in digestive functions and potentially regulating feeding behavior). Insulin Insulin is the only hormone known to directly lower blood glucose. Its functions include: 1. Promoting glucose uptake and storage as glycogen. 2. Preventing fat and glycogen breakdown. 3. Inhibiting gluconeogenesis (the production of glucose from non- carbohydrate sources) and stimulating protein synthesis. Insulin facilitates fat storage by increasing glucose transport into fat cells, leading to triglyceride synthesis. It also enhances protein synthesis and inhibits protein breakdown by promoting the active transport of amino acids into cells. Insulin prevents gluconeogenesis when sufficient glucose and insulin are available, minimizing protein breakdown. Insulin is derived from proinsulin, a larger molecule that is modified in beta cells to produce the active form by cleaving the C-peptide structure. This C-peptide can be measured in the blood to assess beta cell function. Insulin secretion is regulated by blood glucose levels, increasing with higher glucose and decreasing as glucose levels fall. The release process occurs in two phases: an initial rapid release of preformed insulin, followed by a sustained release of newly synthesized insulin. Insulin enters the portal circulation, and approximately 50% is used or degraded by the liver. It binds to cell membrane receptors to facilitate glucose uptake. Glucose transport is mediated by specific transporters (e.g., GLUT-4 for skeletal muscle and adipose tissue), which become active upon insulin signaling, enabling glucose entry into cells. Glucagon Produced by alpha cells in the islets of Langerhans, glucagon raises blood glucose levels. It acts primarily on the liver, promoting glycogenolysis (the breakdown of glycogen) to release glucose. Additionally, glucagon stimulates gluconeogenesis, converting amino acids into glucose, which is crucial for maintaining blood glucose levels during fasting or between meals. Glucagon secretion is inversely related to blood glucose levels: it increases when blood glucose drops and decreases when it rises. It also rises during strenuous exercise to prevent hypoglycemia. Amylin, Somatostatin, and Gut-Derived Hormones Amylin is secreted by beta cells alongside insulin. It helps regulate plasma glucose by suppressing glucagon secretion after meals and slowing gastric emptying. Somatostatin, released by delta cells, inhibits the release of both insulin and glucagon and decreases gastrointestinal activity after food intake. Its role in insulin and glucagon regulation is still not fully understood. Incretin hormones are gut-derived hormones that stimulate insulin secretion after oral nutrient intake, especially following carbohydrate-rich meals. These hormones contribute to about 50% of postprandial insulin release and have significant roles in postmeal metabolism. Incretin-based therapies have been explored for improved glycemic control, satiety, delayed gastric emptying, and weight loss. Counter-Regulatory Hormones Counter-regulatory hormones are those that help increase blood glucose levels by counteracting the actions of insulin, especially during fasting, exercise, or situations that deplete glucose stores. These hormones include catecholamines (epinephrine), growth hormone, and glucocorticoids, in addition to glucagon. Epinephrine Epinephrine, also known as adrenaline, is produced by the adrenal medulla during stress and is crucial in maintaining blood glucose levels. It stimulates glycogenolysis (the breakdown of glycogen) in the liver, releasing glucose into the blood. Epinephrine also inhibits insulin secretion from beta cells, reducing glucose uptake by muscle cells while promoting the breakdown of muscle glycogen for muscle energy. Although the glucose from muscle glycogen cannot be released into the blood, its mobilization helps conserve blood glucose for critical tissues like the brain and nervous system. Additionally, epinephrine promotes lipolysis (the breakdown of fat) in adipose tissue, providing fatty acids as an alternative energy source. Epinephrine's action is also important in managing hypoglycemia (low blood sugar). Growth Hormone Growth hormone has several metabolic effects, including: Stimulating protein synthesis in all body cells. Mobilizing fatty acids from adipose tissue. Antagonizing insulin actions, thus increasing blood glucose levels. The most significant role of growth hormone is promoting longitudinal growth by stimulating bone and cartilage formation. Growth hormone levels rise during fasting, exercise, and stress. In contrast, high levels of blood glucose and insulin typically inhibit its secretion. Chronic overproduction of growth hormone, as seen in conditions like acromegaly, can lead to glucose intolerance and the development of diabetes mellitus (DM). Growth hormone secretion follows a pulsatile pattern, peaking during childhood and puberty, and gradually declining with age. Glucocorticoid Hormones Glucocorticoid hormones, primarily cortisol, are produced by the adrenal cortex and are essential for survival during fasting and starvation. Their main role is to increase blood glucose levels by promoting gluconeogenesis (the production of glucose from non-carbohydrate sources) in the liver. Glucocorticoids also have anti-inflammatory effects and modulate the immune response. Stress, whether physical or emotional, triggers an increase in adrenocorticotropic hormone (ACTH) from the anterior pituitary, which in turn stimulates the release of cortisol. Cortisol secretion is also triggered by hypoglycemia (low blood sugar). Synthetic glucocorticoids like prednisone are commonly used to treat inflammatory diseases but can lead to elevated blood glucose levels as a side effect. Together, these hormones work to increase blood glucose during periods of stress, fasting, or physical exertion, ensuring that energy is available for critical body functions. Diabetes Mellitus (DM) Diabetes mellitus (DM) is a group of metabolic disorders characterized by hyperglycemia (high blood glucose levels). It occurs due to an imbalance between insulin availability and the body's need for insulin. Factors such as reduced insulin secretion, decreased glucose utilization, and increased glucose production contribute to this imbalance. Without proper management, diabetes results in the body's inability to transport glucose into fat and muscle cells, leading to fat and protein breakdown for energy. Historically, diabetes was fatal before the discovery of insulin in the 1920s. Today, type 2 diabetes is the most common form and is rising significantly due to increasing rates of obesity, aging populations, and decreasing mortality. The disease affects the metabolism of carbohydrates, proteins, and fats. Classification and Etiology Diabetes is not a single disease but has several forms, primarily: Type 1 Diabetes: Characterized by beta-cell destruction, leading to absolute insulin deficiency. o Immune-mediated (autoimmune destruction of insulin-producing cells). o Idiopathic (unknown causes). Type 2 Diabetes: Characterized by insulin resistance and relative insulin deficiency. This form accounts for about 90-95% of diabetes cases. Other Specific Types: o Genetic defects affecting insulin production or action (e.g., maturity onset diabetes of the young, type A insulin resistance). o Endocrinopathies (e.g., Cushing disease, acromegaly). o Diseases of the pancreas (e.g., pancreatitis). o Drug or chemical-induced (e.g., glucocorticoids). o Infections (e.g., cytomegalovirus, rubella). o Genetic syndromes (e.g., Down syndrome, Turner syndrome). Gestational Diabetes Mellitus (GDM): Develops during pregnancy and typically resolves postpartum. Pre-Diabetes (Impaired Glucose Tolerance & Impaired Fasting Glucose) Individuals with impaired glucose tolerance (IGT) or impaired fasting glucose (IFG) are classified as having prediabetes, where their glucose levels are higher than normal but not yet meeting the criteria for diabetes. People with prediabetes are at increased risk for developing type 2 diabetes and atherosclerotic heart disease. Impaired Glucose Tolerance (IGT): Plasma glucose levels between 140 to 199 mg/dL (7.8 to 11.0 mmol/L) during a 2-hour oral glucose tolerance test (OGTT). Impaired Fasting Glucose (IFG): Fasting plasma glucose levels between 100 to 125 mg/dL (5.6 to 6.9 mmol/L). Interventions such as weight reduction, physical activity, and calorie reduction are recommended for individuals with prediabetes to prevent progression to diabetes. Criteria for Diagnosis of Diabetes Mellitus (DM) Diabetes can be diagnosed using any of the following criteria: 1. HbA1c ≥ 6.5%. 2. Fasting plasma glucose (FPG) ≥ 126 mg/dL (7.0 mmol/L). Fasting is defined as no caloric intake for at least 8 hours. 3. 2-hour plasma glucose ≥ 200 mg/dL (11.1 mmol/L) during an OGTT. 4. Random plasma glucose ≥ 200 mg/dL (11.1 mmol/L) in a person with classic symptoms of hyperglycemia or hyperglycemic crisis. In the absence of unequivocal hyperglycemia, criteria 1 and 2 should be confirmed by repeat testing. Type 1 Diabetes Mellitus Type 1 Diabetes Mellitus (DM) is a condition marked by the destruction of pancreatic beta cells. It can be divided into two subtypes: Type 1A (immune- mediated) and Type 1B (idiopathic). In regions like the U.S. and Europe, Type 1A accounts for 90-95% of cases. Type 1B occurs less frequently and lacks an autoimmune cause. Type 1A Immune-Mediated Diabetes Type 1A, commonly known as juvenile diabetes, is an autoimmune condition in which T-lymphocytes attack beta cells in the pancreas. This form is more common in youth but can affect individuals of any age. The speed of beta cell destruction can vary, being rapid in some cases (often seen in children) and slow in others (more common in adults). This form is characterized by a complete lack of insulin, high blood glucose levels, and breakdown of fats and proteins. Without insulin, lipolysis (fat breakdown) is unregulated, leading to ketone production and diabetic ketoacidosis. Therefore, people with Type 1A require insulin therapy to manage blood glucose levels and prevent ketosis. The development of Type 1A diabetes is believed to result from a combination of genetic predisposition and environmental factors like infections. A major susceptibility gene is located in the HLA region on chromosome 6. Family history increases the risk, but the overall risk is still relatively low. Early detection of Type 1A is possible through the identification of autoantibodies against insulin, glutamic acid decarboxylase (GAD), and IA-2. In some cases, these autoantibodies appear long before hyperglycemia. Type 1B Idiopathic Diabetes Type 1B diabetes lacks the autoimmune component seen in Type 1A. It is less common and occurs without a clear cause. The treatment approach for both subtypes is similar. Type 2 Diabetes Mellitus Type 2 DM is the most common form, accounting for 90-95% of all diabetes cases. It is characterized by insulin resistance, where the body’s cells (especially muscle, liver, and fat cells) do not respond effectively to insulin. As a result, blood glucose levels remain elevated, and the body produces more insulin to compensate. Over time, this leads to beta cell exhaustion and progressive failure. Unlike Type 1, where there is an absolute lack of insulin, Type 2 can involve normal, low, or even elevated insulin levels. Type 2 diabetes is strongly influenced by genetics, with a familial predisposition. People with one affected parent have an increased risk, and if both parents have diabetes, the risk is higher (around 40%). The main metabolic issues that lead to Type 2 diabetes include: 1. Insulin Resistance: Impaired ability of insulin to work on tissues. 2. Beta Cell Dysfunction: Insufficient insulin secretion due to overexertion of the pancreas. 3. Increased Glucose Production: The liver overproduces glucose despite high insulin levels. Obesity, particularly central obesity (abdominal fat), is closely linked to insulin resistance. Around 80-90% of individuals with Type 2 diabetes are overweight. Central obesity, measured through waist circumference and waist-to-hip ratio, is a strong indicator of insulin resistance. Insulin resistance also contributes to metabolic syndrome, which includes other conditions like high blood pressure, high triglycerides, low HDL cholesterol, and an increased risk of cardiovascular diseases. Excessive free fatty acids (FFAs), often seen in obesity, worsen insulin resistance and can cause beta cell dysfunction (lipotoxicity). This leads to elevated glucose production in the liver and higher blood glucose levels. Metabolic Syndrome and Obesity The insulin resistance syndrome, or metabolic syndrome, includes conditions like obesity, high triglycerides, low HDL cholesterol, hypertension, and an increased risk of cardiovascular disease. Insulin resistance plays a significant role in these conditions, often leading to Type 2 diabetes. Treatment Approach Management of Type 2 diabetes includes addressing obesity and other metabolic abnormalities. Weight loss (5-10% of body weight) can significantly improve insulin sensitivity and help manage diabetes. Pharmacological treatment, lifestyle changes like diet and exercise, and in some cases, insulin therapy may be required to control blood glucose levels. Other Specific Types of Diabetes Pancreatic Disease and Endocrine Disorders: Diabetes can also occur as a result of diseases affecting the pancreas, such as pancreatic disease or the removal of pancreatic tissue. Additionally, endocrine disorders like acromegaly, Cushing syndrome, and pheochromocytoma can contribute to hyperglycemia. These conditions typically increase hepatic glucose production or decrease cellular glucose utilization. Monogenetic Defects: There are cases of diabetes caused by specific monogenetic defects affecting beta cell function or insulin secretion. Drug-Induced Diabetes: Certain medications can alter glucose levels and induce diabetes, including diuretics (thiazide and loop), diazoxide, glucocorticoids, oral contraceptives, antipsychotics, and total parenteral nutrition. These drug-related changes in blood glucose are generally reversible once the medication is discontinued, though long-term use of some medications may require ongoing glucose management. Gestational Diabetes Mellitus (GDM) GDM is a condition of glucose intolerance occurring during pregnancy. It affects around 7% of pregnancies, with higher prevalence in certain populations (e.g., African American, Hispanic, American Indian women). Risk factors include: Family history of diabetes Previous stillbirth or spontaneous abortion History of fetal anomalies or large infants Obesity Advanced maternal age Multiple previous pregnancies Diagnosis: GDM is diagnosed through a 75g Oral Glucose Tolerance Test (OGTT) between 24-28 weeks of pregnancy. If any of the following glucose levels are exceeded, a diagnosis of GDM is made: Fasting: >92 mg/dL (5.1 mmol/L) 1-hour: >180 mg/dL (10.0 mmol/L) 2-hour: >153 mg/dL (8.5 mmol/L) New guidelines based on the HAPO study aim to provide better glycemic control and improve maternal and fetal health outcomes. Treatment: GDM treatment focuses on regular monitoring of maternal and fetal health, nutritional guidance, and, if necessary, medication (insulin or oral agents like glyburide). Close surveillance is crucial due to the increased risks of complications such as macrosomia, hypoglycemia, and other fetal abnormalities. Women with GDM have a 35% to 65% chance of developing type 2 diabetes within 20 years, so post-delivery follow-up is essential for early detection of diabetes. Summary: GDM requires careful diagnosis and management, with the goal of maintaining normal glucose levels to prevent maternal and fetal complications. Clinical Manifestations of Diabetes Mellitus The onset of diabetes mellitus (DM) can be rapid, especially in type 1 diabetes, or more insidious, often in type 2 diabetes, which may go unnoticed for years. The most common signs and symptoms of diabetes are referred to as the "three polys": 1. Polyuria (excessive urination) 2. Polydipsia (excessive thirst) 3. Polyphagia (excessive hunger) These symptoms are related to hyperglycemia and glycosuria. As blood glucose levels rise, the kidneys cannot reabsorb all the glucose, resulting in glucose being excreted in the urine (glycosuria), which pulls water from the body, causing polyuria. This, in turn, leads to dehydration and excessive thirst (polydipsia). Polyphagia in type 1 diabetes occurs due to cellular starvation when the body is unable to utilize glucose, causing a depletion of carbohydrate, fat, and protein stores. Weight Loss in Type 1 Diabetes: Weight loss, despite normal or increased appetite, is common in uncontrolled type 1 diabetes. This occurs because osmotic diuresis leads to fluid loss, and the lack of insulin forces the body to use fat and muscle for energy. Type 2 diabetes may also present with unexplained weight loss if insulin is not being properly utilized, though obesity is more common in this type. Other Symptoms: Other signs of hyperglycemia include recurrent blurred vision, fatigue, and frequent skin infections. Blurred vision occurs as hyperosmolar fluids affect the eye lens and retina. Fatigue results from lowered plasma volume. Skin infections, including Candida infections, are more common in people with type 2 diabetes due to favorable conditions for yeast growth from hyperglycemia and glycosuria. Diagnostic Tests Diagnosis of diabetes mellitus is confirmed through laboratory tests measuring blood glucose levels (see Table 50.2). Testing is recommended for: People aged 45 or older Individuals who are obese Those with a first-degree relative with diabetes People from high-risk groups (e.g., certain ethnicities) Women who have delivered an infant weighing more than 9 lbs or have had gestational diabetes mellitus (GDM) Individuals with hypertension or hyperlipidemia Those previously diagnosed with impaired glucose tolerance (IGT) or impaired fasting glucose (IFG, also known as prediabetes). Blood Tests for Diabetes Diagnosis and Management Blood glucose measurements play a crucial role in diagnosing and managing diabetes. Several diagnostic tests and monitoring methods are used: 1. Fasting Blood Glucose Test (FPG): o This test measures glucose levels after an 8-hour fast. o A normal level is below 100 mg/dL (5.6 mmol/L). o Levels between 100-125 mg/dL (5.6-6.9 mmol/L) indicate impaired fasting glucose (IFG). o A level of 126 mg/dL (7.0 mmol/L) or higher confirms diabetes. 2. Casual Blood Glucose Test: o This test measures blood glucose without regard to the time of the last meal. o A casual plasma glucose level ≥200 mg/dL (11.1 mmol/L) in the presence of classic diabetes symptoms (e.g., polydipsia, polyphagia, polyuria, blurred vision) is diagnostic of diabetes. 3. Oral Glucose Tolerance Test (OGTT): o This test measures the body's ability to store glucose by removing it from the blood after ingesting a 75g glucose solution. o Blood glucose is measured at 1- and 2-hour intervals. o In normal individuals, blood glucose returns to normal within 2-3 hours. In people with diabetes, blood glucose levels remain elevated longer. 4. Capillary Blood Glucose Monitoring: o Self-monitoring of blood glucose using a small drop of capillary blood obtained from a finger or forearm. o The blood is placed on a reagent strip and glucose levels are measured using a glucose meter. o Although more convenient, readings from capillary blood are 10-15% lower than those from plasma. This method is essential for maintaining near-normal blood glucose levels. 5. Continuous Glucose Monitoring (CGM): o This technology uses small subcutaneous catheters to provide frequent glucose readings, helping to fine-tune glucose management. o Although it is growing in use, finger-stick glucose monitoring remains the standard of care. 6. Glycated Hemoglobin (A1C) Test: o A1C reflects average blood glucose levels over the past 6-12 weeks. o It is used to assess long-term glycemic control and diagnose diabetes. o An A1C level above 6.5% is diagnostic of diabetes. Levels above 7% suggest poor control, and the goal is to lower A1C to less than 7.0%. o A higher A1C correlates with increased risk of complications. Urine Tests Urine tests for glucose are no longer commonly used due to the advancement in blood glucose monitoring techniques. These tests are influenced by factors such as renal threshold for glucose, fluid intake, and urine concentration. However, urine ketone tests are important, particularly in people with type 1 diabetes who are at risk for ketoacidosis and in pregnant women with diabetes to assess nutritional adequacy and glycemic control. Diabetes Treatment Treatment Goals Aim: Normalize blood glucose to prevent short- and long-term complications. Type 1 Diabetes: Insulin therapy from diagnosis. Type 2 Diabetes: Lifestyle changes (diet, exercise); oral antidiabetic agents if needed. Components of Treatment 1. Medical Nutrition Therapy (MNT): o Definition: Tailored use of nutrition for disease management (assessment, counseling, supplements). o Goals: Maintain near-normal blood glucose. Achieve optimal lipid levels and weight. Prevent and manage complications. o Type 1 Diabetes: Adjust insulin to food intake; consistent meals. o Type 2 Diabetes: Focus on weight loss (5-10%) and cardiac risk factors. o Dietary guidelines: Carbohydrates: 45–60% of total calories. Fats: 20–35%, with