Canadian Institute for NDE EMC Online Manual 2021-2 PDF
Document Details
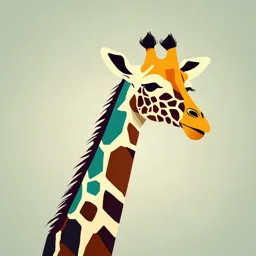
Uploaded by CreativeLogic7024
2021
Tags
Summary
This document is a manual detailing various mechanical testing procedures for materials, especially metals. It describes different testing methods and examines aspects like compressive strength, bend tests, and hardenability. The focus is on practical applications and standards, likely used in industrial or professional settings.
Full Transcript
CANADIAN INSTITUTE FOR NDE Section 2 Compressive Strength Testing Compressive testing is usually conducted in the same apparatus as tensile testing, the loading is reversed and the specimen (usually cylindrical) is shortened by the loading. The...
CANADIAN INSTITUTE FOR NDE Section 2 Compressive Strength Testing Compressive testing is usually conducted in the same apparatus as tensile testing, the loading is reversed and the specimen (usually cylindrical) is shortened by the loading. The compressive strength of the material is determined by the compressive stress when the metal fails. Guided Bend Tests Bend tests are employed to confirm the acceptability of a welding procedure specification, or a welder’s ability to complete a specific procedure. The specification will determine the number and style of bend tests to be performed. The outer surface of the bend is put into tension as a result of the bending action. If the root of the weld is put into tension it is referred to as a root bend, if the crown or face of the weld is put into tension then it is referred to as a face bend, refer to figure 2.0u. On thicker sections, particularly when the weld is made from both faces, a cross section of the weld is taken and the bend is against the cross section. This is referred to as a side bend. Ideally the base material, weld deposit and the heat affected zones of the weld have similar deformation properties otherwise stress is concentrated in one area of the specimen as it is being bent. When the weld deposit is significantly stronger than the base metal a flat spot will occur at the centre of the weld. Figure 2.0u, See figure 2.0s. Weld Coupon Bend Tests Figure 2.0s Figure 2.0t Engineering, Materials and Components Revision Date: 9/17/19 - 75 - CANADIAN INSTITUTE FOR NDE Section 2 When the base material is significantly stronger than the weld material the bending stress will concentrate in the weld and a “dog house“ occurs with the weld failing (see figure 2.0t). Stress raisers and notches in the specimen can cause failure and for that reason the crown of the welds are usually removed by grinding such that the direction of grinding is parallel to the direction of stress applied by the bending test. Defects in the weld will also open and crack indicating a failure of the test. Figure 2.0v, Weld Bend Test Jig Figure 2.0w, Weld Bend Test Jig Figures 2.0v and 2.0w show two of the jigs employed. The dimension A is governed by the tensile strength of the material which is given in the specification as is the acceptance criteria used when visually examining the specimens. Determining Hardenability of Steels Hardenability is a measure of the depth to which steel will harden when quenched from its austenitizing temperature. ASTM A255 describes the Jominy End Quench Test for determining the hardenability of steels with a carbon range of 0.10 to 0.70% carbon (and other chemistry restrictions). It employs a specimen 25.4 mm in diameter by 101.6 mm long, with a small shoulder on one end. The specimen is heated in a furnace to its austenitizing temperature and holding it for 30 minutes. The end of the specimen is quenched in a stream of water (5° to 30° C). Two flats 180 degrees Engineering, Materials and Components Revision Date: 9/17/19 - 76 - CANADIAN INSTITUTE FOR NDE Section 2 apart are ground to a depth of.38mm, along the entire length of the specimen. Rockwell C hardness readings are taken along the length of the bar on both flats. The hardness results are plotted against the distance from the quenched end of the bar and calculations made to determine the depth of hardening, see figure 2.0x. Figure 2.0x, Jominy End Quench Test Results Engineering, Materials and Components Revision Date: 9/17/19 - 77 - CANADIAN INSTITUTE FOR NDE Section 2 Other Mechanical Testing Methods Adhesion Testing This testing method is used to measure resistance to peeling, shearing, delaminations and frictional properties of surfaces under different conditions. There is not a lot of standardization in this type of testing and most test methods are developed for specific applications. Friction Testing Measures the response of materials in certain environmental conditions, geometries and contact pressures to reproduce relative motion between paired materials. ASTM standards have been developed to provide guides in testing procedures. Wear and Surface Damage Testing The test method is chosen based on the type of wear or surface damage being considered. Refer to section 4.3 of this manual dealing with wear and abrasion for a further understanding of the types of damage that can occur. Creep and Stress-Relaxation Testing Creep properties are important for materials that are stressed at elevated temperatures for long periods of time. Creep strain tests measure the amount and evaluate the deformation that occurs over time. Creep rupture tests measure the amount of time to fracture at given stress levels and temperature. Stress relaxation occurs when the strain is held constant and the elastic properties cause a decrease in the stress. This type of test might be used to evaluate the performance of materials used for high temperature bolting, where the bolts will tend to loose their clamping force because of stress-relaxation. References: 1. University of Maryland, Material Hardness, www.calce.umd.edu/general/Facilities/Hardness_ad_.htm#rf05" 2. GE Sensing & Inspection Technologies, DynaMIC, http://www.geinspectiontechnologies.com/en/products/hardness_testers/dynamic.html, DynaMIC is a registered trademark of GE Sensing & Inspection Technologies. 3. Proceq Group. Equotip, www.corvib.com/equotip/, Equotip is a registered trademark of Proceq SA 4. TWI, The Welding Institute, Bend Tests 5. ASTM A255-07, Standard Test Methods for Determining Hardenability of Steel 6. Wikipedia, http://en.wikipedia.org/wiki/Main_Page 7. ASM Handbook, Volume 8, Mechanical Testing and Evaluation Engineering, Materials and Components Revision Date: 9/17/19 - 78 - CANADIAN INSTITUTE FOR NDE Section 3 3.0 MANUFACTURING PROCESSES Manufacturing processes are used to transform raw materials into finished goods. 3.1 Heat Treatment of Steels Stress Relief Stress relief heat treating of steel is accomplished by heating the steel to just below the transformation temperature, holding this temperature for a predetermined period of time and then uniformly cooling the component. Stress relief can be used to improve the fracture toughness of heat affected zones (HAZ) of welds, improve resistance to corrosion and stress corrosion cracking, or reduce the likelihood of brittle failure. Stresses may have been introduced into the part by welding, heating, grinding, bending or physical working of the component. Annealing Annealing of steel involves heating the steel and slow cooling to soften the steel. Annealing is often employed to facilitate machining, cold working, improve mechanical or electrical properties or improve dimensional stability. There are several varieties of annealing: Full Anneal Full Annealing is conducted by heating hypo eutectoid steels 55o C above the upper critical temperature and 55o C Figure 3.1a, Annealing above the lower critical temperature Temperatures hyper eutectoid steels (refer to the equilibrium diagram in figure 3.1a) holding and then slow cooling in the furnace. Full annealing refines the grain, softens the steel, improves electrical and magnetic properties and in some cases improves machinability. Process Anneal Process Annealing is used for sheet and wire steel product after cold working. It softens the steel for further working and is very similar to subcritical annealing in that the steel is heated to just below the lower critical temperature. Subcritical Annealing (stress-relief annealing) Engineering, Materials and Components Revision Date: 9/17/19 - 79 - CANADIAN INSTITUTE FOR NDE Section 3 Subcritical Annealing is used to remove residual stresses due to heavy machining or cold working processes. Heating is carried out to just below the lower critical temperature. Spheroidizing Spheroidizing is performed on steels to improve the cold formability. One way of achieving this would be heating just below the upper critical temperature for a prolonged period of time and then slow cooling in the furnace. The structure that is achieved is spheroids (globules) of carbides in a ferrite matrix. The soft ductile matrix allows Figure 3.1b, for cold forming of the steel. Spheroids Temperature [°C] 1130 908 Normalizing Normalizing is used to produce a harder, stronger 723 steel (compared to full annealing). Normalizing refines the grain, makes the steel more homogenous and improves the machinability particularly in low carbon steels. The steel is heated to about 55o C.8 2.0 above the upper critical temperature followed by slow Percent Carbon by Weight cooling in still air to room temperature. The pearlite Figure 3.1c, Normalizing structure is finer than full annealed. Temperatures Hardening Hardening of carbon, low alloy and tools Temperature [°C] 1130 steels is accomplished by heating to eliminate all the ferrite (refer to the 908 equilibrium diagram in figure 3.2d). The material is then quenched to produce 723 controlled amounts of martensite. The most common materials used to quench the steel maybe: Oil (with additives) Water.8 2.0 Brine Percent Carbon by Weight o Water with salt addition Figure 3.2d, Gases Hardening Temperatures o Helium, Argon, Nitrogen Engineering, Materials and Components Revision Date: 9/17/19 - 80 - CANADIAN INSTITUTE FOR NDE Section 3 Hardness of the steel depends on several factors including the composition of the steel, what quenching medium and how it is used. In using equilibrium diagrams one of the limiting factors is that the rate of temperature change must be very slow. When considering rapid changes in temperature, particularly when considering quenching for the purpose of hardening, we must consider a Time Temperature Transformation Diagram. Refer to figure 3.2e. When the steel is heated to form austenite and then rapidly cooled martensite is formed. If the steel is cooled very slowly, as we discussed when considering equilibrium diagrams, then the Time Temperature Transformation Diagram shows the formation of a pearlitic structure as we saw from the equilibrium diagram. A third option is to rapidly quench the steel to below the knee of the Time Temperature Transformation Diagram and then hold the temperature to avoid the formation of both pearlite and martensite. The resulting structure is Bainite. Martensite is not an equilibrium phase in steels. Its crystalline structure is called body centered tetragonal, its hardness and shape depends on the carbon content of the steel. Figure 3.2e, Time Temperature Transformation Diagram Engineering, Materials and Components Revision Date: 9/17/19 - 81 - CANADIAN INSTITUTE FOR NDE Section 3 Tempering Tempering is a process that follows hardening to increase ductility and toughness, increase grain size, and retain much of the hardness achieved in the hardening process. Tempering variables include: Tempering temperature Time at temperature Cooling rate from the tempering temperature Composition of the steel The process involves heating the steel to below the lower critical temperature, holding at that temperature and then cooling at a suitable rate. Tempering reduces the stresses setup during quenching and improves dimensional stability of the component. Cold Treating of Steel The best structure is achieved if during the hardening process there is complete transformation from austenite to martensite before tempering occurs. If immediately after quenching the temperature of the steel is lowered to -84°C and held 1 hour per 25mm of thickness, then greater dimensional stability, improved wear resistance and reduced residual stress can be achieved. The component is then tempered. In some applications particularly those alloys subject to cracking, the component is tempered after quenching, then cold treated, and then re- tempered to achieve the desired properties and avoid cracking. In variations of cold treating steels cryogenic temperatures are used. Surface Hardening Surface hardening (case hardening) is used to improve the wear resistance of the surface without affecting the tough interior. Often done to achieve a hard surface with good wear resistance properties and still retain a tough interior to resist impact. Gear teeth are often surface hardened. There are a significant number of ways of increasing the hardness of the surface layer of steels: Flame Surface Hardening Flame hardening is used when the part is too large to fit into available furnaces or only a small segment of the surface is to be hardened. Generally used on medium carbon steels, medium carbon alloy steels, some cast irons and lower alloy tool steels. The outer layer is heated by an oxy-fuel flame to the austenite range and then quenched to form an outer martensitic layer. Typical hardened layers are from 1 to 6 mm deep. Induction Surface Hardening A process similar to flame hardening except that the surface layer is heated by electromagnetic induction. Electrical frequencies greater than 1000 Hz are used, case depths can vary from.25 mm to 6 mm. Engineering, Materials and Components Revision Date: 9/17/19 - 82 - CANADIAN INSTITUTE FOR NDE Section 3 Laser Surface Hardening Case depths of.25 to 2 mm can be achieved in medium carbon steel using lasers as the source of heat and self cooling by conduction of heat by the mass of the component. Electron Beam Surface Hardening As with laser hardening, electron beam utilizes self cooling, but generates heat through impact of high speed electrons with the surface. Another group of surface hardening methods are referred to as diffusion methods and rely on changing the chemical composition of the outer layer by diffusing carbon, nitrogen or boron into the surface. These methods include: Carburizing By heating the component to a temperature of 850°C or above in a carbon controlled gaseous atmosphere carbon is taken into the austenitic surface layer, subsequent quenching forms a martensitic case. The source of carbon is from methane, propane or butane. The outer layer can be further tempered if required. Nitriding A process that does not rely on carbon but instead nitrogen is introduced into the surface by heating the component to between 495°C to 565°C in an ammonia atmosphere. Steels to be subjected to Nitriding usually contain.85% to 1.5% aluminum alloy, although chromium alloys can also form nitrides. Nitriding can also be done in liquid molten salt baths usually at a lower temperature than gaseous nitriding. Carbonitriding Boriding Titanium-carbon diffusion Toyota diffusion process Engineering, Materials and Components Revision Date: 9/17/19 - 83 - CANADIAN INSTITUTE FOR NDE Section 3 Stainless steels, super alloys and nonferrous metals and their alloys are routinely heat treated to achieve desired properties. A few terms to keep in mind: Aging Aging is a change in properties that occurs in some metals at ambient or moderately elevated temperatures after hot working or heat treatment. It usually occurs because of a phase change. Bright Annealing An annealing process which is done in an atmosphere to prevent discoloration of a surface, typically stainless steel. Quench Crack A crack which forms during quenching, usually in carbon, alloy or tools steels, originating from changes in section. Recrystallization The formation of a new structure, with reduced strain from previous cold working. Usually achieved by heating. Sensitization Austenitic stainless steels when heated, where chromium carbides have formed leaving the grain boundaries depleted of chromium and susceptible to corrosive attack. Solution Heat Treatment Heating an alloy and holding at a suitable temperature allowing one or more constituents to go into solution. Strain Aging Aging after plastic deformation. Toughness Ability of a material to absorb energy and be plastically deformed. Transformation Temperatures The temperature at which a change in phase occurs. References: 1. ASM, Metals Handbook, Volume 4, Heat Treating 2. ASM, Engineering Materials Handbook Engineering, Materials and Components Revision Date: 9/17/19 - 84 - CANADIAN INSTITUTE FOR NDE Section 3 3.2 Welding, Brazing, and Soldering Table 3.2a – Comparison of Welding, Brazing and Soldering Parameter Soldering Brazing Welding Joint Formed Mechanical Metallurgical Metallurgical Filler metal 450°C but less >450°C less than melting than the melting or equal to the temperature temperature of the melting base metal temperature of the base metal Fluxes Required Optional Optional Base Metal Does not melt Does not melt Melts Residual Stresses Not likely Not likely Likely around the weld Brazing and Soldering Brazing employs a higher temperature than soldering but the concepts are similar. Brazing is accomplished by: 1. Assembling the parts and heating the joint to at least 450°C 2. The filler metal melts 3. The molten filler metal flows into the joint by capillary action and wets the base metal surfaces. 4. The parts are cooled and the filler metal freezes by metallurgical reaction, an atomic bonding occurs. In comparing welding to brazing, virtually no base metal melting occurs in brazing as it does in welding. It therefore has less thermally induced distortion because the entire part can be brought up to the same temperature. Ceramics can be joined to metals and dissimilar metals can be joined that cannot normally be with welding processes. Brazing lends itself to mass production and can be less operator dependent than some welding methods. Brazing does introduce a heat affected zone (HAZ) in the base material. In general terms the HAZ is wider and less sharply defined than with other fusion processes. Capillary action of the joint is critical to draw in the molten filler material and cause wetting of the faying surfaces of the joint. Just as in the study of liquid penetrants the contact angle of the molten filler material to contact surfaces is critical. At contact angles of 90° no wetting takes place as the angle decreases the wetting improves. Other important considerations are the formation of oxide films on the joint surfaces as well as the surface roughness. Engineering, Materials and Components Revision Date: 9/17/19 - 85 - CANADIAN INSTITUTE FOR NDE Section 3 Many filler metals are available for brazing. They include pure metals such as silver and gold. Alloys of silver are often employed as well as alloys of nickel, copper, aluminum, and magnesium. The critical variables in producing sound brazed joints include: 1. Filler metal flow 2. Base metal characteristics 3. Filler metal characteristics 4. Surface preparation 5. Joint design and clearance 6. Temperature and time 7. Rate of heating 8. Protection from the atmosphere Heating methods include: 1. Torch 2. Electrical resistance 3. Induction 4. Furnace with atmosphere Figure 3.2a, 5. Furnace under vacuum Induction Brazing6 6. Dip in molten brazing filler metal 7. Infrared 8. Exothermic 9. Electron beam 10. Laser 11. Microwave The term braze welding is used to describe a process in which the filler metal temperature must be above 450°C to achieve joints such as fillets or groove braze welds. It is not dependent on capillary action and therefore joint spacing is less critical. Often completed with an oxy-fuel torch, but it can also be completed with gas tungsten gas metal arc equipment. It can be used to join materials such as cast iron. It can achieve weld strengths that meet or exceed some base metals. Soldering Soldering has been in use for over 5000 years, original filler metals consisted of two parts lead and one part tin. Scientific studies show that at the interface of the solder to the base metal there is metallurgical reaction but the principle bonding is adhesive and mechanical in nature. As with brazing the ability of the filler metal to wet the surface of the base metal is critical to the process. Engineering, Materials and Components Revision Date: 9/17/19 - 86 - CANADIAN INSTITUTE FOR NDE Section 3 Soldering (and brazing) processes consist of a cleaning cycle, filler metal addition and a heating and cooling cycle. The critical variables associated with soldering include: Temperature Time Vapour pressure Metallurgical and chemical nature of the surfaces Geometry of the surfaces Some metals such as copper, gold, silver, platinum and tin are easy to solder. Some metals such as aluminum and its alloys are very difficult to solder and some metals such as beryllium and titanium cannot be soldered. The base metal(s) to be joined determine the flux and filler metal to be used. Typical examples: Table 3.2b Base Metal Filler Metal Flux Type Steel 40% Sn / 60% Pb CO Copper Sn / Pb NC / CO Stainless Steel > 50% Sn / Pb CO Cast Iron Sn / 20-50% Pb CO Lead 40% Sn / 58% Pb / 2% NC Sb Magnesium Cd / Zn / Sb Notes: Sn = tin, Pb = lead, Sb = antimony, Zn = zinc, CO = corrosive inorganic flux, NC = noncorrosive inorganic flux. Filler metals and fluxes are specified by AWS (American Welding Society). The methods of heating that can be employed when soldering include: soldering iron, torch, dip, induction, resistance, furnace, ultrasonic, focussed infrared radiation, hot gas, wave soldering, vapour phase condensation and laser beam. Fusion Welding Processes Fusion welding encompasses a number of different processes which involves localized melting of the base materials to be joined. The processes may involve the addition of filler metal. For the purpose of this manual, reference is made to the AWS (American Welding Society) electrode designations. The Canadian Standards Association (CSA) publishes standard W48, Filler Metals and Allied Materials for Metal Arc Welding. There are several differences in the systems: Engineering, Materials and Components Revision Date: 9/17/19 - 87 - CANADIAN INSTITUTE FOR NDE Section 3 AWS is a more complete system of consumables than the CSA system which is primarily steel welding consumables, while AWS covers other materials. The CSA system uses the SI system of units as an example AWS E7018 is equivalent to CSA E4918. The first two numbers (70) in the AWS system represent 70ksi (70,000 psi as deposited tensile strength) in the CSA system 49 represents 490 MPa as deposited tensile strength. The other major difference is that CSA electrodes are certified by CWB (Canadian Welding Bureau) as meeting the standard. The AWS designation does not mandate a certification. Most electrodes certified to CSA W48 carry the AWS designation as well as the CSA designation. Shielded Metal Arc Welding (SMAW) Often referred to as stick or covered electrode, Shielded Metal Arc welding involves a manual process in which an arc is created between a flux covered consumable electrode and base metal to be welded. The flux coating provides a variety of functions including: generating a shielding gas, providing fluxing elements to protect the molten puddle and the droplets, provide alloying to the joint, and control the rate of cooling. Figure 3.2b provides a depiction of the process. The process can be applied to base metals thicker than 1.6 mm and in all positions (flat, vertical, horizontal and overhead). The equipment used provides voltages in the range of 15 to 35 volts during the welding process Figure 3.2b, Shielded Metal Arc Welding7 and is referred to as a constant current power supply as it endeavours to provide constant current under varying conditions to provide a stable arc. The power supply will usually be either: transformer; generator or alternator; or inverter. Welding can take place with AC, direct current electrode negative (DCEN or “straight polarity”) or direct current electrode positive (DCEP or “reverse polarity”). The process is primarily used to join steels although it can be used for many metals. Engineering, Materials and Components Revision Date: 9/17/19 - 88 - CANADIAN INSTITUTE FOR NDE Section 3 Several common designations for the electrodes by AWS (American Welding Society): 1. AWS A5.1 Specification for Carbon Steel Electrodes for Shielded Metal Arc Welding. 2. AWS A5.3 Specification for Aluminum and Aluminum alloy Electrodes for Shielded Metal Arc Welding 3. AWS A5.4 Specification for Stainless Steel Electrodes for Shielded Metal Arc Welding 4. AWS A5.5 Specification for Low Alloy Steel Electrodes for Shielded Metal Arc Welding AWS 5.1 (Specification for Carbon Steel Electrodes for Shielded Metal Arc Welding) designation employs the letter “E” for electrode and four digits. The first two digits indicate the nominal as deposited tensile strength of the weld, in units of ksi (1000 pounds per square inch). The next (third) single digit indicates the position in which they can be used: 1. All positions 2. Flat welds and horizontal fillets only 3. Not currently used 4. Used for vertical down, as well as flat, horizontal and overhead. The last two digits (third and fourth) indicates the usability of the electrode, refer to table 3.2c. Table 3.2c Classification Current Penetration Covering Exx10 DCEP Deep Cellulose/sodium Exx11 AC & DCEP Deep Cellulose/potassium Exx12 AC & DCEN Medium Rutile/sodium Exx13 Ac & DC Light Rutile/potassium Exx14 AC & DC Light Rutile/iron powder Exx15 DCEP Medium Low hydrogen/sodium Exx16 AC or DCEP Medium Low hydrogen/potassium Exx18 AC or DCEP Medium Low hydrogen/iron powder Exx20 AC or DC Medium Iron oxide/sodium Exx24 AC or DC Light Rutile/iron powder Exx27 AC or DC Medium Iron oxide/iron powder Exx28 AC or DCEP Medium Low hydrogen/iron powder Engineering, Materials and Components Revision Date: 9/17/19 - 89 - CANADIAN INSTITUTE FOR NDE Section 3 As an example E7018 has a “as deposited weld strength” of 70,000 psi (70 ksi), can be used in all positions; it is a low hydrogen electrode with iron powder. A very important point to observe is that this electrode along with any others ending in 5, 6 or 8 have the term low hydrogen. These electrodes are the ones that must be oven stored until shortly before use. Low hydrogen electrodes were originally developed to reduce hydrogen related cracking. The electrodes must have a moisture level of less than 0.6% when tested at 980°C per AWS 5.1. Hydrogen can reduce ductility in the weld but does not affect the yield strength or ultimate tensile strength. Iron oxide is usually added to the electrode to improve its efficiency by increasing the deposition rate because of the easy melting of the iron powder. Also note, Exx10 and Exx11 both have cellulose in the covering. Common electrodes of these designations are E6010 and E6011, it is the cellulose that provides the energy as it burns to give the deep penetration typically for root (first) passes. These electrodes if oven stored would loose that energy and not provide the deep penetration. They are usually stored under room ambient conditions. AWS 5.4 (Specification for Stainless Steel Electrodes for Shielded Metal Arc Welding) These electrodes are used to weld stainless steel. An example of an electrode is E316 and is followed by a suffix. The suffix may include a letter for example: E316-xx Has a carbon content of.08% E316H-xx Has a carbon content of.04 to.08% E316L-xx Has a carbon content of.04% Other letters include Cb or Mo where specific elements have percentages different than the base alloy. As an example E308-xx has a molybdenum content of.75% and E308Mo-xx has a molybdenum content of 2.0 to 3.0%. The last two digits indicate the welding current and position that can be used. Table 3.2d AWS Classification Welding Current Welding Position Exxx(x) – 15 DCEP All Exxx(x) – 25 DCEP Horizontal and flat Exxx(x) – 16 DCEP or AC All Exxx(x) – 17 DCEP or AC All Exxx(x) – 26 DCEP or AC Horizontal and flat Engineering, Materials and Components Revision Date: 9/17/19 - 90 - CANADIAN INSTITUTE FOR NDE Section 3 AWS 5.5 (Specification for Low Alloy Steel Electrodes for Shielded Metal Arc Welding) These electrodes are used to weld higher tensile strength materials and, with one exception - Exx10, are all low hydrogen electrodes. They include: E70xx, E 80xx, E90xx and E100xx, E110xx, E120xx. They also include a suffix which specifies the chemical composition of the as deposited weld metal. A typical suffix is a letter and a single digit. Low carbon deposits include an “L”, example B2L. For other electrodes or further information consult the appropriate AWS specification or the equivalent standard in ASME. For example AWS 5.1 is identical to ASME SFA-5.1; care must be taken with respect to the revision. Gas Metal Arc Welding (GMAW) This process employs an arc between the consumable electrode (continuous wire) and the work piece; the weld area is shielded by a gas delivered from a cylinder to the welding torch. The process is often referred to as MIG (metal inert gas) welding. The nomenclature gas metal arc welding is preferred particularly since the introduction of CO 2 which is not inert. The process can be automatic or semi-automatic and can be used to weld: steels, stainless steels, aluminum, copper, nickel alloys and can be used in all positions. Advantages: Electrode length is not a restriction as in SMAW Welding can be done in all positions, a feature not true of submerged arc welding (SAW). Welding speeds and deposition rates are higher than SMAW Many stops and starts can be eliminated as compared to SMAW. Penetration can be deeper than SMAW allowing smaller fillet weld sizes to be used. Less operator skill is required than other conventional processes. Minimal post weld cleaning is required because of the absence of slag. Disadvantages: Welding equipment is more complex and usually more costly and less portable than SMAW. The gun may restrict welding in hard to reach places because of its size. The process must be protected from air drafts which can disperse the shielding gas. Relatively high level of radiated heat and arc intensity. Engineering, Materials and Components Revision Date: 9/17/19 - 91 - CANADIAN INSTITUTE FOR NDE Section 3 There are three basic types of transfer of the filler material depending on the current and the shielding gas. Short Circuit Transfer produces a small fast freezing weld pool which is used, to join thin materials, for out of position welding, and for bridging large root openings. The transfer only occurs when the electrode wire is in contact with the base metal, this can occur from 20 to 200 times per second. Globular transfer occurs with a positive electrode when the current density is relatively low. This results in large droplets, larger than the diameter of the wire electrode. Because of the large droplet size and the effect of gravity the process is limited to the flat position. Often characterized by large amounts of spatter when the arc length is too short. The arc length must be long enough to ensure the globule leaves the wire before it touches the weld pool. When higher voltages are used the results may be lack of fusion and lack of penetration. Spray transfer provides a very stable spatter free weld. The shielding gas is argon or an argon rich mixture, the current is higher than that of globular transfer above a critical value (transition current). The droplets are small and are released with force sufficient to overcome gravity. A variation of spray transfer is pulsed spray transfer, the power supply pulses between a peak and background current, allowing the weld pool to cool slightly during the application of the background current which allows the process to be used for out of position welding. Flux Cored Arc Welding (FCAW) This process involves an arc between a tubular flux cored wire and the base metal. The flux in the core can make the process self shielding, alternatively a shielding gas maybe used. The process employs a constant voltage power supply adjusting the voltage to compensate for changes in arc length as is used in GMAW. Engineering, Materials and Components Revision Date: 9/17/19 - 92 - CANADIAN INSTITUTE FOR NDE Section 3 Advantages: High deposition rates particularly for out of position welding. Less operator skill required than GMAW. Simpler and more adaptable than submerged arc welding (SAW). Deeper penetration than SMAW. More tolerant to rust and mill scale than GMAW. Disadvantages: Slag must be removed. More smoke and fumes than other processes. Equipment is more complex and less portable than SMAW. Flux cored arc welding can be used to weld mild steels, weathering steels, high strength low alloy, chrome molybdenum steels, nickel base steels, quench and tempered steels, medium carbon steels and some stainless steels. The AWS classification system employs a 5 character designation: ExxT-x E for electrode xx minimum tensile strength of the as deposited metal in ksi T (tubular) indicates flux cored wire x Usability and performance Usability Factors: Table 3.2e Type Shielding Single or Transfer Polarity Multipass T-1 Gas Multipass Spray DCEP T-2 Gas Single Spray DCEP T-3 Self Single Spray DCEP T-4 Self Multipass Globular DCEP T-5 Gas Multipass Globular DCEP T-7 Self Multipass Globular DCEN For a complete listing refer to AWS A5.20 for carbon steel electrodes, AWS A5.29 for low alloy electrodes, and AWS A5.22 for stainless steel electrodes Engineering, Materials and Components Revision Date: 9/17/19 - 93 - CANADIAN INSTITUTE FOR NDE Section 3 Gas Tungsten Arc Welding (GTAW) This process sometimes referred to as “HeliArc”, “tungsten inert gas” or “TIG” was developed in the 1930’s originally to weld magnesium. Heat is generated between a non-consumable tungsten alloy electrode and the base metal being welded. Filler material if required is fed to the molten puddle independent of the arc. The puddle is protected by a gas; normally argon, helium or a mixture of argon and helium. GTAW is used to weld stainless steel, aluminum, magnesium, copper, titanium and carbon and alloy steels. Material thicknesses which can be welded, range from a few thousandths of an inch to several inches. It is often employed for root passes in piping and the remainder of the weld completed with a higher deposition rate process. The filler material (as with GMAW) must match the base metal without any alloy addition as there is no coating or core. Advantages: High quality low distortion Free of spatter Can be used with or without filler wire Can be used with a variety of power supplies Welds most metals including dissimilar metals Precise control of welding heat Disadvantages: Low deposition rate Requires more skill and dexterity than some of the other methods Less economic than other methods above 9mm thick. Problematic in drafty environments The power supply is usually of a constant current. The electrode designations are specified in AWS A5.12 (Specification for Tungsten and Tungsten-Alloy Electrodes for Arc Welding and Cutting) and the ends of the electrodes are colour coded. Figure 3.2g, GTAW Torch5 Engineering, Materials and Components Revision Date: 9/17/19 - 94 - CANADIAN INSTITUTE FOR NDE Section 3 Plasma Arc Welding (PAW) The process uses a non-consumable tungsten alloy electrode, a plasma gas, a shielding gas and a copper alloy nozzle. A pilot arc is established between the electrode and the copper alloy nozzle, usually a high-frequency open circuit voltage, the arc is then transferred from the electrode to the work piece through an orifice in the nozzle. The plasma gas is used to generate the arc and the shielding gas protects the weld from the atmosphere. Typical gas selections: Table 3.2f Material Being Welded Plasma Gas Shielding Gas Mild steel Argon Argon Argon/2-5%Hydrogen Low-alloy steel Argon Argon Austenitic stainless steel Argon Argon/2-5%Hydrogen Helium Nickel and nickel alloys Argon Argon Argon/2-5%Hydrogen Titanium Argon Argon 75% Helium/ 25% Argon Aluminum and alloys Argon Argon Helium Copper and alloys Argon Argon 75% Helium/25% Argon There are three modes of operation: Micro plasma (melt in mode) forms a pool similar to GTAW Medium current plasma Keyhole plasma, the arc fully penetrates the work piece Advantages: In the keyhole plasma greater thickness of metal can be penetrated in a single pass In some materials up to 12mm square butt joint can be penetrated High integrity weld Fewer weld passes required than GTAW The electrode is protected by the nozzle More tolerant to arc length variations than GTAW A longer arc makes it easier to view the welding operation Engineering, Materials and Components Revision Date: 9/17/19 - 95 - CANADIAN INSTITUTE FOR NDE Section 3 Disadvantages Higher capital cost than GTAW Not as tolerant to misalignment of the joint as with GTAW More torch maintenance than GTAW PAW can be used to weld the same materials as GTAW also zirconium and tantalum. Single pass welding can handle material thicknesses from.025mm (micro plasma) to 12.5mm of aluminum. Micro plasma is used to weld high numbers of welds such as lamp filaments. Keyhole plasma is used mostly for welding stainless steel tanks and piping. It is often used for root passes where other methods are used to complete the weld and for making welded tube. Submerged Arc Welding (SAW) SAW is a process in which an arc is established (concealed by a blanket of granular flux) between a bare solid metal or cored wire, and the work piece. See figure 3.2h. A continuous electrode is fed into the joint and a layer of granular flux is deposited in front of the arc. The current can be AC, DCEN, or DCEP. After welding un- fused flux maybe screened and reused. The solidified slag can be re-crushed and blended with Figure 3.2h, virgin flux and re-used. Submerged Arc Welding Advantages: The arc is under the blanket of flux eliminating arc flash, spatter and fumes Good penetration High deposition. Cost per length of weld relatively low Good mechanical properties of the deposited weld Shielding provides protection from drafts and thermal insulation Minimal welder operator training is required Engineering, Materials and Components Revision Date: 9/17/19 - 96 - CANADIAN INSTITUTE FOR NDE Section 3 Disadvantages: Initial cost of equipment is high Joint must be flat or horizontal Slag must be removed between subsequent passes Submerged arc can be used to weld plain carbon and alloy steels, it can also be used to weld stainless steels and nonferrous metals. Electrode and Flux AWS classifications for Carbon Steels: Fxxx-Exxx F represents flux xx represents the as deposited tensile strength in 10ksi’s x represents the heat treat condition that the tensile tests were conducted (A = as welded, P = post weld heat treated) E indicates a solid electrode, EC indicates a composite electrode xxx represents the classification of electrode e.g. EL8 is a low manganese electrode EM12 is a medium manganese electrode EH11K is a high manganese electrode Electrodes for alloy steels have additional characters (refer to the appropriate AWS specification). Stud Arc Welding (SW) This process is used to join a metallic stud to a work piece, the equipment controls the arc length and dwell time. An arc is created between the stud (or fastener) and the work piece and after both pieces reach the correct temperature they are brought together under pressure. There are two basic types of arc stud welding based on the power supply used. One type uses a DC power source similar to SMAW, the second type uses capacitors and is known as capacitor discharge welding (CDSW). In the capacitor discharge stud welding the tip of the stud is melted almost instantly when energy discharged from the capacitors is released through the stud. There are three modes of operation of the CDSW process: 1. Initial-gap 2. Initial-contact 3. Drawn-arc Engineering, Materials and Components Revision Date: 9/17/19 - 97 - CANADIAN INSTITUTE FOR NDE Section 3 Plasma-MIG Welding This is a combination of plasma arc welding and gas metal arc welding employing a plasma and shielding gas and a continuous consumable solid electrode. The process can be used for welding high melting point metals such as tungsten and molybdenum but is also used to weld aluminum, stainless steels and for hard facing and cladding operations. Figure 3.2i, Plasma MIG Torch4 Resistance Spot Welding (RSW) This method is most widely used for the assembly of sheet metal products, auto- body assemblies, building products and appliances. Heat is created by the resistance to the flow of electric current through the work pieces held together under pressure. Variations of the process include resistance seam welding (RSEW) where a high speed spot welding process is used to create continuous over lapping spots between rotating electrodes to produce an air tight or liquid tight joint. Another variation employs projections on one of the components to be joined which serve to concentrate the heat in the specific area of contact or projection. The process is referred to as projection welding (PW). Both steel and aluminum can be resistance spot welded. Engineering, Materials and Components Revision Date: 9/17/19 - 98 - CANADIAN INSTITUTE FOR NDE Section 3 Resistance Seam Welding (RSEW) There are three basic types of joints: Lap seam weld Mash seam weld Butt seam weld Advantages: Joints can be gas or liquid tight High speed welds are possible Coated steels are often more weldable than with other methods Not as fit-up sensitive as other methods, e.g. laser welding Hardening is not as much of an issue as with other methods, e.g. laser welding Figure 3.2j, Resistance Seam Welding Disadvantages Geometry restrictions related to the plane of the weld There cannot be obstructions along the weld to interfere with the rotating electrodes Length of the seams may produce issues related to the throat length of the equipment and changes in electrical impedance as the weld progresses Crossing seams present quality issues at the intersection of the seams Flash Welding (FW) Flash welding is a variation of resistance welding in which the action of joining the materials employs a flash and pressure. The flash occurs as the two components are brought together with light contact or a minute air gap and then pressure is applied. The process can achieve strengths equal to the parent material. Steel, aluminum, brass, Figure 3.2k, copper and dissimilar metals can be joined. Schematic of a Flash Welding Operation3 Engineering, Materials and Components Revision Date: 9/17/19 - 99 -