ELET 307 Week 1 Review PDF
Document Details
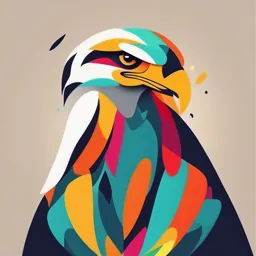
Uploaded by DevoutTanzanite4841
Tags
Related
Summary
This document covers lab/class rules, safety, the death of an electrician, and various definitions and concepts related to instrumentation and process control. It's for reviewing the week one materials of an undergraduate electrical engineering course, ELET 307.
Full Transcript
• Lab/class rules • When working at the lab stations we will adhere to industrial rules. • 1. You will wear safety classes! • 2. You will wear closed toe shoes/boots • 3. There will be no horseplay. You will act responsibly. SAFETY • The most recent statistics from the Association of Workers' Comp...
• Lab/class rules • When working at the lab stations we will adhere to industrial rules. • 1. You will wear safety classes! • 2. You will wear closed toe shoes/boots • 3. There will be no horseplay. You will act responsibly. SAFETY • The most recent statistics from the Association of Workers' Compensation Boards of Canada (AWCBC) tell us that in 2019, 925 workplace fatalities were recorded in Canada, a decrease of 112 from the previous year (1027). Among these deaths were 15 workers aged 20-24 & 31 workers aged 25-29. • Add to these fatalities the 271,806 accepted claims (an increase from 264,438 the previous year) for lost time due to a work-related injury or disease, including 24,778 from workers aged 20-24, and the fact that these statistics only include what is reported and accepted by the compensation boards, there is no doubt that the total number of workers impacted is even greater. • And it’s not just these numbers on which we need to reflect. With each worker tragedy there are loved ones, family members, friends and co-workers who are directly affected, left behind, and deeply impacted – their lives also forever changed. (Numbers from www. awcbc.org) Electrician, 27 killed at Novelis plant. • Kingston Fire and Rescue was called to the plant for a technical rescue at about 2:15 p.m. When crews arrived they performed CPR on Baerwald before he was taken to hospital. • Brady said the Ministry of Labour was at the plant yesterday investigating the incident but have since left. He said they’re now waiting for the ministry’s report while also investigating as to what happened in the incident. • “It was reported a worker was working on a crane when they were exposed to live, electrical parts,” the ministry of Labour, Training and Skills Development said in a statement. “Two (Labour, Training and Skills Development) inspectors and an engineer attended the scene.” • The ministry said two orders were issued and the investigation is ongoing. • Brady said Baerwald had worked for the company for a little over three years. Types of process measurement • Point Measurement • Detects the process variable at a predetermined point or value • The input is a simple ON or OFF, 0 or 1, true or false • Can be thought of as a digital system • Continuous Measurement • Detects the process variable within a range of all possible values at all times • Can be thought of as an analog system • Direct or Invasive Measurement • The measurement device will be in direct contact with the process • May change process • Source of contamination • Indirect or Non-Invasive Measurement • No contact, good for extreme processes • Not as accurate as results are inferred or calculated Instrumentation Terminology • Process – the system or “thing“ we want to measure and/or control. • Manipulated Variable- The part of the process that is being adjusted by the final control element. • Final Control Element: The last device the directly controls the manipulated variable. Usually, a control valve but could be a pump too. • Primary Element: The sensor. The device that senses the process. • Process Variable – the current measured condition of a process. E.g., the current temperature of your house as indicated by a thermometer. • Set point – the desired setting of the process variable. Your thermostat is set for 20C. • Error – the difference between the setpoint and the process variable E.g., your furnace is set to keep your house at 20C, but the process variable or current temperature is 17C, so the error is 3C. • Range – It can be defined as the values of the lowest and highest readings it is designed to operate. A thermometer has a scale from −40°C to 100°C. So the range varies from −40°C to 100°C • Zero – the lowest value that corresponds to the bottom or lowest limit of the process. Also called LRV (lower range value). • Span – Is defined as the difference between the minimum to maximum range value. In the case of the thermometer, its scale goes from −40°C to 100°C. So its span is 140°C. Instrumentation Devices The purpose of instrumentation or process control is to, as the name implies, control a process through the act of measuring and changing that process. Devices are parts of the process control that perform this function. • Sensors or elements • Devices that measure the process. RTD’s, Paddle wheels, Orifice plates. • Transmitters • Devices that converts non-standard signals to standard instrumentation signals. • Usually there is a sensor/element connected to a transmitter. • Controllers • Devices that controls the signal to an Actuator to cause the process to be maintained at a certain set-point. • Transducers • Devices that converts a standard instrumentation signals to a different standard instrumentation signal. 4-20mA to 3-15 psi. • Actuators (Final Control Element) • Devices that create changes in a process. Pumps, Control Valves. P&ID and ISA Symbols • P&ID is the acronym for “Piping and Instrumentation Diagram. • The (ISA) Instrumentation, systems and automation society is one of the leading trade and standards organization. • The ISA S5.1, ISO 10628, and BS 5070 cover the standardization of P&ID symbols. The list is updated periodically. • The P&ID is used by electricians (you), engineers, and operators to better understand the process and how the instrumentation is interconnected • The list of diagrams is extensive and by no means is it feasible/reasonable to show you all of them. Those shown in this lecture are only the most common! Problem with high level probe Tank 315 Building the P&ID The P&ID will use symbols and circles to represent each instrument, where they are located and how they are interconnected in the process. By “how” I mean what type of signal is each using. Standard, non-standard, electrical, pneumatic. • ISA Symbols ISA Symbols Individual Instruments • To specify an individual instrument in the P&ID we use a circle that is also called a balloon. How they are drawn will help you locate the instrument. Tag numbers • The design of the balloons help us understand where the device is located. But there is still more that needs to be added. We need to know what the process is, we need to know what the device is, and we need to know if the device has any additional functions like alarms. For this we add TAG numbers . • We can have a lot of different instruments used in a process, so we have to be able to identify them. For this we use letters and numbers. The letters are called functional identifiers and the order they are in is very important. The first letter is always the process variable, for example a level process would be identified with a “L”. The second tells us what the device is, for example a “T” would be a transmitter. The next page shows a more complete list. You should note that you cannot substitute any of these letters. ISA S5.1 Identification Letters • Note that the letter “Y” now represents a transducer!! Instrumentation signal lines • So now we have taken the simple temperature process and replaced the pictures with balloons and with the addition of tags we also know what the devices are and any additional functions. But there is one bit of information left that we can get. How do they communicate. That is accomplished by creating different lines that run between the balloons. • In order to connect the various devices in a control system together we need signal lines. The signals that are being transmitted could be pneumatic, hydraulic, electrical or some other medium. The lines we use must be designated as to the type of signal they are carrying. • Solid bold line: Indicates the actual piping of the process. • Solid thin line: Indicates a connection from the process to an instrument. • Dashed line: Indicates a standard electrical signal. Usually 4-20 mA but could be voltage too. • Solid w/ two diagonal marks: Indicates a standard pneumatic signal. 3-15 psi. • These are the most common. But you can see that there are others too. ISA Symbols Valves P&ID P&ID legend •S Sample of industrial P&ID Resistive Temperature Detector (RTD) • The most common RTDs used in industry are made from platinum. They are very stable and the most linear. Other materials such as Nickel, Balco and Copper can be used but have limitations due to nonlinearity and oxidation. • The platinum RTD can come in either industrial grade or laboratory grade. Depending on the desired accuracy. Laboratory is about ±0.01 % at 0° C and industrial can be ±0.15 to ± 0.3%. Industrial RTD’s typically have a resistance of 100Ω at 0°C. • There are 3 common designs of RTDs • Thin film sensing element, wire wound element and coil element and can be a 2, 3 or 4 wire configuration. Resistive Temperature Detector (RTD) • An RTD is a temperature device/sensor that will exhibit a positive change in resistance for a positive change in temperature. Therefore, an RTD also has a positive temperature coefficient. • Positive coefficient = a rise in temp, an increase in resistance. This is an RTD. • Negative coefficient = a rise in temp, a decrease in resistance. This is a Thermistor. Advantages & Limitations • Advantages of Platinum RTDs: • Stable for a long time. • Accurate readings for a narrow temperature range. • Disadvantages: • Limited temperature ranges • Initial higher cost • Less rugged in certain processes, Vibration. The 2-wire RTD • A simple 2-wire RTD is only used for short distances and where high accuracy isn’t necessary. Because you are adding the resistance in the leads to the resistance of the sensor. So a resistance reading of 123.4 would by found on the RTD chart to represent approx. 60 °C The 3-wire RTD • The 3-wire RTD is the most common. Because you can subtract the lead resistance. An assumption is made that the resistance of the two leads across the resistance is the same. Comparing this to the two wire RTD from before we see that subtracting the lead resistance of 3.4Ω leaves use with 120 Ω. Looking at the chart we see its closer to 52°C so an 8 °C error using a 2-wire. Rt = R-RL Rt= 123.4 – 3.4 = 120 ohms. The 4-wire RTD • The 4-wire RTD is used when very high accuracy is needed. With the 4-wire configuration, the instrument will pass a constant current (I) through the outer leads. The addition of the two additional leads makes it possible to eliminate errors due to change in lead wire resistance. This is because of a very high input impedance of the circuitry. Since there is no current flow there is no associated voltage drop in the lead wires from the RTD to the to the sensor input AB. This results in a measurement of the RTD only and the lead wires will have no effect on the reading. The voltage drop is measured across the inner leads AB. So from E = IR we learn the resistance of the element alone, with no effect from the lead wire resistance. Resistive Temperature Devices (Thermistors) • Thermistors, derived from the term THERMaIly sensitive reslSTORS, are constructed from mixtures of metal oxides. They are available in 2 types, NTC (negative temperature coefficient) and PTC (positive temperature coefficient). The NTC thermistor is more commonly used. This means an increase in temp results in a decrease in resistance. A thermistor exhibits a large change in resistance to a small change in temperature and are usually very small. Communicating process information • A standard instrumentation signal is used to represent a standard unit of measurement. For example, we can use a standard signal to represent temperature °C or level (feet). • Standard Instrumentation Signals • For a temperature transmitter to send a signal to another device (temperature controller), an agreed upon standard method of communications must be used. • Common standard instrumentation signals: • Voltage – 1 to 5V. Most common voltage. Instruments that receive a 4-20mA signal through a 250Ω resistor will produce 1- 5V. Drives can use 0-10 vdc. Other ranges, 1-10 Vdc • Current – 4 -20 mA. Most common signal to eliminate line loss and to provide power to the transmitter. • Pressure – 3 to 15 psi Rarely used. Leaks and condensation issues . Standard Signals Connections • 4-20 mA. The most popular signal used in industry. Simple to connect, configure. Better for long distances. Not as susceptible to noise caused by electrical interference compared to voltage loops. Can only transmit one signal per loop. Wrong sized wire used and improper shielding methods (ground loop). Standard Signals • 1-5 volts. Improper size or length of wire can cause a voltage drop causing a measurement error. Improper shielding to prevent EMI. Best for short distances. The controller below requires a 1-5 v signal. The transmitter sends a 4-20 mA signal. The controller measures the voltage drop across the 250 Ω resister as the current flows through it. We noticed standard signals lower range values (LRV) do not start at 0. Most standard signals have an elevated zero that is 1/4 times the value of the span. There are three reasons for this. 1. The elevated zero can provide enough power to operate the devices such as transmitters which require about 3 mA to work 2. The elevated zero can also indicate when we have a broken loop instead of a zero signal (0 mA instead of 4 mA etc.) 3. Mechanical devices respond better with a signal that starts above zero. Looking at a transmitter. If the input has a range of (0C LRV - 100C URV) then the output must be 4ma LRV and 20mA URV. Zero C relates to 4 mA ( both LRV’s) and 100 C represents 20 mA ( both URV’s). The both input and output signals will be directly proportional to each other. The range of the (4 –20mA) will be proportional to the range of the process (0C - 100C) and must be calibrated to meet this. A transmitter must be calibrated to provide a linear signal converting a nonstandard signal to a standard signal. Process Signal Calculations • No doubt you are aware that math is an important part of the skilled electricians' tools. Basic electrical equations such as Ohms law E=I x R and Power P=E x I. • Instrumentation has basic equations too: • Pv−LRV %= x100 Pspan and PV= % 100 x Pspan + LRV From these basic formulas we can derive many other useful equations Process signal conversion • There must be a specific order of operation when we solve equations. • 5 + 7 x 4 - 2 = 46 or 19 or 31 • The rules for the order of operations are: • Solve brackets first. If equations contains more then 1 set, start from inner most. • Solve exponents next • Perform all multiplication and division from left to right • Perform all addition and subtraction from left to right You can remember the order as BEDMAS Process signal conversion • Using BEDMAS lets solve this equation. • 5 + 7 x 4 -2 = • There are no brackets or exponents. • There is no division move to multiplication 5 + 7 x 4 -2 5+28 - 2 • Last step is add and then subtract from left to right. • 5+28 - 2 • 33-2 = 31 Process signal conversion • Try another one with brackets (BEDMAS ) • 80-5 x (4÷2+5) • 80-5x(2+5) • 80-5x7 • 80-35 • 45 Process signal conversion • Next with exponent and with brackets (BEDMAS ) • (3x10+ 52) +4 • (3x10 +25)+ 4 • (30+25) + 4 • 55+4 = 59 Process signal conversion • Remember, an equation is simply a statement that is equal on both sides. What we do on one side, we must do the same to the other side. • 6P+6= 24 • 6P+6-6=24-6 • 6P=18 6𝑃 18 • = 6 6 18 • P= 6 P=3 Process signal conversion When we had to find the percentage of a single variable, we divided by the span to change to a decimal format and then multiplied by 100. To convert back we divide by 100. You got 20 out of 30 on a test. What is the percent? 20 30 = 0.667 x 100 = 66.67% Going from the other direction: You got 66.67% on a test with 30 questions. How many did you get right? 66.67 x 30 = 20 100 But this was always assuming we started from zero. What if we don’t? Calculating Process and Signal Values First, we determine the % the process variable is at using the formula. %= 𝑃𝑟𝑜𝑐𝑒𝑠𝑠 𝑉𝑎𝑟𝑖𝑎𝑏𝑙𝑒 −𝐿𝑅𝑉 𝑃𝑠𝑝𝑎𝑛 x 100 A compressor has a range of 120 psi to 300 psi. Span is 180 psi. A T T output is 4– 20mA and is calibrated so that 4mA (LRV) will represent 120 psi and 20mA (URV) will represent 300 psi. • The pressure signal from the PE to the transmitter is 173 psi (PV). Find the mA output signal from the transmitter. Calculating Process and Signal Values • Range of 120 psi to 300 psi. Span is 180 psi, LRV is 120 psi. Process variable (PV) is 173 psi. The proportional signal will be: • 𝑷𝑽 −𝑳𝑹𝑽 %= 𝑷𝒔𝒑𝒂𝒏 • %= x100 173 −120 =.2944 180 x (100) = 29.44 % • Now we know that 173 psi represents 29.44% of the range. Next, we can convert that to a signal. This is because the signal range is proportional to the temperature range. Can you recognize that this is the job of a transmitter? Converting a nonstandard signal (psi) to a standard signal. In this case a 4-20 mA signal. Calculating Process and Signal Values Second, we take the percentage and use it to calculate the correct standard signal % amount. Using Pv() = x Span + LRV 𝟏𝟎𝟎 % 100 • 4-20 mA signal mA= mA = • 3-15 psi signal psi = 29.44 100 % 100 psi = x 16mA+4mA x 16mA + 4mA= 8.71mA x 12psi+3psi 29.44 100 x 12psi+3psi= 6.53psi • Since standard instrumentation signals are proportional to each other they can be converted from one standard to another. Devices designed to do this are called transducers. Calculating Process and Signal Values Something Extra • There is another way to calculate this. I think it is simpler Our Step #1 formula is converting the nonstandard signal input (°C) into a percent. Then step #2 we take the percent and calculate the output into the standard signal (mA). Why not combine the 2 formulas? 𝑃𝑉 −𝐿𝑅𝑉 %= 𝑃𝑠𝑝𝑎𝑛 Output= { x100 and Pv() = 𝑰𝒏𝒑𝒖𝒕 −𝑳𝑹𝑽𝒊𝒏𝒑𝒖𝒕 𝒔𝒑𝒂𝒏𝒊𝒏𝒑𝒖𝒕 % 100 x Span + LRV }𝑺𝒑𝒂𝒏𝒐𝒖𝒕𝒑𝒖𝒕 + 𝑳𝑹𝑽𝒐𝒖𝒕𝒑𝒖𝒕 Calculating Process and Signal Values • What should the psi output signal be if the input range is 4-20mA and the PV is 13.6 mA. • Transducer correct!!!! • Output= { • 𝐼𝑛𝑝𝑢𝑡 −𝐿𝑅𝑉𝑖𝑛𝑝𝑢𝑡 𝑠𝑝𝑎𝑛𝑖𝑛𝑝𝑢𝑡 13.6𝑚𝐴−4𝑚𝐴 { } 16𝑚𝐴 }𝑆𝑝𝑎𝑛𝑜𝑢𝑡𝑝𝑢𝑡 + 𝐿𝑅𝑉𝑜𝑢𝑡𝑝𝑢𝑡 12psi +3 psi= 10.2 psi