Electrolyte Analyzer Ion Selective Electrode Method PDF
Document Details
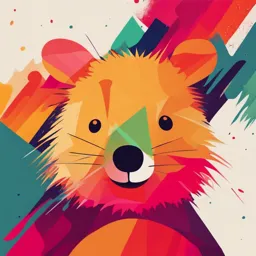
Uploaded by FortunateVerisimilitude
Tags
Summary
This document details the principle and method of the electrolyte analyzer using the ion-selective electrode (ISE) method. It explains electrolytes, ions, and ionic bonds in the context of body function. The document includes explanations of potential energy and electric fields in the context of chemical reactions.
Full Transcript
MLT 304 Electrolyte analyzer Ion Selective Electrode method SEC 1 1|Page Introduction: The electrolyte analyzer is a device for measuring the electrolytes in the human body. They are primarily used in the quantitative me...
MLT 304 Electrolyte analyzer Ion Selective Electrode method SEC 1 1|Page Introduction: The electrolyte analyzer is a device for measuring the electrolytes in the human body. They are primarily used in the quantitative measurement of sodium, potassium, and chloride in whole blood, serum, or plasma. The most common methods of electrolyte analysis are the flame emission photometry and ion-selective electrode (ISE). The ISE method of electrolyte measurement is based on the principle of potentiometry in which the voltage that develops between the inner and outer surfaces of an ISE (membrane) is measured. Electrolytes are minerals in your body that dissociate in water into electric charged particles called ions. Electrolytes are essential for basic life functioning, such as maintaining electrical neutrality in cells and generating and conducting action potentials in the nerves and muscles. Significant electrolytes include sodium, potassium, chloride, magnesium, calcium, phosphate, and bicarbonates. Electrolytes come from our food and fluids. An ion is an atom or group of atoms that has an electric charge. Ions form when atoms lose or gain electrons to obtain a full outer shell. Since an ion has an unequal number of electrons and protons, its net charge is never zero. Based on the type of charge and the ratio of protons and electrons, ions are of two types: 1. Positively charged atoms (More protons > Fewer electrons): called CATION (formed by loss of electrons in an atom) 2. Negatively charged atoms (Fewer protons < More electrons) called ANION (formed by the gain of electrons in an atom) 2|Page In other word the elements and compounds that are electrically neutral have the same number of electrons as protons, so the negative charges of the electrons are balanced by the positive charges of the protons. However, this is not always the case. Electrons can move from one atom to another; when they do, species with overall electric charges are formed. Such species are called ions. Species with overall positive charges are termed cations, while species with overall negative charges are called anions. Remember that ions are formed only when electrons move from one atom to another; a proton never moves from one atom to another. Compounds formed from positive and negative ions are called ionic compounds. potential and Kinetic energy of atoms result from the motion of electrons. When electrons are excited, they move to a higher energy orbital farther away from the atom. The further the orbital is from the nucleus, the higher the potential energy of an electron at that energy level. When the electron returns to a low energy state, it releases the potential energy in the form of kinetic energy. Potential energy is defined as the energy that is associated with the work related to an object's potential. An ionic bond is formed when an atom loses or gains one or more electrons to form a positively or negatively charged ion, respectively. When two ions with opposite charges come close to each other, the positively charged ion is attracted to the negatively charged ion. This attraction is due to the electrostatic force of attraction between the opposite charges, which is also known as Coulomb's force. The decrease in potential energy is a result of the electrostatic force of attraction between the ions. As the ions approach each other, the potential energy of the system decreases, because the electrostatic force of attraction between the ions is doing work on the system. This work is done by the electrostatic force of attraction, which is decreasing the potential energy of the system. In other words, the electrostatic force of attraction between the ions is a This attraction is due to the electrostatic force of attraction between the opposite charges, which is also known as Coulomb's force. The decrease in potential energy is a result of the electrostatic force of attraction between the ions. which means that it can do work on the system without changing its own energy. Therefore, the decrease in potential energy is equal to the work done by the electrostatic force of attraction. In summary, the decrease in potential energy in an ionic bond is a result of the electrostatic force of attraction between the positively and negatively charged ions, which is doing work on the system and decreasing its potential energy. 3|Page Electric potential energy: Electric forces are responsible for almost every single chemical reaction that occurs in your body. Understanding how these forces cause electrons to move between atoms and the alterations in the structure or composition that result from this movement are essential to almost all biochemistry. Electric force is the force that pushes two similar charges apart or pulls two dissimilar charges together. What is the electric field? We know that if you have a single positively charged particle, a positively charged particle will be pushed away from it by the electric force. Electric field defined as the area in which the stress or electric force acts around an electric charge. Electric potential energy is the energy that is needed to move a charge against an electric field. You need more energy to move a charge further in the electric field, but also more energy to move it through a stronger electric field. The electric potential, or voltage, is the difference in potential energy per unit charge between two locations in an electric field. Example: Consider a negatively charged plate as an example. We are aware that it will attract a positively charged particle. As a result, we may infer that our hypothetical positively charged particle would have a little amount of electrical potential energy if we set it close to the plate and a larger amount of electrical potential energy if we place it farther away. Therefore, it is possible to state that the electrical potential is low close to the negative plate and high farther away. 4|Page Suppose our plate had a positive charge. The plate would be pushed away from a positively charged particle. The last instance is exactly the opposite of this. Electrical potential is either high or low depending on how close you are to the plate. 5|Page Redox reactions: are oxidation and reduction reactions that happen simultaneously in a chemical reaction. oxidation occurs when an atom, molecule, or ion loses one or more electrons in a chemical reaction. Reduction occurs when an atom, molecule, or ion gains one or more electrons in a chemical reaction. The ion or molecule that accepts electrons is called the oxidizing agent - by accepting electrons it oxidizes other species. The ion or molecule that donates electrons is called the reducing agent - by giving electrons it reduces the other species. Electrochemical cell and potentiometry An electrochemical cell is a device capable of either generating electrical energy from chemical reactions or using electrical energy to cause chemical reactions. 6|Page Half-Cells and Cell Potential Electrochemical Cells are made up of two half-cells, each consisting of an electrode which is dipped in an electrolyte. The same electrolyte can be used for both half cells. These half cells are connected by a salt bridge which provides the platform for ionic contact between them without allowing them to mix with each other. An example of a salt bridge is a filter paper which is dipped in a potassium nitrate or sodium chloride solution. One of the half cells of the electrochemical cell loses electrons due to oxidation and the other gains electrons in a reduction process. It can be noted that an equilibrium reaction occurs in both the half cells, and once the equilibrium is reached, the net voltage becomes 0 and the cell stops producing electricity. The tendency of an electrode which is in contact with an electrolyte to lose or gain electrons is described by its electrode potential. Primary and Secondary Cells Primary cells are basically use-and-throw galvanic cells. The electrochemical reactions that take place in these cells are irreversible in nature. Hence, the reactants are consumed for the generation of electrical energy and the cell stops producing an electric current once the reactants are completely depleted. Secondary cells (also known as rechargeable batteries) are electrochemical cells in which the cell has a reversible reaction, i.e. the cell can function as a Galvanic cell as well as an Electrolytic cell. Types of Electrochemical Cell: Galvanic Cells [voltaic cells]. Electrolytic Cell. 7|Page 1- Properties of Galvanic Cells [voltaic cells]. The standard Daniell Cell is a galvanic cell (or voltaic cell) composed of two half-cells. A Galvanic cell converts chemical energy into electrical energy. Here, the redox reaction is spontaneous and is responsible for the production of electrical energy. In one half-cell a solid copper electrode is placed in 1 mol L-1 aqueous solution of copper(II) sulfate. In the other half cell, a solid zinc electrode is placed in 1 mol L-1 aqueous solution of zinc sulfate. The two electrodes are connected by an external wire. The two solutions are connected by a salt bridge. the anode is negative and cathode is the positive electrode. The reaction at the anode is oxidation and that at the cathode is reduction. The electrons are supplied by the species getting oxidized. They move from anode to the cathode in the external circuit. 8|Page node Half-cell (-) Cathode Half-cell (+) electrode: electrode: zinc metal, Zn(s) copper metal, Cu(s) electrolyte: electrolyte: 1 mol L-1 ZnSO4(aq) 1 mol L-1 CuSO4(aq) oxidation reaction: reduction reaction: Zn(s) → Zn2+(aq) + 2e- Cu2+(aq) + 2e- → Cu(s) Redox reaction Anode (oxidation) e- cathode (reduction) Zn(s) → Zn2+(aq) + 2e- → 2e- + Cu2+(aq) → Cu(s) Anode (oxidation) e- cathode (reduction) Zn(s)|Zn2+(aq)||Cu2+(aq)|Cu(s) 2- Properties of Electrolytic Cell An electrolytic cell converts electrical energy into chemical energy. The redox reaction is not spontaneous and electrical energy has to be supplied to initiate the reaction. Both the electrodes are placed in a same container in the solution of molten electrolyte. the anode is positive and cathode is the negative electrode. The reaction at the anode is oxidation and that at the cathode is reduction. The external battery supplies the electrons. They enter through the cathode and come out through the anode. 9|Page In other words, two different kinds of electrochemical cells—galvanic and electrolytic— involve the transmission of electrons between electrodes and an electrolyte. An electric current is produced by a spontaneous chemical reaction in a galvanic cell. At two separate electrodes connected by a wire, the reaction takes place. As one electrode oxidizes (loses electrons), the other electrode reduces (gains electrons). This causes an electric current to travel through the wire and create a flow of electrons. In an electrolytic cell, a non-spontaneous chemical reaction is sparked by the application of an external electric current. Two separate electrodes that are submerged in an electrolyte are where the reaction takes place. Reduction occurs on the electrode attached to the power source's positive terminal, and oxidation occurs on the electrode connected to the negative terminal. The non-spontaneous reaction is fueled by an electron flow caused by this through the electrolyte. Consequently, the source of the electric current is the primary distinction between electrolytic and galvanic cells. In an electrolytic cell, a non- spontaneous chemical reaction is driven by an external electric current as opposed to a spontaneous chemical reaction in a galvanic cell. Potentiometry is a technique that is used in analytical chemistry, usually to find the concentration of a solute in solution. In this technique, the potential between two electrodes is measured using a high-impedance voltmeter. This method has been developed to use ion-selective-membrane electrodes. These electrodes have many advantages, such as simple design, construction, and manipulation; reasonable selectivity; fast response time; applicability to colored and turbid solutions; and possible interfacing with automated and computerized systems. 10 | P a g e ion-selective-membrane electrodes. Ion selective electrode is a widely used analytical tool for sensing ions in various real samples of clinical, industrial, environmental, and laboratory research. These are membrane electrodes, that act as a sensor, that converts the activity of a specific ion into an electrical potential. Ion selective electrode (ISE) is an electrochemical sensor that works based on the principle of a galvanic cell. It converts the activity or concentration of a specific ion present in a solution into electrical potential. Ion selective electrodes are the electrode that generates potential in the response to the presence of a solution of a specific ion. Since this electrode detects specific ions only, it can also be considered a transducer or sensor. An ideal ISE consists of a thin membrane across which only the specific ion can be transported. The transport of ions from a high concentration to a low concentration one through selective binding with some sites within the membrane creates a potential difference, this is the fundamental principle of ISE. ISE consists of a thin membrane. Only specific ion can be diffuse. By measuring the electric potential generated across a membrane by “selected” ions and comparing it with reference electrode. And net charge is determined. 11 | P a g e General component 12 | P a g e Indicator electrode: Electrode that responds to analyte (sodium electrode, potassium electrode, ionized calcium electrode, chloride electrode, etc Membrane (Glass membranes, Crystalline membranes, Ion-exchange resin membranes & Enzyme electrodes) Reference electrode: Second ½ cell at a constant potential. Cell voltage is difference between the indicator and reference electrode. Ion selective electrodes can be used: 1. To determine various ions in aqueous solutions. 2. To measure the pH of the solution. 3. To measure different types of ions present in the soil. 4. To measure NO3–, NO2– ions in meat preservatives, and salt content in meat, fish, and fruit juices. 5. To determine fluoride ions in drinking water and other drinks. 6. To determine ions such as Ca++, K+, Cl– etc in body fluids like plasma, serum, and sweat. 7. As a chemical sensor for research purposes. 13 | P a g e 1- Sodium (Na+) Sodium is the major extracellular cation and exerts a major influence on plasma osmolality. It plays a central role in maintaining the normal distribution of water and osmotic pressure. Changes in serum sodium most often reflect changes in water balance rather than sodium balance. It is adjusted by antidiuretic hormone (ADH) secretion and the thirst receptors to maintain plasma osmolality and volume. Aldosterone causes tubular reabsorption of sodium. Determinations of plasma sodium levels detect changes in water balance rather than sodium balance. Sodium levels are used to determine electrolytes, acid-base balance, water balance, water intoxication, and dehydration. Normal Adults: 136–145 mEq/L (136–145 mmol/L) Children (1–16 years): 136–145 mEq/L (136–145 mmol/L) Full-term infants: 133–142 mEq/L (133–142 mmol/L) Premature infants: 132–140 mEq/L (132–140 mmol/L) In urine (24h): 40-220 mmol/day Critical Values < 125 mEq/L (_125 mmol/L) causes weakness and dehydration. 90–105 mEq/L (90–105 mmol/L) causes severe neurologic symptoms and vascular problems. > 152 mEq/L (_152 mmol/L) results in cardiovascular and renal symptoms. > 160 mEq/L (_160 mmol/L) can cause heart failure. 14 | P a g e I) Hyponatremia occurs when serum sodium level is 150 mmol/L. a) Usually occurs when water is lost as through diarrhea, excessive sweating, or diabetes insipidus, and when sodium is retained as through acute ingestion, hyperaldosteronism, or infusion of hypertonic solutions during dialysis. Serum, plasma, and urine are all acceptable for Na+ measurements. When plasma is used, lithium heparin, ammonium heparin, and lithium oxalate are suitable anticoagulants. Hemolysis does not cause a significant change in serum or plasma values because of decreased levels of intracellular Na+. However, with marked hemolysis, levels may be decreased because of a dilutional effect. Whole blood samples may be used with some analyzers; however, consult the instrument operation manual for acceptability. The specimen of choice in urine Na+ analyses is a 24-hour collection. Sweat is also suitable for analysis. Sodium is stable in serum for at least 1 week at room or refrigerator temperature, or it can be frozen for up to 1 year. 1- Many drugs affect levels of blood sodium. a. Anabolic steroids, corticosteroids, calcium, fluorides, and iron can cause increases in sodium level. b. Heparin, laxatives, sulfates, and diuretics can cause decreases in sodium level. 2- High triglycerides or low protein causes artificially low sodium values. 15 | P a g e Through the years, Na+ has been measured in various ways, including chemical methods, flame emission spectrophotometry, atomic absorption spectrophotometry (AAS), and ISEs. Chemical methods are outdated because of large sample volume requirements and lack of precision. ISEs are the most routinely used method in clinical laboratories. 2- Potassium (K+): Potassium (K+) is the major intracellular cation in the body, with a concentration 20 times greater inside the cells than outside. Many cellular functions require that the body maintain a low ECF concentration of K+ ions. As a result, only 2% of the body's total K+ circulates in the plasma. Functions of K+ in the body include regulation of neuromuscular excitability, contraction of the heart, ICF volume, and H+ concentration. Evaluation of electrolyte balance, cardiac arrhythmia, muscular weakness, hepatic encephalopathy, and renal failure. Diagnosis and monitoring hyperkalemia and hypokalemia in various conditions (e.g., treatment of diabetic coma, renal failure, severe fluid and electrolyte loss, effect of certain drugs). Diagnosis of familial hyperkalemic periodic paralysis and hypokalemic paralysis. Normal Adults: 3.5–5.2 mEq/L (3.5–5.2 mmol/L) 16 | P a g e Children (1–18 years): 3.4–4.7 mEq/L (3.4–4.7 mmol/L) Infants: (7 days–1 year): 4.1–5.3 mEq/L (4.1–5.3 mmol/L) Neonates (0–7 days): 3.7–5.9 mEq/L (3.7–5.9 mmol/L) In urine (24h): 25-125 mmol/day. Critical Values 8.0 mEq/L (_8.0 mmol/L) causes muscle irritability, including myocardial irritability. 1) Hypokalemia occurs when serum potassium level is 5.0 mmol/L. Results from increased intake, renal failure, hypoaldosteronism metabolic acidosis, increased red blood cell lysis, leukemia, chemotherapy. Serum, plasma, and urine may be acceptable for analysis. Hemolysis must be avoided because of the high K+ content of erythrocytes. Heparin is the anticoagulant of choice. Whereas serum and plasma generally give similar K+ levels, serum reference intervals tend to be slightly higher. Significantly elevated platelet counts may result in the release of K+ during clotting from rupture of these cells, causing a spurious hyperkalemia. In this case, plasma is preferred. Whole blood samples may be used with some analyzers. Urine specimens should be collected over a 24-hour period to eliminate the influence of diurnal variation. 1. Proper collection and handling of samples for K+ analysis is extremely important because there are many causes of artifactual hyperkalemia. 17 | P a g e 2. the coagulation process releases K+ from platelets, so that serum K+ may be 0.1 to 0.7 mmol/L higher than plasma K+ concentrations. If the patient's platelet count is elevated (thrombocytosis), serum K+ may be further elevated. this situation may be avoided by using a heparinized tube to prevent clotting of the specimen. 3. If a tourniquet is left on the arm too long during blood collection or if patients excessively clench their fists or otherwise exercise their forearms before venipuncture, cells may release K+ into the plasma. 4. Because storing blood on ice promotes the release of K+ from cells, whole blood samples for K+ determinations should be stored at room temperature (never iced) and analyzed promptly or centrifuged to remove the cells. 5. if hemolysis occurs after the blood is drawn, K+ may be falsely elevated—the most common cause of artifactual hyperkalemia. Slight hemolysis (≈50 mg/dL of hemoglobin) can cause an increase of approximately 3%, while gross hemolysis (>500 mg/dL of hemoglobin) can cause an increase of up to 30%. 6. Drug usage a. IV administration of potassium penicillin may cause hyperkalemia b. Glucose administered during tolerance testing or the ingestion and administration of large amounts of glucose in patients with heart disease may cause a decrease of as much as 0.4 mEq/L (0.4 mmol/L) in potassium blood levels. c. A number of drugs raise potassium levels, especially potassium-sparing diuretics and nonsteroidal anti-inflammatory drugs, especially in the presence of renal disease. As with Na+, the current method of choice is ISE. 18 | P a g e 3- Chloride (Cl-): Chloride is found in serum, plasma, CSF, tissue fluid, and urine. Physiologically, only the concentration of chloride in the extracellular fluid is important. Chloride (Cl−) is the major extracellular anion, and its precise function in the body, it is involved in maintaining osmolality, blood volume, and electric neutrality. It reflects changes in sodium; if it changes independent of sodium, this is usually due to an acid–base disorder. In most processes, Cl− shifts secondarily to a movement of Na+ or HCO3-. With sodium, potassium, and carbon dioxide to assess electrolyte, acid– base, and water balance. Chloride usually changes in the same direction as sodium except in metabolic acidosis with bicarbonate depletion and metabolic alkalosis with bicarbonate excess, when serum sodium levels may be normal. Normal Adults: 96–106 mEq/L (96–106 mmol/L) Newborns: 96–113 mEq/L (96–113 mmol/L) Urine (24h): 110-250 mmol/day. Critical Values 120 mEq/L (_70 or _120 mmol/L). 1) Hypochloremia occurs when serum chloride level is 107 mmol/L. a) Results from prolonged diarrhea, renal tubular disease, dehydration, excess loss of bicarbonate. 19 | P a g e Serum or plasma may be used, with lithium heparin being the anticoagulant of choice. Hemolysis does not cause a significant change in serum or plasma values as a result of decreased levels of intracellular Cl−. However, with marked hemolysis, levels may be decreased as a result of a dilutional effect. Whole blood samples may be used with some analyzers. The specimen of choice in urine Cl− analyses is 24-hour collection because of the large diurnal variation. Sweat is also suitable for analysis. The plasma chloride concentration in infants is usually higher than that in children and adults. Certain drugs may alter chloride levels. Increases are associated with excessive IV saline infusions. There are several methodologies available for measuring Cl−, including ISEs, amperometric-coulometric titration, mercurimetric titration, and colorimetry. The most commonly used is ISE. For ISE measurement, an ion-exchange membrane is used to selectively bind Cl− ions. 20 | P a g e