Electrical Control Components PDF
Document Details
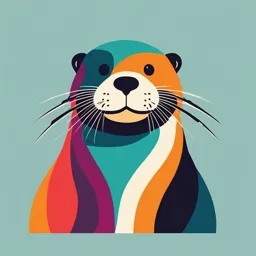
Uploaded by ScenicHummingbird6529
Null
Tags
Summary
This document provides detailed information about electrical control components, including relays, contactors, and magnetic amplifiers. It explains the principles of operation for these components and their applications. The information focuses on the technical aspects of these components.
Full Transcript
Relays Contactors Magnet Valve Capacitors Magnetic amplifier ELECTRICAL CONTROL COMPONENTS ***************************** RELAYS A relay is essentia...
Relays Contactors Magnet Valve Capacitors Magnetic amplifier ELECTRICAL CONTROL COMPONENTS ***************************** RELAYS A relay is essentially, a switch which is operated by an electromagnet instead of manually. A relay may open or close one or more circuits when energised or it may open one circuit and close another. When the requirements of one circuit depend on the function of another a relay is used, In other words, it may be essential for current to flow or cease to flow in a certain circuit when the current starts or ceases to flow in another circuit. Further-more a relay may be calibrated in such a way that it will only operate when the current passing through its own operating coil has risen or fallen to a certain value. A relay is shown in a circuit as a coil and a pair of contacts. When the operating coil is energised a normally open contact is closed, whereas a normally closed contact is opened. Circuit diagrams always show relays in de-energised position. CONTACTORS Contactors are switches which handle heavy current and operate as a result of the action of other switches or relays. They fall into two main classes electromagnetic (EM) and electro pneumatic (EP). ELECTRO-MAGNETIC CONTACTORS Magnetic contactors are operated directly by electricity. When the current flows through the operating coil, the core becomes a magnet and pulls the armature against the core. This is the force that closes the contact tips and completes the circuit. As the armature moves, it also stretches the armature spring. When the current flow to the coil is stopped the core ceases to be a magnet. The armature spring pulls the armature away from the core and back against the stop. This is the force that opens the contact tips. The knife edge on the armature may become worn and may cause faulty operation. All contactors have a flexible lead called a shunt. It is made by a woven strands of very fine copper wire. It is expected to last several hundred thousands operations of the contactor. But since copper strands are very fine, the shunt can be easily damaged on large contactors. This may happen through misdirected arcs. If an arc strike the shunt it may weld together a large number of strands. Then this part of the shunt is no longer flexible. The result is usually a broken shunt. While a damaged shunt may last to the next inspection, it is good idea to replace it as soon as possible. Loose connection also shorten shunt life. If the bolts that fasten the ends of the shunt to the contactor get loose, there is a poor connection and the shunt terminals will over heat. This makes the copper strands brittle and dis-coloured such a shunt will break in a little while. CONTACT TIPS The exposed surfaces which actually make contact and complete the circuit are called contact tips. They get mechanical wear and electrical burning. They are usually made to copper and bolted in place. If these bolts get loose the tips will overheat. The heat will carry out to surrounding parts and cause warping and bending. INTERLOCKS These are small contact fingers that work at the same time as the main contacts. They are usually connected in the low voltage or battery circuit and give a signal when the main contacts operate. Two kinds are common. One is normally open, that is the interlocks are opened when the main contacts are closed. The other is normally closed. That is, the inter lock circuits are closed when the main contacts are open and open when the main contacts are closed. Interlocks, being in low voltage circuits usually have silver contact tips to reduce electrical resistance. USE (i) When the current is not of a strength which demands a contactor of large physical size. Examples are Generator field contactor (GF), fuel pump contactor (FPC), starting contactors (CK1, CK2), etc. (ii) When no compressed air is available. An electromagnetic contactor has its contacts drawn together by an electromagnet. When the magnet is de-energised the contacts are open and when the magnet is energised the contacts are drawn together under influence of the magnetic field. The current which energises the operating coil of a contactor is provided by a relay or a switch contact. AIR OPERATED CONTACTORS In the main traction circuits, that carry heavy currents, contactors operated by air pressure are commonly used. Such contactor has a piston, piston rod, spring and cylinder. The piston packing is of some flexible material usually rubber or leather. The other end of the rod is connected to a lever which moves one of the contact tip commonly called the movable tip. The cylinder is a casting with the walls honed to a very smooth finish. All air operated contactors have some means of lubricating the cylinder. This lubricant does three things. First, it reduces wear and prolongs the life of the piston packing. Second, piston moves freely. Thirdly, it helps the packing to fit closely and form an airtight seal. If the cylinder walls get sticky the operation of the contactors becomes sluggish. The control air available at 70 psi or 5 kg./cm2 is used for operation of the contactors. If the pressure is below normal the operation of the contactors is sluggish. Air entering the cylinder, builds up pressure and pushes the piston away from the contactor base. This is the force that closes the contact tips. At the same time the piston return spring is compressed. When the air supply is cut off, the spring forces the piston back. This is the force that opens the contact tips. MAGNET VALVE A magnet valve is used to control the flow of air to the piston. It is just two position air cock remotely controlled by electricity. In one position it admits air to the cylinder. In the other, it cuts off the air supply and opens on exhaust port to let the air in the cylinder escape. The valve position is determined electrically. When the current flows through the operating coil the valve moves to one position. When the current is cut off, a return spring moves the valve to the other position. Magnet valves usually require very little maintenance. Many of them have a ground valve seat. If this does not fit properly the valve will leak. This can usually be detected by hissing sound. A leaky valve should either be repaired or replaced for it may causes faulty operation of a contactor. A magnet valve may also have a bent or sticky valve stem. When a contactor opens a circuit carrying current, an arc is drawn between contact tips. This is very hot like the arc used in electric welding. For long life of contact tips, this arc must be got rid off as quickly as possible. Near the tip of contactors a "blow out" coil is provided, made up of a few turns of heavy copper. This coil has an iron core inside. The current flowing through the contact tips also flows through out the "blow out" coil and causes the arc around the coil to become magnetic. When an arc is drawn as the contactor opens, it will be in this magnetic area. The magnetic action will force the arc to the end of the tips and out, as if it were blown by an air jet. Contactor tips are made to have a rolling or knuckling action. When the tips first touch each other, contact is made on their toes and when they are pressed together by their proper action rolling takes place and contact is established on their heels. Conversely, when the contact tips part, it is the toes which actually break the current. The purpose or this is to have the arcing taking place between the toes of contact tips when they open, leaving the heels unburnt, so that good contact is maintained. SWITCHES Switch is a device for making and breaking a flow of current. Switches may be made in varying sizes and capacities according to their purpose. A common example of the use of switch is for switching on and off an electric light. This type of switch, the current passed is usually, at the most, only one of an amp. and the switch is required to break the circuit by a relatively small gap. If a switch is to carry a heavier current its construction will be heavier and its contact carry heavy current. Usually it consists of a hinged blade made of copper and fitted with an insulated handle, which is moved in and out of copper jaws. The blade portion is couped to one terminal and the jaws to the other terminal. It may be required to open two circuits simultaneously and two switches may be "gauged" together for this purpose. An insulated bridge piece is used to separate the two blades. Battery switches on many locomotives are made on this principle. A switch can be operated when a heavy current is flowing is provided with a "Flick" blade. This is a spring loaded blade and is attached to the main blade. When the main blade is opened initially the flick blade remains in position and the current continuous to flow through it. When the main blade is almost fully opened, the spring tension pulls the flick blade from its jaws very rapidly and thereby ensures that the current is broken instantaneously. A switch without a flick blade would allow a person to open the switch at a short distance and cause surface arcing between the blade and the jaws. CAPACITORS Capacitance is the property of a circuit to oppose any change in the amount of voltage. It is present practically in all circuits. A capacitor is formed whenever two conductors are placed close to one another but separated by an insulation know as dielectric, conductors are usually thin plates of metal to give sufficient area. Insulator is usually a very thin piece of material. The unit of capacitance is the "farad". A circuit has a capacitance of a farad, when a Voltage changing at the rate of 1 volt per second, causes an average current of 1 ampere to flow, Farad being too large a unit for practical purposes, a micro-farad is commonly used, Even this unit is large so pico- farad is now internationally used. The capacitance is generally affected by (a) Area of plates (b) Dielectric constant (C) Thickness of dielectric The above three points are to be kept in view by the designers. Another important point to be remembered is the break down voltage of the dielectric. A capacitor for higher voltage would have a thicker di-electric. Capacitors can be classified as:- (a) Fixed type (b) Variable type FIXED TYPE (i) Air dielectric type (ii) Paper dielectric type (iii) Ceramic capacitor (iv) Mica dielectric type (v) Oil dielectric capacitor (vi) Electrolytic capacitor (a) Dry type (b) Wet type VARIABLE TYPE (a) Can be classified depending on type of application (b) Depending on dielectric material chosen for construction (c) Depending on nature of variation of capacitance with rotation of the shaft. For practical purposes it can be said that capacitor will allow a-c to pass through it. Whenever a capacitor is connected to a circuit with d-c voltage across its terminals, it is charged and whenever disconnected discharged. Small capacitors are often connected across relay when contacts open, there is a good bit of electricity that must be got rid off in hurry. This would naturally cause a burning flash. A capacitor connected across these contacts stores this electricity and prevents the flash. If a burnt finger on such a relay contact is found, it is likely that the capacitor is open. MAGNETIC AMPLIFIER PRINCIPLE OF MAGNETISM All materials are composed of atoms in which electrons revolve around a nucleus. There are many orbits each containing electrons revolving around the nucleus and at the same time spinning on their own axis. Due to these movements, tiny magnetic fields exist between the electron and the nucleus, as well as around the electrons, with north and south poles established. The modern theory of magnetism is that the direction of spin of electrons are different (clockwise or anti-clockwise), so that within one atom some of the electrons are spinning clockwise and some counter clockwise. An atom may contain an excess of spins in a clock or counter clockwise direction or a balanced number of spins. Although each electron has a tiny magnetic field with a north and south pole, a collection or group of these electrons spinning in the same direction would add their fields to create a greater magnetic field, unbalanced, are considered to be magnetic material. Those materials having a balanced number of spins are non-magnetic. If the tiny magnetic field of a spinning electron lines up (alignment of poles) with other field within the atom, and this one atom aligns itself with other atoms to form a group, this aligned group of atoms is called a "domain". The domains of magnetic materials are considered as tiny magnets with direction (north and south poles). These domains are arranged in a haphazard or random fashion within the materials when it is de-magnetised. An illustration of this is shown in the Fig. with the arrows representing and showing the direction of "Domain". When an external force capable of causing these tiny magnets to align or orient themselves so that they are pointing in the same direction, the magnetic material is said to be magnetised. Fig. shows all the domains oriented in one direction. Any attempt by an external force to improve on this alignment would be useless since all the domains are already aligned and pointing in one direction and the magnetic material is said to be saturated and it would have its maximum magnetic strength. Magnetic materials require varying amounts and different types of external means to cause maximum alignment of these domains. This also means that degree of magnetic strength of materials can be varied by varying the alignment or orientation of these domains which act as tiny magnets. We know that when iron fillings are sprinkled on a piece of cardboard placed on top of a magnet they arrange themselves in a definite pattern. This is due to the influence of lines of force and area in which this influence is felt is know as the magnetic field. The lines of force, within a magnetic field is often referred as "lines of flux" or simply flux and are designated by symbol O. The unit of flux is called Maxwell. The flux per unit area is know as flux density and is designated by ‘B’. Flux density (B) =Total flux () / Area (A) To cause flux to flow in a particular material, a force is necessary. It is called "Magnetomotive Force". Magnetomotive is often referred to as ampere-turns of a coil, since it is the product of the number of turns of the coil and the current flowing through the coil. The symbol used for this is mmf (or AT). If the current flowing through the coil is increased, the intensity of magnetic field also increases. For a given set of conditions, Magnetizing force (H) is proportional to strength of magnetizing current. (Magnetising force, H =AT per unit length of coil). Permeability of a substance may be defined as measure of its magnetic conductivity and is designated by the Greek letter ( ). Actually it indicates the relative case with which a material will permit magnetic flux to pass through it. Permeability () = Flux density (B) / Magnetic Force (H). or B = x H Permeability compares the relative case of a substance in conducting flux lines in air (air being standard). For example, a given coil with a known current produces 1000 lines of flux when air as its core. If with a change in core material the coil produces 6000 lines of flux, this material has permeability as 6. Ferromagnetic materials have high permeability para-magnetic materials have low permeability. Reluctance may be defined as measure of magnetic resistance. It is designated by. The MMF, flux and reluctance in a magnetic circuit is analogous to EMF, current and resistance in an electric circuit. We have seen above that B = x H i.e. the flux density depends on two things permeability () of the conducting materials and magnetising force (H). The variation of flux density with the change of magnetising force can be observed by B-H curve. From the B.H. curve it can be seen that at very low values of magnetising force (0-2) flux density increases at a very low rate. This is due to the initial inertia possessed by the domains within the material, and an energy is required to overcome this inertia. In the region 2-4 ampere turns per inch, the curve is almost linear, indicating that the domains are now responding by orienting in a particular direction at a nearly linear rate. Beyond 5 ampere turns per inch, the rate of increase of flux drops off until a point is finally reached no matter how great the magnetising force is, the amount of flux lines the magnetic material is capable of holding, has reached a maximum. The magnetic material at this point is said to have maximum magnetism or be saturated. The domains of the material have completed their alignment and are all pointing in the same direction. The magnetisation curve differs in shape and characteristics with different materials. Some would have large slopes, others small slopes. Some would saturate with small amount of magnetizing force, others would require large amount of magnetising force to saturate the material. A magnetising curve would depend upon type of material, Degree of purity, Heat treatment. Degree of radiation exposure and previous magnetic history. The magnetisation curve can be divided into three operating regions. REGION – A (Unsaturated region), increasing values of magnetising force (H) causes flux density (B) to increase very rapidly. REGION - B (Transition region), Core tending toward saturation despite increasing values of magnetizing force (H), flux density (B) decreases slowly. REGION-C (Saturation region), large values of magnetising force (H) results in little or no flux density change (B) within the core. When a magnetic material subjected to an increasing magnetising force until the saturation point is reached and then the magnetising force is decreased to zero and established in the opposite direction until the saturation point is again reached. The magnetising force is again decreased to zero and again increased until the cycle is completed, the relationship between flux density and magnetising force so obtained is represented by a loop called by hysteresis loop. Hysteresis is a Greek word meaning to lag. Note that the lines of forces lag behind the magnetising force that causes them. The characteristic of these loops are as follows: - 1. Points A - B : Positive saturation levels with increasing positive magnetising force. 2. Points E - F: Negative saturation levels with increasing negative magnetising force. 3. OO and OC1: Positive residual magnetism in the core. The magnetic material has the ability of retaining its magnetism despite the removal of the magnetising force (Note that hard steel is capable of retaining a larger amount of flux density than soft iron). 4. OD and OD1: Coercive force: This is the amount of magnetising force necessary to reduce the residual magnetism to zero, or the amount of force necessary to clear the material of all its flux. 5. OF and OF1: Negative residual magnetism produced by the negative cycle of an alternating current. 6. Areas within the loop: Represent the power losses per cycle of magnetism of the iron core. BASIC PRINCIPLES OF MAGNETIC AMPLIFIER OPERATION. There are mainly two categories of magnetic materials: (a) Linear B-H loop magnetic materials (b) Rectangular or non-linear B-H loop magnetic materials. The two types of the B-H loops are shown in Fig. In case of linear magnetic materials the magnetomotive force required to saturate the core is extremely high compared to that of a non-linear materials. Secondly, saturation in the linear materials is not sharp enough for practical purposes. Permeability is constant and quite low even before saturation compared to the rectangular loop materials. The uniqueness of square loop materials with low maximum coercive force (HC) and sharp saturation makes it very useful for magnetic amplifiers and saturating transformers. The ideally suited rectangular loop material for magnetic amplifiers should have: (a) High saturation flux density (BS) (b) Very high permeability in the unsaturated region (c) Very low permeability in the saturated region (d) Very low coercive force (H.C.) From the rectangular B-H loop one can classify: (i) When the core is unsaturated (ii) When the core is fully saturated From the faraday’s laws of electromagnetism we know: e = L di/dt and e = N d/dt From the above two equations we get L = N d/di di is proportional to `H` the magnetising force. From the above equation, when the core is unsaturated (when O increases as H increased) L is of very high value and maximum as do/di is very high and if the core is saturated (O is constant) L is zero or very small as do/di is zero or very low. Thus one can change the impedance of the magnetic core by changing the H and hence the saturating levels. If L of the core is high, small current will flow in the circuit and if L is small, very high current flows through the circuit, thus giving rise to variable impedance operation. The simple saturable reactor consists of two electric circuits connected by a magnetic circuit, as shown in the Fig. D. C current in the control loop flows through windings N1, which establishes the magnetomotive force for ampere terms of the control winding. Current flows in this winding and sets up a d.c. flux. Since an a-c source is connected in the load loop, a-c current flows through winding N2 establishing the magnetomotive force (f1 ampere turns) of load winding N2. Let us consider that d-c control current is zero. Since only a-c current is flowing through the load winding: an extremely high impedance due to high value of inductance L of the core is present in the load 1 winding. This limits the flow of a-c current to a low value and hence low voltage drop across the load. In case of a-c control current in control loop gives rise to d-c flux which super imposes on the d-c flux and collectively saturates the core. Hence the impedance of the a-c circuit decreases and more current flows in the load. Thus the output across the load depends upon the control current. The operation of the magnetic amplifier can be further understood if we review the knowledge of alternating current. We know that when unidirectional (DC) current is flowing in a circuit it has to overcome only the resistance. But when A-C flows it has not only to overcome the resistance but also Inductive Reactance, the vector sum of these two is known as impedance and is mathematically expressed as Z (impedance) =R2+XL2 where XL = Inductive reactance and R = resistance. The inductive reactance is due to the fact that alternating current is carrying from 0 to maximum back to zero, then maximum on the negative side and again zero in a sinusoidal wave form. Due to this variation of current the flux linking the circuit also varies and as stated by Faraday`s Law, an emf is induced and we know from lenz`s law that this induced emf sets up a current which opposes the very source which is creating it. If we have a core as shown in the Fig. Having an A-C winding (Load) and a D.C. winding (Control). Suppose there is no d.c. current in the control winding and A-C flows in the load winding, then do/di i.e. change of flux with change in current will be very high. With high do/di inductive reactance becomes very high and hence impedance will be very high and so the A-C flowing in the loading will be small. Now if D.C. is allowed to flow in control winding this will set up a flux in the core and if it saturates the core, the flux variation due to A-C will have no effect on the core (as it is already saturated). So do/di=0 inductive reactance will be zero and hence impedance almost zero so maximum A-C (Load current) will flow. In this way the load current (A-C) can be controlled by the control current (D-C) i.e. increase D.C., A.C. will increase, decrease D.C., A.C. will also decrease. If the control current is sensed from voltage or current to be measured, then the output across the load will be directly proportional to the voltage or current to be measured. The same principle is used in ACCR & VCR. NOTE : SOME RELAY TYPES DO NOT HAVE CONTACTS 1 AND 5 Fig. 1 contact identification. CONTACT TIPS TOUCHING Fig. 2 CONTACTOR OPEN 1. Arc Chute 5. Cotter pin 9. Piston 13. Shunts 2. Cylinder 6. Piston- Rod nut 10. Sealing Washer 14. Cylinder Head assembly 3. Cylinder cavity 7. Follower 11. Spring 15. Mounting screws (4) 4. Gasket 8. Packing 12. Piston Rod 16. Magnet valve 1. ARMATURE 10. SCREW 2. ARMATURE 11. EXHAUST GUIDE VALVE STEM 3. SCREW 12. RETAINING RING 4. FRAME 13. FRICTION WASHER 5. VALVE BODY 14. WASHER 6. INLET VALVE 15. VALVE COIL 7. SPRING 16. TERMINALS 8. GASKET 17. VALVE SEAT 9. CAP PLUG SECTIONAL VIEW OF MAGNET VALVE Fig. 4 Fig. 5