Microbial Ecosystems - Pearson PDF
Document Details
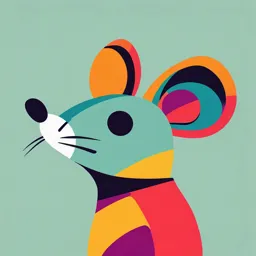
Uploaded by TrustyNephrite2315
University of Calgary
Tags
Summary
This document covers the principles of microbial ecosystems, including the unique characteristics of different environments like soil and the ocean. It also explores the diversity of bacteria with an emphasis on extremophiles. The impact of environmental stresses and the importance of microbial life are also included in this document.
Full Transcript
Microbial Ecosystems Sections 20.1-20.7 pp 652-666; Section 20.10-20.12 pp 671-679 MICROBIAL ECOSYSTEMS Habitat – Parts of an ecosystem suited to a particular group of populations Examples –soils, air, lakes, oceans, deep sediments (to 2-3 km), tissues of plants and animals...
Microbial Ecosystems Sections 20.1-20.7 pp 652-666; Section 20.10-20.12 pp 671-679 MICROBIAL ECOSYSTEMS Habitat – Parts of an ecosystem suited to a particular group of populations Examples –soils, air, lakes, oceans, deep sediments (to 2-3 km), tissues of plants and animals –only constrained by physical limits of temperature, water activity, pH – prokaryotes have much broader ecosystem ranges than eukaryotes Figure 20.13 Constraints on habitat composition Table 20.1 General Ecological Concepts Microbial communities can be described in terms of: –Species richness: the total number of different species present –Species abundance: the population size of each species in an ecosystem Figure 20.1 Guilds – Metabolically-related microbial populations – Perform key steps in biogeochemical cycles Figure 20.2 Environments and Microenvironments The small part of a local environment encountered by a given species Physicochemical conditions in a microenvironment are subject to rapid change, both spatially and temporally Resources in natural environments are highly variable, and many microbes in nature face a feast-or-famine existence Growth rates of microbes in nature are usually well below maximum growth rates defined in the laboratory Competition and cooperation occur between microbes in natural systems Soil Soils are composed of –Inorganic mineral matter (~40% of soil volume) –Organic matter (~5%) –Air and water (~50%) –Living organisms The availability of water often limits microbial activity in surface soils Energy source (organic matter) and inorganic nutrient availability are important limiting factors The rhizosphere, the area around plant roots where plants secrete sugars and other compounds, is rich in organic matter and microbial life Figure 20.11b Soil There are diverse microenvironments in soils and microbial diversity is very high Microcolonies 21 Sand 6 1 Distance (mm) 15 10 5 1 3 Sand Silt Silt 0 Clay particle Organic matter Sand 3 Water Air 6 Clay particle 6 3 0 3 6 Distance (mm) Figure 20.12 Figure 20.3 How to identify bacteria in an environmental sample based on SSU rRNA sequences Figure 13.25 Instead of a pure culture, extract from Instead of the SSU gene from Simultaneously sequence an environmental a single bacterium, you get a thousands of your SSU genes sample mixture of SSU genes from all with next-generation sequencing bacteria in your sample technologies Soils Phylogenetic community analysis –An OTU (operational taxonomic unit) is usually defined as a 16S rRNA gene sequence that differs from all other sequences by >3% (presumed to represent a species) –Molecular sampling indicates that there are 1000s to 100,000s of different microbial species (OTU’s) in most soils –Microbial diversity varies with soil type and geographical location Figure 20.14 Soil Bacterial and Archaeal Diversity Nearly all 16S rRNA genes recovered from environments like soil do NOT match cultured species at >97% identity (or 98.7%) There are about 13,000 cultured, named species BUT an estimated 1 million to 1 trillion uncultured species! The great plate count anomaly Why are so many bacteria hard to cultivate? 100% 40% of the biomass of marine phototrophs ~50% of the net primary production of the ocean Figure 20.25 Pelagic (open ocean) Bacteria The most abundant marine organoheterotroph is “Pelagibacter”, an oligotroph Oligotroph: an organism that grows best at very low nutrient concentrations “Pelagibacter” was long known from molecular DNA studies to be the most abundant bacterium in the ocean, but it took decades to finally grow a strain, and it has still not been validated as a true species. Contains proteorhodopsin, a form of rhodopsin that allows cells to use light energy to drive ATP synthesis Figure 20.30 The Marine Environment Deepwater Horizon oil spill Largest marine oil spill ever – Oil released as a plume at great depths – Bloom of hydrocarbon-degrading Gammaproteobacteria, Colwellia, and Cycloclasticus – Early growth of hydrocarbon-degrading bacteria reduced the environmental impact satellite image Figure 20.23 of oil slick The Deep Sea and Deep-Sea Sediments >75% of all ocean water is deep sea, lying primarily between 1,000 and 6,000 m depths Organisms that inhabit the deep sea must deal with – Low temperature – High pressure – Low nutrient levels – Absence of light energy- many lithotrophs! (Extremophiles!) Planktonic vs. Attached Bacteria Microbes can be planktonic (floating freely) or attached to a surface Biofilms are assemblages of bacterial cells adhered to a surface and enclosed in an adhesive matrix excreted by the cells. The matrix is typically a mixture of polysaccharides. Figure 20.4 Biofilms Biofilm formation is initiated by attachment of a cell to a surface followed by expression of biofilm-specific genes Genes encode proteins that initiate matrix formation Quorum sensing (sensing and responding to the population density) is critical in the development and maintenance of a biofilm The major quorum sensing molecules are acylated homoserine lactones Figure 4.16 Pseudomonas aeruginosa Biofilm Development Biofilms Bacteria form biofilms for several reasons – Self-defense Biofilms resist phagocytosis by immune system cells, and penetration of toxins (e.g., antibiotics) – Trap nutrients for microbial growth and help prevent detachment of cells in a flowing system – Allow bacterial cells to live in close association with one another- facilitates cross-feeding and symbioses Biofilms Biofilms have been implicated in several medical and dental conditions – Including periodontal disease, cystic fibrosis, tuberculosis, Legionnaires‘ disease, and Staphylococcus infections – big problem with medical implants like catheters and artificial joints In industrial settings, biofilms can slow the flow of liquids through pipelines and accelerate corrosion (e.g. oil pipelines- damages are in the billions of dollars per year) Very few effective antibiofilm agents are available Microbial mats are very thick biofilms – Built by phototrophic and/or chemolithotrophic bacteria – Phototrophic mats have existed for over 3.5 billion years (stromatolites) – Often occur in systems with low predation/grazing, e.g. extreme ecosystems Thiobacillus in the Cave and Basin, Banff Extremophiles Section 4.11- 4.16 pp 126-137 Extremophiles: Organisms that prefer conditions outside the limits of what is “normal” Q: Who decides what is normal? PHOTO: Christine Sharp IMAGE: Dewar Creek BC- Canada’s hottest geothermal spring (84oC) Environmental Stresses Temperature pH Pressure Water activity/[salt] Oxygen concentration Radiation Organisms that can grow in different ranges of these parameters are given the suffix –philes Organisms that can survive in different ranges of these parameters are given the suffix -tolerant Figure 4.20 The Cardinal Temperatures: Minimum, Optimum, and Maximum Figure 4.21 Temperature and Growth Response in Different Temperature Classes of Microorganisms Optimum 80oC Thermophilic habitats -compost, decaying organic matter -the deep biosphere (30oC increase for every 1 km depth) -geothermal systems hot springs, mud pools etc. undersea vents Photo P Dunfield, Tikitere NZ Hyperthermophiles (>80oC) Archaea- the champions… There are also hyperthermophilic Bacteria, but NO Eukarya grow above 62oC Methanopyrus kandlerii grows at 122oC Methanopyrus kandlerii https://microbewiki.kenyon.edu/index.php/File:Mkandcells.jpeg Photo. P. Dunfield Orakei Korako, NZ Different processes have different maxima. e.g. Photosynthesis stops at 73oC Figure 4.25 Figure 20.43 Hydrothermal Vents Thermophiles and hyperthermophiles Chemolithotrophic prokaryotes utilize inorganic materials from the vents (S, H2S, H2, Fe2+, Mn2+, etc.) Thriving animal and microbial communities associated with deep-sea hydrothermal vents Riftia pachyptila M. L. Jones, 1981 https://en.wikipedia.org/wiki/Riftia _pachyptila Bacteria are the primary producers, any animals are eating the microbes or have symbiotic microbes Bathymodiolus thermophilus Sabine Gollner, et al(2010). "Diversity of Meiofauna from the 9°50′N East Pacific Rise across a Gradient of Hydrothermal Fluid Emissions". PLoS ONE 5(8): e12321. doi:10.1371/journal.pone.0012321 Microbial Life at High Temperatures High temperature increases reaction rates and growth rates- to a point. High temperatures can also have negative effects on cells: Problems: 1) Proteins denature 2) DNA/RNA denature 3) Membranes too fluid Solutions: 1) Stronger bonds to stabilize proteins 2) Increase DNA/RNA stability (GC content, reverse gyrase) 3) Decrease membrane fluidity (tetra-ethers!) Microbial Life at High Temperatures Thermophilic enzymes and proteins function optimally at high temperatures. Features that provide thermal stability: Critical amino acid substitutions in a few locations provide more heat-tolerant folds Increased number of ionic bonds between basic and acidic amino acids resists unfolding in the aqueous cytoplasm highly hydrophobic interiors Production of solutes (e.g., di-inositol phosphate, diglycerol phosphate) helps stabilize proteins Smaller, more spherical proteins with less quaternary structure Microbial Life at High Temperatures DNA/RNA stability at high temperatures –Positive supercoiling of DNA via reverse gyrase IMAGE: Tatsuya Hirano, (2014) Condensins and the evolution of torsion- mediated genome organization, Trends in Cell Biology, 24: 727- 733, https://doi.org/10.1016/j.tcb.2014.06.007. ELSEVIER SCIENCE DIRECT JOURNALS_ U of C licensed RNA stability: Higher G=C content tRNA Fig 6.33 rRNA mRNA is linear, but secondary folding structure is critical to tRNA and rRNA function Images: Ribosome 50S LSU: David S. Goodsell, RCSB Protein Data Bank, public domain; 5S rRNA: Rfam database, public domain