Ecology PDF: Amphibian Population Decline
Document Details
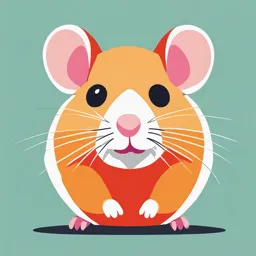
Uploaded by DarlingPegasus8544
Tags
Summary
This document contains ecology notes detailing the factors contributing to amphibian population decline, including parasites, nutrient run-off, and pesticides. It explores research on the effects of these factors on amphibian health and highlights the importance of managing host populations and controlling nutrient pollution. Studies are presented, focusing on the interactions between these environmental stressors and the impact on amphibian populations.
Full Transcript
Amphibian Population Decline 1/16/25 Initial Observations ​ Time and Place: In 1995, a middle school class in Henderson, Minnesota, observed deformed...
Amphibian Population Decline 1/16/25 Initial Observations ​ Time and Place: In 1995, a middle school class in Henderson, Minnesota, observed deformed frogs. ​ Findings: ○​ 30-40% of frogs were deformed (e.g., missing legs). ○​ Reported findings to the MN Pollution Control Agency. ○​ Deformed frogs were later identified in 46 U.S. states, impacting over 60 species of amphibians. ​ Global Concern: Research from the 1980s and 1990s highlighted declining amphibian populations worldwide, including pristine areas. Amphibians as Biological Indicators ​ Why Amphibians? ○​ Amphibians have permeable skin, making them sensitive to environmental changes like pollution or temperature shifts. ○​ They have dual habitats (aquatic and terrestrial), exposing them to multiple environmental stressors. ○​ Their health reflects ecosystem stability and potential threats to other species, including humans. Research Insights Ruth and Sessions (Deformities and Parasite Hypothesis) ​ Observation: Ribeiroia ondatrae (a trematode parasite) was linked to frog deformities. ​ Experimental Test: ○​ Glass beads mimicking parasite cysts were inserted into tadpoles. ○​ Result: Similar deformities observed, supporting the hypothesis that physical interference caused deformities. ​ Importance: ○​ Proved a direct mechanism for deformities. ○​ Highlighted parasites as a significant factor in amphibian decline. Johnson et al. (Snails as Hosts and Nutrient Pollution) Pond Survey: ​ Study Area: The research was conducted in Santa Clara, California, near San Francisco. Researchers surveyed 35 ponds for amphibian populations and health, focusing on deformities and their potential causes. ​ Findings: ○​ Presence of Deformities: Pacific tree frogs were found in 13 of the surveyed ponds, and 4 of these ponds contained frogs with deformities. ○​ Parasite Host Association: All 4 ponds with deformities also contained the aquatic snail Helisoma tenuis, an intermediate host for the trematode parasite Ribeiroia ondatrae. This pointed to a strong correlation between the presence of the snails and frog deformities. ○​ Initial Hypothesis: At first, researchers hypothesized that pollutants such as heavy metals, pesticides, or PCBs might be directly responsible for the deformities. However, the findings suggested a shift in focus toward the role of the parasite-host dynamics. Nutrient Pollution and Life Cycle Link: ​ Role of Nutrient Runoff: ○​ Fertilizers containing nitrogen and phosphorus from agricultural runoff entered the ponds. ○​ This nutrient influx caused algal blooms, significantly altering the pond ecosystems. ○​ Algal blooms provided an abundant food source for snails like Helisoma tenuis, increasing their populations in the affected ponds. ​ Parasite Transmission: ○​ The increased snail population facilitated the life cycle of Ribeiroia ondatrae, as these snails serve as an intermediate host for the parasite. ○​ The parasite’s life cycle involves multiple hosts: 1.​ Snail Host: Ribeiroia reproduces within the snails, releasing free-swimming larval forms. 2.​ Amphibian Host: The larval parasites penetrate amphibians (e.g., frogs), causing cyst formation in developing tadpoles. These cysts disrupt normal growth, leading to deformities such as missing limbs or extra legs. 3.​ Definitive Host: The parasite completes its life cycle in bird or mammal predators that consume infected amphibians. Experimental Confirmation: ​ Researchers further confirmed these observations by dissecting amphibians from the ponds. The dissections revealed that frogs with deformities were consistently infected with Ribeiroia cysts, providing additional evidence linking the parasite to the deformities. ​ Controlled experiments in the lab replicated these findings by exposing tadpoles to Ribeiroia larvae, directly linking the parasite to the deformities observed in the field. Key Insights: 1.​ Shift in Hypothesis: ○​ Initial assumptions that pollutants directly caused deformities were disproven. ○​ The study redirected its focus to the indirect effects of nutrient pollution (via agricultural runoff) and its role in enhancing parasite-host interactions. 2.​ Ecological Cascade: ○​ Nutrient runoff → Algal blooms → Increased snail populations → Higher Ribeiroia transmission → Increased amphibian deformities. ○​ This cascade illustrates how human activities (e.g., agriculture) can indirectly impact ecosystems and species health. 3.​ Importance of Host Dynamics: ○​ The presence of Helisoma tenuis as an intermediate host played a crucial role in the transmission of Ribeiroia and subsequent deformities in amphibians. ○​ Managing these host populations and controlling nutrient pollution could mitigate the issue. Kiesecker’s Experiments (Pesticides and Immune Suppression) Field Studies: ​ Objective: Kiesecker's field studies sought to correlate the presence of pesticides in natural environments (e.g., ponds) with increased deformity rates in amphibians and their susceptibility to parasites. ​ Findings: ○​ Deformity Rates: Field observations showed that frogs living in ponds exposed to pesticides exhibited a higher frequency of deformities, particularly in regions where pesticide levels were higher. ○​ Linking Pollution to Health: This suggested that not only the direct toxicity of pesticides was affecting the frogs, but also that exposure might be impairing their ability to combat infections, such as those caused by Ribeiroia ondatrae. ○​ Environmental Stressors: The study showed that ponds exposed to pesticides had more pronounced deformities among amphibians. These deformations were attributed to the synergistic effects of pollutants on both the amphibian's health and immune defenses. Lab Studies: ​ Objective: In a controlled laboratory setting, Kiesecker sought to isolate the effects of pesticide exposure on the immune system of amphibians, particularly looking for how immune suppression could make amphibians more susceptible to parasite infections. ​ Methodology: ○​ Exposure to Pesticides: Tadpoles were exposed to laboratory conditions where some were exposed to sublethal doses of commonly used pesticides (often simulating real-world contamination levels). ○​ Immunity Assessment: The researchers then tested the immune system's response, particularly focusing on how well the tadpoles could resist parasite infections, such as Ribeiroia ondatrae. ​ Findings: ○​ Reduced Immunity: Tadpoles exposed to pesticides showed a significant decrease in immune function. This was measured by a reduction in their ability to mount an immune response to the parasite. ○​ Increased Susceptibility to Infection: Tadpoles exposed to pesticides were more likely to develop infections from the parasite Ribeiroia ondatrae, leading to deformities, as the weakened immune systems could not effectively fend off the parasite. ○​ Mechanism of Impact: The reduction in immunity was believed to be due to direct toxicity to the tadpoles’ immune cells, making them less capable of fighting off infections. This effect is compounded when pesticides suppress not just immunity but other biological functions, leading to a general decrease in amphibian health. Conclusions: ​ Indirect Effects of Pesticides: The study concluded that while pesticides directly harm amphibians, their most insidious effect is indirect—they weaken the immune systems of amphibians, which in turn makes them more vulnerable to infections from parasites like Ribeiroia ondatrae. This suggests that the increasing prevalence of deformities in amphibian populations in pesticide-exposed areas is not solely due to direct effects of pesticides but also due to these environmental stressors facilitating parasite infection. ​ Synergistic Effects of Multiple Stressors: Beyond pesticides, Kiesecker’s research underscored how multiple environmental stressors—such as nutrient loading from agricultural runoff (nitrogen and phosphorus)—combine to exacerbate the effects of parasites. These stressors can create a "perfect storm" for amphibians: 1.​ Nutrient Loading: Increased nutrients in water promote algal blooms, which in turn support the growth of host species like snails (e.g., Helisoma tenuis), which are involved in the parasite’s life cycle. 2.​ Pesticides: These impair the immune system of amphibians, reducing their ability to resist the parasite. 3.​ Parasite Prevalence: This combination of weakened immunity and increased parasite transmission results in higher rates of deformities and mortality in amphibian populations. ​ Wider Implications: Kiesecker's findings highlight the importance of considering how anthropogenic activities, like pesticide use, interact with natural ecological processes. The cascading effects on species populations (e.g., amphibians) demonstrate how interconnected environmental factors are, with human-induced changes directly affecting species' survival and ecosystem health. Broader Ecological Impact: ​ Ecosystem Imbalance: The decline in amphibian populations, influenced by these compounded factors, has broader implications for ecosystem health. Amphibians are critical for controlling insect populations and serving as prey for higher trophic levels. Their decline can thus destabilize food webs and impact biodiversity. ​ Lessons for Conservation: Kiesecker's work emphasizes the need for integrated conservation strategies that address multiple environmental threats simultaneously. Protecting amphibian populations and their habitats requires reducing pesticide use, controlling nutrient pollution, and mitigating other anthropogenic pressures. ○​ Factors Contributing to Declines 1.​ Nutrient Runoff: ○​ Agricultural fertilizers increase algal growth, boosting snail populations and Ribeiroia infections. 2.​ Pesticides: ○​ Weakens amphibian immune defenses, making them more prone to parasites. 3.​ Climate Change: ○​ Alters precipitation and temperature patterns, stressing ecosystems and facilitating pathogen spread. 4.​ Habitat Loss: ○​ Reduces breeding and living spaces, intensifying population pressures. Key Takeaways ​ Interconnected Systems: Amphibian decline illustrates the interplay between pollutants, parasites, and ecological stressors. ​ Ecological Research: ○​ Observational studies (e.g., pond surveys) provide initial insights. ○​ Controlled lab experiments isolate causal factors. ○​ Field experiments verify ecological relevance in natural settings. ​ Broader Implications: Amphibian health serves as a sentinel for broader environmental and ecological issues. Climate and Weather 1/21/25 ​ Weather refers to short-term atmospheric conditions, including temperature, precipitation, humidity, and cloud cover. ​ Climate represents the long-term patterns and averages of weather, measured over decades. It also includes variability, such as daily, seasonal, and decadal cycles. ​ Example: A single hurricane is a weather event, but an increasing trend in hurricane intensity reflects climate change. Global Energy Balance ​ The sun is the primary energy source for Earth’s climate system. ​ Solar energy heats the Earth’s surface, while Earth loses energy through outgoing infrared radiation. ​ To maintain a stable climate, the energy gained from solar radiation must equal the energy lost. ​ Greenhouse Effect: ○​ Greenhouse gases (H₂O, CO₂, CH₄, N₂O) trap heat by absorbing and reradiating infrared radiation. ○​ While the greenhouse effect is essential for life on Earth, human activities have amplified it, leading to a rapid energy imbalance. Latitudinal Temperature Gradients ​ Uneven Heating: ○​ Near the equator, sunlight is concentrated and heats the surface more efficiently. ○​ At higher latitudes, sunlight is spread over a larger area, resulting in cooler temperatures. ​ These gradients drive atmospheric and oceanic circulation, which redistributes heat and moisture globally. Air Properties and Atmospheric Circulation ​ Warm Air vs. Cool Air: ○​ Warm air is less dense and rises, while cool air sinks. ​ Moist vs. Dry Air: ○​ Moist air contains water vapor, making it heavier than dry air. ​ Tropical Uplift: ○​ At the equator, warm, moist air rises and cools, leading to precipitation and low-pressure zones. This explains why tropical regions are hot and wet. Global Circulation Patterns ​ Hadley Cells: ○​ Rising air in the tropics moves poleward, cools, and descends around 30°N and 30°S, creating dry deserts. ​ Polar Cells: ○​ Cold air sinks at the poles, creating high-pressure zones with minimal precipitation. ​ Ferrell Cells: ○​ Found in mid-latitudes, they interact with Hadley and Polar cells to produce temperate climates. ​ These circulation cells divide the planet into distinct climatic zones: tropical, temperate, and polar. Ocean Currents and Wind Interaction Surface Currents: ○​ Winds drive surface ocean currents, moving heat and nutrients across the globe. ○​ Example: The Gulf Stream transports warm water from the tropics to Northern Europe, moderating its climate. ​ Vertical Water Movement: ○​ Downwelling: Warm, salty water sinks in polar regions, driving the global conveyor belt. ○​ Upwelling: Deep, nutrient-rich water rises to the surface, supporting marine ecosystems. ​ Heat Capacity: ○​ Water absorbs and retains heat better than land, moderating temperatures in coastal regions and creating maritime climates. Seasonality and Aquatic Environments ​ Earth’s 23.5° tilt relative to the sun causes seasonal changes in solar intensity and day length. ​ Aquatic Stratification: ○​ Water is most dense at 4°C, leading to layers that resist mixing. ○​ In summer, lakes stratify into: ​ Epilimnion: Warm surface water. ​ Hypolimnion: Cold, dense bottom water. ​ Thermocline: Transition zone between layers. ○​ Spring and Fall Turnover: ​ When temperatures equalize throughout the water column, mixing occurs, redistributing oxygen and nutrients. Longer-Term Climate Cycles ​ El Niño-Southern Oscillation (ENSO): ○​ During El Niño, trade winds weaken, allowing warm water to move eastward across the Pacific, disrupting global weather patterns. ○​ La Niña follows El Niño, intensifying normal trade wind patterns and often causing opposite climate effects. ​ Other Cycles: ○​ The North Atlantic Oscillation (NAO) and the Pacific Decadal Oscillation (PDO) also affect climate variability, impacting regions like Europe, North America, and the North Pacific. Regional Climate Trends ​ Coastal vs. Inland Climates: ○​ Coastal areas experience mild, stable temperatures due to the moderating effect of the ocean. ○​ Inland regions have greater seasonal and daily temperature variation (continental climate). ​ Mountains: ○​ Temperature decreases with elevation due to lower air pressure and density. ○​ The rain shadow effect: ​ Moist air rises and cools on the windward side of a mountain, causing precipitation. ​ The leeward side remains dry as descending air warms and holds less moisture. Vegetation and Climate Interactions ​ Albedo: ○​ Surfaces reflect solar radiation. Light-colored surfaces (e.g., ice, snow) have high albedo, reflecting more sunlight, while dark surfaces (e.g., forests) absorb more. ​ Evapotranspiration: ○​ Plants release water vapor through transpiration, transferring heat and moisture to the atmosphere. ○​ This process helps regulate air temperature and humidity, particularly in forested regions. Key Concepts and Connections ​ Energy and Heat Transfer: ○​ Differential heating by the sun drives atmospheric and oceanic circulation. ○​ Ocean currents act as "heat pumps," transferring warmth from the tropics to the poles. ​ Climate Zones: ○​ Atmospheric cells determine global patterns of wet and dry regions, influencing the distribution of ecosystems and biomes. Biomes 1/23/25 Learning Check 1.​ Question 1: Which town has a more maritime climate? ○​ Answer: B. Khatyrka ​ A maritime climate has smaller seasonal temperature changes due to the moderating influence of large bodies of water. ​ Khatyrka exhibits such characteristics, unlike Sangar, which has a more continental climate. 2.​ Question 2: How much does temperature change over the course of the year in Sangar? ○​ Answer: C. ~55°C ​ Sangar experiences extreme seasonal temperature variation, characteristic of continental climates. Key Concepts Biomes Defined ​ Large-scale terrestrial communities shaped by: ○​ Physical environment. ○​ Dominant plant growth forms (e.g., deciduous vs. succulent plants). ○​ Characteristics like leaf type and water storage capacity. ​ Key Idea: Biomes are best categorized by plant traits because plants cannot migrate. Convergence ​ Evolution of similar growth forms in different regions due to similar environmental selection pressures. ​ Example: Cacti in the Americas and euphorbs in Africa. ○​ Both evolved succulent stems, CAM photosynthesis, and spines independently. Impacts of Human Influence ​ Land-use changes have altered biome distributions. ​ Examples: ○​ Deforestation for agriculture reduces biodiversity. ○​ Grasslands degraded by overgrazing can lead to desertification. Terrestrial Biomes 1.​ Tropical Rainforest ○​ Location: Between 10°N and 10°S. ○​ Characteristics: ​ Abundant rainfall with one or two peaks annually. ​ High biomass and biodiversity, containing about 50% of Earth’s species. ​ Holds almost 40% of terrestrial carbon. ​ Competition for light is intense due to limited water constraints. ○​ Human Impact: ​ Forests are often converted to pastures or croplands, resulting in drier ecosystems with drastically reduced biodiversity. 2.​ Tropical Seasonal Forests and Savannas ○​ Characteristics: ​ Marked by seasonal rainfall, shorter trees, and deciduous foliage during dry seasons. ​ Thorn woodlands and tropical savannas with grasses interspersed with trees are common. ​ Fires and large herbivores like elephants shape savanna ecosystems by maintaining grass dominance. ○​ Human Impact: ​ Only half of these ecosystems remain, with many lost to agricultural conversion. ​ Example: The Cerrado in Brazil faces significant deforestation pressures. 3.​ Deserts ○​ Characteristics: ​ Water availability severely limits plant abundance. ​ Plant adaptations include succulent stems for water storage and drought-deciduous shrubs. ​ Convergence between cacti in the Western Hemisphere and euphorbs in the Eastern Hemisphere demonstrates independent evolution of similar traits. ○​ Human Impact: ​ Irrigation in some arid areas can lead to salinization, further degrading desert soils. 4.​ Temperate Grasslands ○​ Characteristics: ​ Grasses dominate due to frequent fires and grazing by herbivores such as bison. ​ Warm, moist summers and cold, dry winters. ​ Soil is highly fertile, supporting significant agricultural activity. ○​ Human Impact: ​ Overgrazing can exceed regrowth capacity, causing desertification. ​ Conversion to croplands often removes native grass species. 5.​ Temperate Shrublands and Woodlands ○​ Characteristics: ​ Cool, wet winters and hot, dry summers. ​ Sclerophyllous leaves minimize water loss and deter herbivores. ​ Fire-adapted ecosystems; without regular fires, shrublands may transition to forests. ○​ Human Impact: ​ Urban development has significantly reduced these biomes. 6.​ Temperate Deciduous Forests ○​ Characteristics: ​ Dominated by deciduous trees that shed leaves in autumn and regrow in spring. ​ Found in areas with sufficient rainfall to support tree growth. ○​ Human Impact: ​ Extensive logging and development lead to permanent forest loss. ​ Forest regrowth in some regions as agriculture shifts to tropical areas. 7.​ Temperate Evergreen Forests ○​ Characteristics: ​ Composed of evergreen trees adapted to nutrient-poor soils. ​ Fire-adapted ecosystems, with intervals averaging 200 years. ○​ Human Impact: ​ Logged extensively for wood and paper pulp. ​ Climate change increases fire frequency and severity. 8.​ Boreal Forests (Taiga) ○​ Characteristics: ​ Dominated by conifer species and underlain by permafrost, preventing drainage. ​ Peat bogs commonly form in low-lying areas. ​ Fires caused by lightning are a natural part of the ecosystem. ○​ Human Impact: ​ Boreal wildfires are increasing in intensity due to climate change, with severe impacts on air quality and carbon storage. 9.​ Tundra ○​ Characteristics: ​ Short summers with low-growing vegetation like sedges, grasses, and mosses. ​ Permafrost is widespread, limiting root penetration. ○​ Human Impact: ​ Arctic warming at almost double the global average disrupts tundra ecosystems. Freshwater Biological Zones Aquatic Biomes and Stream Dynamics 1.​ Riparian Vegetation: ○​ Provides organic detritus and shade to the aquatic ecosystem. ○​ Affects nutrient availability and water temperature regulation. 2.​ Main Channel: ○​ Characterized by flowing water where swimming organisms like fish dominate. ○​ Organisms are adapted to currents and oxygen-rich environments. 3.​ Benthic Zone: ○​ The bottom layer of the stream or river, consisting of sediments. ○​ Insect larvae and crustaceans thrive here, feeding on organic material. Hyporheic Zone: ○​ A region beneath and alongside the streambed where groundwater and surface water mix. ○​ Populated by rotifers, copepods, and other small invertebrates adapted to the substrate. Stream Order System: ○​ First-order streams: Originate at high elevations, forming the smallest channels. ○​ Second-order streams: Created when two first-order streams join. ○​ Third-order streams: Formed when two second-order streams converge, and so on, leading to larger rivers. Stream Connectivity: ○​ Streams connect terrestrial ecosystems to marine systems by transporting nutrients and detritus downstream. ○​ Changes in stream order reflect transitions in nutrient sources and ecological processes. 1.​ Key Features ○​ Defined by water velocity, depth, temperature, clarity, and chemistry. ○​ Examples: ​ Streams connect terrestrial to marine systems. ​ Lakes are categorized as nutrient-rich (shallow) or nutrient-poor (deep). 2.​ Stream Characteristics ○​ River Continuum Concept: ​ Detritus is important in smaller streams. ​ Algae and macrophytes dominate in larger rivers. ○​ Organisms: ​ Shredders break down leaves in headwaters. ​ Collectors filter particles in larger streams. 3.​ Lakes ○​ Zones: ​ Pelagic: Open water with plankton. ​ Plankton – small organisms suspended in water some are photosynthetic, like some algae (phytoplankton) – limited to photic zone Zooplankton – eat bacteria, fungi in form of detritus ​ Littoral: Nearshore, supports diverse plant life. ​ Benthic: Bottom, low oxygen. ○​ Life: ​ Phytoplankton (photosynthetic) in the photic zone. ​ Zooplankton consume bacteria and fungi. Marine Biological Zones 1.​ Key Features ○​ Cover 71% of Earth’s surface. ○​ Zones defined by depth, light, and proximity to shorelines. 2.​ Nearshore Zones ○​ Examples: ​ Estuaries: Mix of salt and freshwater, high productivity. ​ Salt Marshes: Grass-dominated, adapted to saline conditions. ​ Mangroves: Provide critical coastal protection and habitats. 3.​ Open Ocean ○​ Photic Zone: ​ Supports photosynthetic organisms like phytoplankton. ​ Nekton (swimming organisms) and zooplankton thrive here. ○​ Deep Pelagic Zone: ​ No light; high pressure and cold temperatures. ​ Crustaceans and fishes dominate. ○​ Benthic Zone: ​ Bottom zone; organisms adapt to extreme conditions. 1.​ Salt Marshes & Mangroves: ○​ Salt Marshes: ​ Dominated by grasses adapted to saline environments. ​ Provide critical ecosystem services such as coastal protection and habitat for diverse species. ○​ Mangroves: ​ Found in tropical and subtropical regions. ​ Roots stabilize coastlines and support marine life by providing shelter and nutrients. 2.​ Rocky Intertidal Zones: ○​ Characterized by tidal changes that expose organisms to alternating periods of submersion and exposure. ○​ Organisms like barnacles, mussels, and sea stars are adapted to cling to surfaces and survive wave action. 3.​ Sandy Shores: ○​ Dynamic environments with shifting sands and limited plant growth. ○​ Home to burrowing organisms like crabs, worms, and clams. 4.​ Shallow Ocean Zones: ○​ Corals: ​ Form coral reefs in warm, shallow waters. ​ Provide habitat for numerous marine species and are highly sensitive to temperature changes and ocean acidification. ○​ Kelp Forests: ​ Found in cooler coastal waters with nutrient-rich upwellings. ​ Support diverse marine life by creating complex underwater structures. ○​ Seagrass Beds: ​ Found in shallow, sandy environments with clear water. ​ Serve as nurseries for juvenile fish and stabilize sediments. In Class Assignment Question 1 How would you expect the area and geographic locations of the tropical rainforest, tropical seasonal forest, and desert biomes to change if the Hadley cells expanded north and south? If the Hadley cells expand north and south: ​ Tropical rainforests: These would shrink in area as the zones of high precipitation would move poleward. Tropical rainforests would retreat from their current locations near the equator. ​ Tropical seasonal forests: These forests may expand into areas previously occupied by tropical rainforests and deserts due to the intermediate levels of precipitation and seasonal variability. ​ Deserts: These would expand poleward, taking over regions that were previously temperate grasslands or tropical seasonal forests, as descending dry air at the edges of the Hadley cells increases aridity. Question 2 Projections for the movement of the Hadley cells suggest an expansion rate of around 0.8° latitude per decade has occurred since 1979. If this rate of movement of the Hadley cell in the Northern Hemisphere continues, how far north would you expect the desert biome to occur by 2080? 1.​ Expansion Rate: 0.8° latitude per decade. 2.​ Time from 1979 to 2080: 101 years or 10.1 decades. 3.​ Total expansion: 0.8×10.1=8.08∘0.8 \times 10.1 = 8.08^\circ0.8×10.1=8.08∘. If deserts previously reached about 30°N, they are expected to expand to approximately 30+8.08=38.08∘N30 + 8.08 = 38.08^\circ N30+8.08=38.08∘N by 2080. Question 3 Graph the data as climate diagrams to estimate what would happen if the Hadley cell moved according to the prediction from Question 2. What biomes would you expect at each location? I'll generate climate diagrams for Zaragosa, Big Lake, and Spur and assess the impact of the Hadley cell's northward movement. Matplotlib Chart Biomes Expected with Hadley Cell Expansion 1.​ Zaragosa (28.5°N):​ As the Hadley cell expands, Zaragosa may transition toward a more desert-like biome with reduced precipitation and higher aridity. 2.​ Big Lake (31.2°N):​ Big Lake, currently in a transition zone, may move closer to a desert biome with drier conditions and reduced water availability for plant growth. Tempered grass land 3.​ Spur (33.5°N):​ Spur may transition from a temperate grassland to a drier temperate shrubland or even a semi-arid biome if the precipitation levels decrease further.Temperate grass land Coping with Environmental Variation: Temperature and Water 1/28/25 1. Avoidance vs. Tolerance ​ Avoidance: ○​ Strategies to escape environmental stress temporarily or spatially. ○​ Examples: ​ Plants complete their lifecycles quickly during favorable conditions (e.g., annual plants) or shed leaves during drought to conserve water. ​ Animals migrate to warmer or cooler climates to avoid temperature extremes. ​ Tolerance: ○​ The ability to endure stress through structural or physiological adaptations. ○​ Example from slides: ​ Woolly plants in tundra environments utilize boundary layers (low growth and fuzzy leaves) to reduce wind exposure, trap heat, and reflect sunlight. ​ Hemlock plants develop waxy coatings to prevent water loss. 2. Acclimation vs. Adaptation ​ Acclimation: ○​ Definition: Temporary, reversible physiological changes that allow individuals to cope with stress. ○​ Examples: ​ Goldfish acclimating to changing water temperatures within their tank. ​ Gil dogs panting to cool down and reduce heart rate in response to heat. ​ Adaptation: ○​ Definition: Long-term, irreversible genetic changes in populations due to natural selection. ○​ Examples: ​ Sea otters with denser fur survive better in cold water and pass this trait to future generations, creating populations with increased thermal insulation. ​ Shrubland plants develop drought tolerance over generations, ensuring survival in dry climates. ​ Key Comparison: ○​ Acclimation happens within an individual’s lifetime and is reversible (e.g., humans adapting to high altitudes). ○​ Adaptation occurs over multiple generations and results from genetic selection. 3. Thermal Limitations to Life ​ Organisms’ internal temperatures typically range from -2°C to 50°C, with some exceptions: ○​ Too cold: ​ Cells freeze, become "leaky," and lose function due to ice formation. ​ Example from slides: Ice fish produce antifreeze proteins that prevent their cells from freezing. ○​ Too hot: ​ Enzymes denature, halting key processes like photosynthesis and metabolism. How Plants Regulate Temperature: 1.​ Stomatal Function: ○​ Stomata open during the day to release water vapor, cooling the plant while taking in CO2. ○​ Rising CO2 levels reduce water loss, increasing photosynthetic efficiency (CO2 fertilization effect). 2.​ Leaf Modifications: ○​ Color Changes: ​ Red pigments in autumn act as a natural sunscreen, reducing sunlight absorption. ○​ Waxy Coatings: ​ Reflect sunlight and minimize water loss. ○​ Hairs/Boundary Layers: ​ Trap heat, deflect wind, and reduce exposure. ​ Example from slides: Woolly plants like lousewort grow close to the ground in tundra regions, minimizing wind effects and retaining warmth. 4. Ectotherms vs. Endotherms ​ Ectotherms: ○​ Rely on external heat sources to regulate body temperature. ○​ Examples: ​ Tuna generate some internal heat to maintain a slightly higher body temperature than their surroundings. ​ Greenland sharks have extremely slow metabolisms, allowing them to thrive in icy water for centuries. ​ Endotherms: ○​ Generate heat metabolically, allowing them to maintain constant internal temperatures regardless of external conditions. ○​ Key Challenges: ​ High energy demands for food and metabolic heat production. ​ Smaller animals lose heat faster due to their higher surface area-to-volume ratio. ○​ Examples: ​ Olympic runners: Athletes in hot climates (e.g., Athens 2004) tend to be shorter and leaner, following Bergmann’s Rule, which helps dissipate heat. ○​ Adaptations: ​ Insulation via dense fur or fat layers. ​ Torpor or hibernation to conserve energy and reduce metabolic demands in extreme cold. 5. Water Flow and Stress (Expanded with Sugar Details) ​ Water Movement: ○​ Water flows along energy gradients: ​ Gravity: Water flows downhill. ​ Pressure: Water moves from high to low pressure. ​ Osmotic Potential: Water moves toward regions with higher solute concentrations. ​ Matric Potential: Water clings to surfaces like soil particles or cell walls. ​ How Plants Cope with Water Stress: ○​ Stomatal Regulation: ​ Plants lose approximately 80 molecules of water for every 1 molecule of CO2 absorbed. ​ Rising atmospheric CO2 reduces this ratio, conserving water and improving efficiency during photosynthesis (CO2 fertilization effect). ○​ Osmotic Adjustments: ​ During drought, plants allocate solutes like sugars to their cells to maintain water potential. ​ Sugar Use: ​ Stored sugars stabilize cells by attracting water (osmotic regulation). ​ Plants convert glucose into starch for energy storage, which can be broken down and used during stress conditions like drought. ​ This helps plants survive longer periods without access to water. ​ How Animals Cope with Water Stress: ○​ Marine animals excrete salts to balance water levels. ○​ Terrestrial animals conserve water by producing highly concentrated urine or relying on physiological mechanisms like sweating and panting. CO2 Fertilization and Sugar Production (Linked to the Sugar Section): ​ Higher CO2 levels result in better water use efficiency for plants. ​ Plants use the absorbed CO2 to produce glucose (sugar), which is converted into: ○​ Starch: A storage molecule for energy, used in times of stress. ○​ Fructose: Another sugar that contributes to cellular function and osmotic balance. 6. Biome Shifts with Climate ​ Example Question from Slides: ○​ If Florida’s average temperature rose to 19°C and precipitation increased to 275 cm/year: ​ The biome would shift from temperate evergreen forests to tropical seasonal forests or tropical rainforests. ​ Increased water and warmth support lush vegetation growth and biodiversity. Sources of Energy 1/30/25 ​ Autotrophs: Convert energy from sunlight (photosynthesis) or inorganic compounds (chemosynthesis) into chemical energy. ​ Types of Autotrophs: 1.​ Photoautotrophs: Use sunlight for energy (e.g., plants, algae, cyanobacteria). 2.​ Chemoautotrophs: Use inorganic molecules like hydrogen sulfide or ammonia for energy (e.g., deep-sea vent bacteria, nitrifying bacteria). ​ Heterotrophs: Obtain energy by consuming organic compounds from other organisms. Types of Heterotrophs 1.​ Detritivores: Consume nonliving organic matter (e.g., earthworms, fungi). 2.​ Parasites & Herbivores: Feed on live hosts without necessarily killing them (e.g., leaf miners, mistletoe). 3.​ Predators: Capture and consume live prey. Photosynthesis ​ Equation:6CO2 +6 H2O = C6H12O6 + 6O2 ​ Rubisco: Most abundant enzyme on Earth, crucial for CO₂ fixation. ​ Photosynthesis and Light: ○​ Plants acclimate to different light levels. ○​ Light can be damaging if excess electrons are not quenched in the Calvin cycle due to closed stomata, leading to oxidative damage. ○​ Leaf Adaptations to reduce light damage: ​ Adjust leaf angle ​ Change color (e.g., fall foliage) ​ Develop lighter or more reflective surfaces ​ Temperature & Nutrient Limitations: ○​ Temperature affects photosynthesis rates. ○​ Nitrogen and phosphorus availability influence plant growth. ○​ High nitrogen levels attract herbivores. Three Major Photosynthetic Pathways 1.​ C3 Plants (~90%): ○​ Most common pathway. ○​ Rubisco binds both CO₂ and O₂, leading to photorespiration (energy loss). ○​ More efficient in high CO₂ environments but struggles in low CO₂. 2.​ C4 Plants (~5%): ○​ Minimizes photorespiration and water loss. ○​ Uses extra energy to concentrate CO₂ for photosynthesis. ○​ Efficient in hot, dry environments (e.g., maize, sugarcane, grasses). 3.​ CAM Plants (~5%): ○​ Takes in CO₂ at night, performs photosynthesis during the day. ○​ Conserves water but requires extra energy. ○​ Some plants are facultative CAM, switching between C3 and CAM based on water availability. Heterotroph Energy Acquisition ​ Energy Sources: ○​ Carbohydrates, proteins, fats provide the most energy. ○​ Secondary compounds in plants (toxins) deter herbivores but are not a good energy source. ​ Animal vs. Plant Cells: ○​ Animal cells contain more accessible energy than plant cells. ○​ Herbivores need to eat more food than carnivores to meet energy needs. ○​ Carnivores expend more energy hunting but eat more energy-rich food. ​ Microbial Digestion: ○​ Bacteria, fungi, and archaea release enzymes to digest organic matter outside their bodies. Adaptations for Finding & Processing Food 1.​ Mobility: Helps locate and capture food. 2.​ Mouth Shape/Size: Adapted for specific diets. 3.​ Digestion: ○​ Heterotrophs must transform food into simpler molecules. ○​ Proteins, carbohydrates, and fats are broken down into amino acids, simple sugars, and fatty acids. ○​ Herbivores consume fiber-rich plants, requiring longer digestive tracts to maximize absorption. ○​ Increased digestive surface area allows for longer food processing times. 4.​ Tool Use: Some animals use tools to increase energy efficiency (e.g., primates using sticks to extract insects). Analysis of Graphs and Charts from Lecture Photosynthesis Rates and Environmental Conditions ​ Graphs show how temperature, CO₂ levels, and light intensity impact photosynthesis. ​ High CO₂ levels increase photosynthesis efficiency in C3 plants. ​ C4 plants maintain higher efficiency in dry, low CO₂ conditions. Osmoregulation in Saltwater Fish ​ Chart illustrates how fish actively excrete salts to maintain water balance. ​ Saltwater fish have specialized gill cells that pump excess salt out of their bodies. Nutrient Availability and Plant Growth ​ Graphs show how nitrogen and phosphorus levels impact plant biomass. ​ High nitrogen leads to rapid growth but can attract herbivores. ​ Phosphorus is crucial for energy transfer in plants (ATP production). Heterotroph Energy Trade-offs ​ Data shows that carnivores need less food overall but spend more energy hunting. ​ Herbivores require large amounts of plant material due to lower energy content per gram. Additional Class Discussion Notes ​ CAM plants can switch to C3 photosynthesis when water is abundant. ​ C4 plants evolved multiple times in response to different dry environments. ​ Energy trade-offs exist: carnivores need less food but expend more energy hunting. ​ The spotted salamander has a symbiotic relationship with algae, making it one of the few photosynthetic vertebrates. ​ Fungi play a critical role in breaking down organic material externally. Evolution and Ecology 02/04/25 Detailed Notes on Class 7: Ecology and Evolution I. Introduction to Ecology and Evolution ​ The class covers key concepts in evolution, focusing on allele frequency changes, adaptation, and the mechanisms of evolution. ​ Case studies like plant acclimation to light levels and tuskless elephants in Gorongosa provide real-world examples. II. Bjorkman’s Study on Plant Acclimation to Light ​ Researchers measured photosynthesis in plants exposed to three light levels: high (red line), medium (blue line), and low (orange line). ​ Key Question: At what light intensity do high-light plants photosynthesize at twice the rate of low-light plants? ○​ Answer choices: 100, 300, 600, or 1,000 μmol/m²/s III. Evolution in Gorongosa: The Case of Tuskless Elephants ​ Study Reference: Campbell-Staton, S.C., et al. (2021) Science, 374: 483-487. ​ Key Findings: ○​ Before the civil war, a small proportion of female elephants were tuskless. ○​ After the war, tuskless females increased significantly due to selective survival from poaching. ○​ Tuskless females were significantly more likely to survive than their tusked counterparts. ○​ Consequences for Gender Ratios: Tusklessness is linked to the X chromosome and is lethal in males, skewing population ratios. ○​ Genetic Basis: The MEP1a locus is under selection. IV. What is Evolution? ​ Definition: Evolution is the change in allele frequencies over time. ​ Key Principles: 1.​ Populations evolve, individuals do not. 2.​ Adaptation vs. Acclimation: ​ Adaptation: Genetic change over generations. ​ Acclimation: Temporary physiological adjustment. 3.​ Time Scale: Evolution can be rapid (few generations) or take millennia. 4.​ Evolution is not always adaptive (some changes result from neutral processes). V. Mechanisms of Evolution 1.​ Mutation ○​ Provides raw material for evolution. ○​ Occurs in 1 per 10,000 to 1,000,000 gene copies. ○​ Generally weak in changing allele frequencies alone. 2.​ Genetic Drift ○​ Random fluctuations in allele frequencies. ○​ Greater effect in small populations. ○​ Example: Prairie Chickens – habitat loss caused increased harmful alleles. 3.​ Natural Selection ○​ Reduces harmful mutations and increases beneficial ones. ○​ Acts on phenotypes, leading to genetic shifts. ○​ Example: Andean goose – evolved special hemoglobin for high altitudes. 4.​ Gene Flow ○​ Alleles move between populations (via individuals or gametes). ○​ Effects: ​ Populations become more similar. ​ New alleles enter a population (similar to mutation). ○​ Example: Sky Islands – isolated populations experience divergence due to limited gene flow. VI. Speciation ​ Definition: Formation of new species due to barriers in gene flow. ​ Key Factors: ○​ Physical barriers (geographic isolation). ○​ Reproductive barriers (behavioral or genetic incompatibility). VII. Limits to Evolution 1.​ Lack of Genetic Variation ○​ Evolution depends on existing genetic diversity. ○​ Mutations are random, not goal-directed. 2.​ Evolutionary Constraints ○​ Evolution builds on existing traits (e.g., dolphins cannot suddenly evolve gills). 3.​ Ecological Trade-offs ○​ Organisms allocate limited resources between survival and reproduction. Summary ​ Evolution is driven by mutation, genetic drift, natural selection, and gene flow. ​ Real-world examples: Elephant tusklessness, plant light acclimation, Andean geese. ​ Evolutionary limits: Constraints due to genetic availability, history, and trade-offs. Femoral pounds Cascading match in the ecosystem based on time Class 8: Life History Life History Overview Life history is the study of the patterns and strategies organisms use for growth, development, reproduction, and survival. Each species follows a unique life history strategy based on how they allocate resources between these factors. These strategies evolve due to genetic variation and environmental conditions, which influence reproductive success and survival. Within a species, individuals may display different life history strategies due to genetic diversity or external pressures like climate, food availability, or predation. Natural Selection and Life History Strategies The learning check question in the presentation highlights the role of natural selection in shaping life history traits. Natural selection acts on traits like coat color in mice if: 1.​ The coat color affects survival, such as by providing camouflage in the environment. 2.​ There is genetic variation in coat color, allowing certain individuals to be favored over others. This demonstrates how an organism’s physical traits, if heritable, can contribute to its fitness—its ability to survive and reproduce. In a population, traits that increase fitness become more common over generations. Key Life History Characteristics Life history strategies revolve around several key traits: ​ Age and size at sexual maturity – Determines when an organism begins reproducing. ​ Amount and timing of reproduction – Some organisms reproduce early and frequently, while others delay reproduction and invest more in fewer offspring. ​ Survival and mortality rates – These influence how many offspring need to be produced to maintain population levels. Life history traits exist on a spectrum and are shaped by trade-offs in energy allocation. Organisms must balance investment in growth, reproduction, and survival, as no species can maximize all three simultaneously. Environmental Influence on Life History Strategies Organisms of the same species can exhibit different life history strategies depending on their environment. This variation can be seen through: ​ Morphs – Alternative physical forms of an organism that arise due to environmental conditions rather than genetic differences. ​ Phenology – The timing of seasonal life history events such as reproduction, migration, and flowering. Changes in climate and habitat conditions can alter phenology, affecting species interactions and survival. An example given in the presentation is the spadefoot toad, which can develop into different morphs depending on water availability. If ponds dry quickly, some larvae accelerate their development to metamorphose sooner, while others stay in a larval state longer under stable conditions. Reproductive Modes Reproduction plays a central role in life history strategies and exists in two main forms: 1.​ Asexual Reproduction – Offspring are clones of the parent, produced through binary fission (in prokaryotes and protists) or vegetative propagation (in plants). Some organisms, like corals, can reproduce both sexually and asexually. ○​ Advantages: Rapid reproduction, no need for a mate. ○​ Disadvantages: Lack of genetic diversity makes populations vulnerable to environmental changes. 2.​ Sexual Reproduction – Involves the combination of genetic material from two parents, leading to genetic diversity. ○​ Isogamy: Gametes are equal in size. ○​ Anisogamy: One gamete (usually the egg) is much larger and provides nutrients for the developing embryo, while the sperm is smaller and more mobile. ○​ Advantages: Increases genetic variation, which helps populations adapt to environmental changes. ○​ Disadvantages: Requires more time and energy, finding mates can be difficult, and only half of an individual's genes are passed to offspring. A major evolutionary advantage of sexual reproduction is genetic recombination, which reshuffles genes to create new combinations, enhancing adaptability. Complex Life Cycles Some organisms go through distinct stages with different forms and ecological roles. Complex life cycles involve at least two stages with different body forms, habitats, and diets. This strategy is common in insects, marine invertebrates, amphibians, and some fish. A key example is metamorphosis, an abrupt developmental transition between larval and juvenile stages. In species like frogs, the larval stage (tadpole) is specialized for feeding and growth, while the adult is adapted for dispersal and reproduction. Complex life cycles allow organisms to exploit different ecological niches at different stages, reducing competition among individuals of the same species. Trade-offs in Life History Strategies Because resources are limited, organisms face trade-offs between survival, growth, and reproduction. Some key trade-offs include: ​ Number of Offspring vs. Size of Offspring – Producing many small offspring increases chances of survival through sheer numbers, but individual offspring receive fewer resources. In contrast, producing fewer, larger offspring allows for more parental investment, increasing survival chances per individual. ​ Reproductive Effort vs. Parental Survival – High reproductive investment can reduce the survival of the parent. Studies, such as David Lack’s research on birds, show that increasing clutch size (number of eggs per reproductive event) leads to lower survival rates in offspring due to resource limitations. ​ Reproductive Investment vs. Future Reproduction – Some species, like annual plants and the giant Pacific octopus, reproduce once and die (semelparity), investing all resources into a single reproductive event. Others, like mammals and many trees, reproduce multiple times over their lifespan (iteroparity), balancing current and future reproductive efforts. Trade-offs are seen in trees as well—years with high cone production lead to reduced growth because resources are diverted to reproduction. r-Selection vs. K-Selection Life history strategies can be categorized into r-selected and K-selected species, based on their reproductive patterns and population dynamics. ​ r-Selected Species: ○​ Short lifespans, rapid development, early reproduction. ○​ High reproductive rates but low parental investment. ○​ Often found in unstable environments where rapid reproduction helps ensure survival. ○​ Examples: Insects, small rodents, weedy plants. ​ K-Selected Species: ○​ Long lifespans, slow development, delayed reproduction. ○​ Low reproductive rates but high parental investment. ○​ Thrive in stable environments where competition is high. ○​ Examples: Large mammals, reptiles, oak trees. This classification is not absolute; many species show characteristics of both strategies depending on their environment. Early Life Stages and Survival Strategies Juvenile organisms face high risks from predation, competition, and environmental stress. Strategies to improve survival include: ​ Parental Provisioning – Investing resources in eggs or embryos (e.g., yolk in bird eggs). ​ Parental Care – Protecting and feeding offspring to increase survival rates. ​ Dispersal – Moving offspring away from the birthplace to reduce competition and avoid predators or disease. Studies show that marine snails with swimming larvae had larger geographic ranges and lower extinction rates compared to those that developed directly into crawling juveniles. Specialization at Different Life Stages Many species adapt different life stages for specific functions: ​ Feeding specialization – Insects often have larvae specialized for feeding and adults adapted for dispersal and reproduction. ​ Niche switches – Some organisms, like the mole salamander, retain larval characteristics while maturing sexually (paedomorphosis) if the larval habitat is highly favorable. Research on marine organisms shows that juveniles may occupy different habitats than adults to reduce predation risk. For example, smaller juvenile fish often hide in algae clumps, while larger adults move into rocky areas. Number of Reproductive Events in a Lifetime Species can be categorized based on how many times they reproduce: ​ Semelparous species – Reproduce once and die, investing all energy into a single reproductive event (e.g., annual plants, agave, octopus). ​ Iteroparous species – Reproduce multiple times, balancing reproductive effort over their lifetime (e.g., most mammals, trees). This distinction reflects different evolutionary strategies for maximizing reproductive success in different environments. What is Basic Data Manipulation, and how do you calculate the mean and standard deviation? Basic Data Manipulation involves working with data to extract key measures. Mean: The sum of all data values divided by the number of values. It represents the average. Mean = Sum of all values / Number of values. Standard Deviation: A measure of how much data points differ from the mean. A small standard deviation means values are close to the mean; a large one means they are spread out. New cards 2 What are the key steps in Reading and Interpreting Graphs? Define axes and trend. Axes: The horizontal (x-axis) and vertical (y-axis) lines on a graph that represent variables. Trend: A pattern or direction shown in data points. Steps: Identify what the axes represent, look for patterns or trends in data, and compare different data points. New cards 3 What is Experimental Design? Define hypothesis, variables, and controls. Experimental Design: Planning experiments to test a hypothesis with measurable results. Hypothesis: A testable prediction or explanation. Variables: Independent Variable: What you change. Dependent Variable: What you measure. Controlled Variables: Kept constant for accuracy. Controls: A baseline for comparison to determine the effect of the independent variable. New cards 4 What is the key focus of Chapter 1? Define interconnected and unintended consequences. Interconnected: All parts of nature are linked; changes in one part affect others. Unintended Consequences: Effects that occur due to changes but were not anticipated. The key focus of Chapter 1 is that nature is interconnected, and changes to systems often lead to multiple unintended consequences. New cards 5 What is the difference between climate and weather? Define both terms. Climate: Long-term patterns and averages of temperature, precipitation, and atmospheric conditions over a region for several decades or longer. Weather: Short-term atmospheric conditions lasting from hours to a few days. Key Difference: Climate describes long-term trends, while weather refers to daily or short-term changes. New cards 6 What is the global energy balance (Fig. 2.4)? Define inputs and outputs in this system. Global Energy Balance: The balance between incoming energy from the sun and outgoing energy radiated back to space, regulating Earth's climate. Inputs: Solar radiation absorbed by the Earth’s surface and atmosphere. Outputs: Infrared radiation (heat) emitted from the Earth back into space. New cards 7 What are the types of heat fluxes? Define sensible heat, latent heat, conduction, and convection. Heat Flux: The transfer of heat energy between Earth’s surface and the atmosphere. Sensible Heat Flux: Heat transfer through the air that can be felt and measured. Latent Heat Flux: Heat transfer when water changes phases. Conduction: Direct transfer of heat between objects in contact. Convection: Heat transfer through the movement of fluids. New cards 8 What does water’s high heat capacity mean, and how does it affect life in water compared to air? Define heat capacity. Heat Capacity: The ability of a substance to absorb and retain heat without a large change in temperature. Water’s High Heat Capacity: Water absorbs and stores large amounts of heat with only a small temperature change, making aquatic environments more stable. Impact on Life: Aquatic organisms experience less extreme temperature variation compared to those living in air. New cards 9 What are the consequences of ocean currents? Define nutrients and climate regulation. Nutrients: Chemical substances that provide essential nourishment for organisms. Climate Regulation: The process by which natural systems maintain global temperature balance. Ocean Currents: Move nutrients and organisms, regulate global climate by transferring heat, and influence weather patterns. New cards 10 How do mountain ranges shape local climate (Figures 2.14 and 2.16)? Define windward side, leeward side, and rain shadow. Mountain Ranges: Affect local climates by blocking airflow and creating distinct weather patterns. Windward Side: Receives more precipitation as it faces prevailing winds. Leeward Side: Drier because it lies in the rain shadow where moisture is lost. Rain Shadow Effect: a weather pattern that occurs when a mountain range blocks moisture-laden winds, resulting in reduced rainfall on the downwind side of the range New cards 11 How do vegetation and climate interact (Figure 2.19)? Define albedo and evapotranspiration. Vegetation: Plants influence local climate by altering heat and moisture. Albedo: The fraction of solar energy reflected from the Earth's surface. Evapotranspiration: (Sum of water loss) The combined process of evaporation and transpiration that transfers moisture into the atmosphere. New cards 12 What is a biome, and how are they defined? Differentiate between terrestrial and aquatic biomes. Biome: A large ecological area with distinct climate, vegetation, and organisms adapted to that environment. Terrestrial Biomes: Land-based biomes classified by dominant vegetation and climate. Aquatic Biomes: Water-based biomes classified by salinity, depth, and flow. New cards 13 What are some key adaptations in different biomes? Define fire adaptation and CAM plants. Adaptation: A trait that helps an organism survive and reproduce. Fire Adaptation: Traits that help plants survive after a fire. CAM Plants: Plants that minimize water loss by opening stomata at night. New cards 14 How does land-use change impact Earth’s biomes? Define deforestation and desertification. Land-Use Change: Human activities altering the landscape. Deforestation: Removal of forests for agriculture, reducing biodiversity. Desertification: The process of fertile land becoming desert due to various factors. New cards 15 How do water flow and light penetration structure life in aquatic systems? Define photic zone and benthic zone. Water Flow: Affects oxygen levels and nutrient distribution. Light Penetration: Determines photosynthesis depth. Photic Zone: The upper layer of water supporting photosynthesis. Benthic Zone: The bottom layer inhabited by decomposers. New cards 16 How do coastal substrates and plant life structure coastal communities? Define mangrove, salt marsh, and rocky intertidal zone. Coastal Substrate: The type of surface influencing organisms present. Mangroves: Tropical trees that grow in saline environments. Salt Marshes: Coastal wetlands dominated by grasses (herbaceous plants). Rocky Intertidal Zone: Area between high and low tide, home to organisms like barnacles. New cards 17 How do plants and animals cope with temperature extremes? Define avoidance and tolerance. Avoidance refers to strategies organisms use to prevent exposure to extreme environmental conditions. Tolerance is the ability of an organism to withstand extreme conditions by adapting physiologically or structurally. Avoidance Strategies ​ Behavioral Adaptations: Animals migrate, burrow, or seek shade during extreme heat or cold. ○​ Example: Desert lizards bask in the sun in the morning to warm up but hide in burrows during the midday heat. ​ Seasonal Avoidance: Some animals avoid extreme temperatures by hibernating (cold) or estivating (heat). ○​ Example: Bears hibernate in winter to avoid cold temperatures, reducing metabolism to conserve energy. ​ Deciduous Trees: Shed leaves to reduce water loss during extreme cold or dry seasons. ​ Tolerance Strategies ○​ Physiological Adaptations: Some organisms can survive extreme temperatures by adapting their internal processes. ○​ Example: Antarctic fish produce "antifreeze" proteins to prevent their blood from freezing. ○​ Heat-Shock Proteins: Produced by many organisms to protect cells from heat damage. ○​ Succulents: Tolerate heat by storing water and having thick, waxy surfaces to reduce evaporation. New cards 18 What is the difference between acclimation and adaptation? Acclimation: Temporary changes in an individual’s physiology. Adaptation: Long-term genetic changes through natural selection. New cards 19 What are thermal limitations to life, and why do they matter? Temperature plays a critical role in biological processes. Most organisms have a specific temperature range where they can survive, grow, and reproduce. When temperatures go beyond these limits, it can lead to: 1.​ Enzyme Denaturation (Heat) – Enzymes are proteins that control biological reactions. High temperatures can denature (unfold) them, rendering them non-functional. ​ Example: Enzymes in most organisms function optimally between 0°C and 40°C. Above this, enzyme activity declines. 2.​ Freezing of Water (Cold) – Water inside cells can freeze, forming ice crystals that rupture cell membranes and cause death. ​ Example: Few organisms survive below -20°C unless they have antifreeze compounds or desiccation strategies. 3.​ Metabolic Disruption – Extreme temperatures can slow down or speed up metabolism beyond what an organism can handle, leading to energy imbalances and death. ​ Example: Cold-blooded animals become sluggish in cold temperatures due to reduced metabolic activity. New cards 20 What mechanisms help plants deal with variable temperatures? Define stomata and evapotranspiration. Plant Mechanisms for Dealing with Variable Temperatures: Stomata & Evapotranspiration 1.​ Stomata ​ Definition: Stomata are small openings on the surfaces of leaves that regulate gas exchange and water loss. They are crucial in helping plants cope with temperature fluctuations. ​ Mechanism in Variable Temperatures: ○​ High Temperatures: Stomata open to release water vapor through transpiration, cooling the plant (similar to sweating in humans). ○​ Low Temperatures or Drought Conditions: Stomata close to reduce water loss and prevent dehydration, which can help the plant survive colder or drier environments. ​ Example: Desert plants (like cacti) keep stomata closed during the day and open them at night (CAM photosynthesis) to minimize water loss. 2.​ Evapotranspiration ​ Definition: Evapotranspiration is the process of water movement through a plant and its evaporation from aerial parts, primarily through the stomata. This process helps regulate plant temperature. ​ Cooling Effect: When water evaporates from leaf surfaces, it dissipates heat, keeping the plant cool in hot environments. This is essential for preventing heat stress and maintaining photosynthesis. ​ Influence on Water Loss: While evapotranspiration is beneficial for cooling, it increases water loss. Therefore, plants in dry or hot climates have evolved adaptations (e.g., smaller or fewer stomata, waxy cuticles) to balance cooling with water conservation. New cards 21 How do plants modify their boundary layers to deal with temperature changes? Define boundary layer. Boundary Layer Definition: ​ A thin layer of still air around the leaf surface that acts as an insulating buffer, slowing heat, water vapor, and gas exchange. How Plants Modify It: 1.​ Leaf Size & Shape: ​ Large leaves → Thick boundary layer (slows heat exchange) ​ Small/narrow leaves → Thin boundary layer (faster cooling) 2.​ Leaf Hairs (Trichomes): ​ Increase boundary layer thickness, reducing water loss and heat stress. 3.​ Leaf Orientation: ​ Adjust angle to minimize direct sunlight and control temperature. 4.​ Cuticle Thickness: ​ Thick cuticle increases boundary layer, conserving water and stabilizing temperature. New cards 22 What are the differences between ectotherms and endotherms? Define both terms. Ectotherms ​ Rely on external sources to regulate body temperature. ​ Body temperature fluctuates with the environment. ​ Lower metabolic rate than endotherms. ​ Examples: Reptiles, amphibians, fish. Endotherms ​ Generate heat internally to maintain a stable body temperature. ​ Can remain active in a wide range of temperatures. ​ Higher metabolic rate to support heat production. ​ Examples: Mammals, birds. Key Differences: 1.​ Temperature Regulation: ​ Ectotherms: Use behavioral strategies (e.g., basking, seeking shade). ​ Endotherms: Use metabolic heat production (e.g., shivering, sweating). 2.​ Energy Demand: ​ Endotherms require more energy (higher food intake) to maintain body temperature. ​ Ectotherms need less food because they don’t generate heat internally. New cards 23 What is Bergmann’s Rule, and how does it relate to body size and temperature? Bergmann’s Rule: ​ States that individuals of a species tend to have larger body sizes in colder climates and smaller body sizes in warmer climates. Relation to Body Size and Temperature: ​ Larger Body Size → Lower surface area-to-volume ratio, reducing heat loss and helping conserve body heat in cold environments. ​ Smaller Body Size → Higher surface area-to-volume ratio, increasing heat dissipation and helping stay cool in warm environments. Example: ​ Polar bears are large to retain heat, while desert foxes are small and slender to release heat efficiently. New cards 24 What is Allen’s Rule, and how does it affect body shape in different climates? Allen’s Rule: ​ States that animals in colder climates tend to have shorter limbs and appendages (ears, tails, legs) to conserve heat, while animals in warmer climates have longer limbs and appendages to dissipate heat. How It Affects Body Shape: ​ Colder Climates: Short, compact body shapes minimize heat loss. ○​ Example: Arctic foxes have short ears and tails to retain heat. ​ Warmer Climates: Long, slender body shapes promote heat dissipation. ○​ Example: Jackrabbits have long ears to release heat. New cards 25 What are some general adaptations that animals have for retaining heat or dealing with cold? ​ Insulation: Thick fur, feathers, or fat layers (blubber) reduce heat loss. ​ Behavioral Adaptations: Hibernation, burrowing, or huddling for warmth. ​ Physiological Adaptations: Shivering, vasoconstriction (reducing blood flow to extremities), or countercurrent heat exchange. New cards 26 How does water flow along gradients? Define osmotic, pressure, and gravitational gradients. Water moves from areas of higher potential to lower potential along these gradients: 1.​ Osmotic Gradient: Water moves toward areas with higher solute concentration. ​ Example: Water enters plant roots from soil due to higher solute concentration in root cells. 2.​ Pressure Gradient: Water flows from high-pressure areas to low-pressure areas. ​ Example: Xylem transports water upward due to pressure differences. 3.​ Gravity: Water naturally flows downward due to gravity unless overcome by capillary action or pressure. ​ Example: Water drains downward through the soil. New cards 27 What are some general adaptations that plants have to deal with water stress? 1. Morphological Adaptations: ​ Thick Cuticle: Reduces water loss by minimizing evaporation. ​ Small or Waxy Leaves: Less surface area reduces water loss. ​ Deep Roots: Access water deep underground. ​ Succulent Tissues: Store water for use during droughts (e.g., cacti). 2. Physiological Adaptations: ​ CAM Photosynthesis: Stomata open at night to reduce water loss. ​ Stomatal Control: Stomata close during heat or drought to conserve water. ​ Leaf Shedding: Drop leaves during dry seasons to minimize transpiration. 3. Behavioral Adaptations: ​ Dormancy: Reduce growth during drought periods. ​ Leaf Rolling: Reduces exposure to sunlight and water loss. New cards 28 What are some general adaptations that animals have to deal with water stress? 1. Behavioral Adaptations: ​ Nocturnal Activity: Avoid heat and conserve water by being active at night (e.g., desert foxes). ​ Burrowing: Stay in cool, humid environments underground during the day (e.g., kangaroo rats). ​ Migration: Move to areas with better water availability. 2. Morphological Adaptations: ​ Water-Storing Tissues: Some reptiles and amphibians can store water in their bladders. ​ Specialized Skin or Scales: Reduce water loss (e.g., thick skin in reptiles, waxy cuticles in insects). ​ Efficient Kidneys: Produce highly concentrated urine to conserve water (e.g., camels, desert rodents). 3. Physiological Adaptations: ​ Metabolic Water Production: Some animals obtain water from metabolizing food (e.g., seeds provide water for kangaroo rats). ​ Tolerance to Dehydration: Certain animals can lose a significant percentage of body water without harm (e.g., camels). New cards 29 How do organisms obtain energy? Define autotrophs and heterotrophs. 1. Autotrophs (Producers) ​ Definition: Organisms that produce their own food using energy from sunlight or inorganic chemicals. ​ Types: ○​ Photoautotrophs: Use sunlight for energy through photosynthesis. Example: Plants, algae, and cyanobacteria. ○​ Chemoautotrophs: Use energy from chemical reactions involving inorganic molecules (e.g., sulfur or iron compounds) through chemosynthesis. Example: Certain bacteria and archaea. ​ Importance: They are the foundation of most ecosystems, providing energy-rich compounds (like glucose) that sustain other organisms. 2. Heterotrophs (Consumers) ​ Definition: Organisms that cannot produce their own food and rely on other organisms (autotrophs or other heterotrophs) for energy. ​ Types: ○​ Herbivores: Eat only plants. Example: Deer, rabbits. ○​ Carnivores: Eat other animals. Example: Lions, hawks. ○​ Omnivores: Eat both plants and animals. Example: Humans, bears. ○​ Decomposers: Break down dead organic matter, recycling nutrients back into the ecosystem. Example: Fungi, bacteria. ​ Energy Source: They obtain energy through the consumption and digestion of organic matter New cards 30 What is the difference between chemosynthesis and photosynthesis? Define both terms. Photosynthesis: Converts sunlight, CO₂, and water into glucose. 6CO2​+6H2​O+light energy→C6​H12​O6​+6O2​ Chemosynthesis: Uses chemical reactions to produce energy. New cards 31 What are detritivores, parasites, and predators? Define each. ​ Detritivores: Organisms that feed on dead organic matter, recycling nutrients. ○​ Example: Earthworms, fungi, dung beetles. ​ Parasites: Organisms that live on or inside a host, benefiting while harming the host. ○​ Example: Tapeworms, lice, mistletoe. ​ Predators: Organisms that hunt, kill, and consume other organisms for energy. ○​ Example: Lions, hawks, sharks. New cards 32 How do light, CO₂ concentrations, and nutrient availability impact the rate and capacity of photosynthesis? ​ Light Intensity ○​ Effect: Increases photosynthetic rate until it reaches a saturation point. ○​ Why: Light powers the light-dependent reactions that produce ATP and NADPH for glucose synthesis. ​ CO₂ Concentration ○​ Effect: Higher CO₂ increases the rate until enzymes become the limiting factor. ○​ Why: CO₂ is fixed by Rubisco in the Calvin cycle to produce glucose. ​ Nutrient Availability ○​ Effect: Nutrient deficiencies reduce photosynthetic capacity. ○​ Why: Nutrients like nitrogen (for proteins and chlorophyll), phosphorus (for ATP), magnesium (for chlorophyll), and iron (for electron transport) are essential for photosynthesis. New cards 33 What are the basic differences between C3, C4, and CAM photosynthesis? 1.​ C3 Plants ​ Most common type. ​ Use the Calvin cycle directly. ​ Found in cool, moist environments (e.g., rice, wheat). 2.​ C4 Plants ​ Have a special process to reduce water loss and avoid wasteful photorespiration. ​ Found in hot, sunny environments (e.g., maize, sugarcane). 3.​ CAM Plants ​ Open stomata at night to conserve water. ​ Found in dry, arid environments (e.g., cacti, succulents, pineapple). New cards 34 What is the tradeoff between low-energy foods that are easy to obtain and high-energy foods that are harder to obtain? Low-Energy Foods: Easy to find, fewer calories. High-Energy Foods: Harder to obtain, more calories. Organisms balance energy spent obtaining food with energy gained. New cards 35 What are some adaptations animals have for finding food? Define mobility and mouth shape/size. 1.​ Mobility ​ Adaptation: Helps organisms reach different food sources. ​ Examples: ○​ Birds with strong wings migrate to find food. ○​ Fish with streamlined bodies swim quickly to catch prey. ○​ Predators like cheetahs rely on speed to hunt. 2.​ Mouth Shape/Size ​ Adaptation: Specialized for different diets and feeding strategies. ​ Examples: ○​ Birds: Sharp beaks for tearing meat (eagles), long beaks for nectar (hummingbirds). ○​ Fish: Wide mouths for gulping prey (groupers) or long, narrow mouths for picking food (pipefish). ○​ Herbivores: Flat teeth for grinding plants (cows, horses). New cards 36 What are some adaptations animals have for eating food? Define unique digestive systems, symbiosis, and tools. 1.​ Unique Digestive Systems ​ Specialized digestive systems help process specific types of food. ​ Examples: ○​ Ruminants (e.g., cows): Multi-chambered stomachs for digesting tough plant material (cellulose). ○​ Carnivores (e.g., wolves): Shorter digestive tracts for processing meat. ○​ Birds (e.g., chickens): Gizzard for grinding food. 2.​ Symbiosis ​ Organisms form partnerships to help digest food or obtain nutrients. ​ Examples: ○​ Termites: Host gut bacteria to digest wood. ○​ Coral: Use symbiotic algae for energy. ○​ Humans: Gut bacteria help break down complex carbohydrates. 3.​ Tools ​ Some animals use tools to access or prepare food. ​ Examples: ○​ Chimpanzees: Use sticks to extract termites. ○​ Sea otters: Use rocks to crack open shells. ○​ Birds (crows): Use sticks to retrieve insects. New cards 37 What is evolution? Evolution: The process by which populations change over generations due to changes in allele frequencies. New cards 38 What are allele frequencies, and how do you calculate them? Define allele and frequency. Allele: A gene version found at a chromosome location. Frequency: Proportion of an allele in a population. Calculated by number of copies of an allele divided by total alleles. New cards 39 What are the four main mechanisms of evolution? Define each. Mutation: Random changes in DNA. Genetic Drift: Random changes in small populations. Gene Flow: Allele exchange between populations. Natural Selection: Favoring individuals with beneficial traits. New cards 40 How can speciation arise? Define speciation and the types of reproductive barriers. Speciation: Formation of new species through reproductive isolation. Reproductive Barriers: Prezygotic (prevent fertilization) and Postzygotic (affect offspring viability). New cards 41 What are some limits to evolution? Define genetic variation, evolutionary constraint, and ecological trade-off. 1.​ Genetic Variation ​ Definition: The diversity of alleles and genes within a population. ​ Why it limits evolution: Without enough genetic variation, populations cannot adapt to changing environments, which reduces the potential for evolution. ​ Example: Cheetahs have very low genetic diversity, making them vulnerable to disease and environmental changes. 2.​ Evolutionary Constraint ​ Definition: Restrictions or limitations on the possible evolutionary outcomes due to genetic, developmental, or historical factors. ​ Why it limits evolution: Some traits can't evolve because of developmental constraints or lack of necessary genetic mutations. ​ Example: Vertebrates can't evolve wheels because of anatomical limitations. 3.​ Ecological Trade-off ​ Definition: Situations where gaining an advantage in one trait causes a disadvantage in another. ​ Why it limits evolution: Trade-offs prevent organisms from being "perfect" by forcing compromises. ​ Example: Fast-growing plants may reproduce quickly but are less resistant to drought compared to slow-growing species. New cards 42 What characteristics are included in life histories? Define life history. Life History Definition Life history is the study of the patterns and strategies organisms use for growth, development, reproduction, and survival Key Characteristics of Life Histories 1.​ Age at First Reproduction ​ When an organism begins reproducing. ​ Example: Some fish reproduce in their first year, while elephants may take 10–15 years. 2.​ Reproductive Frequency ​ How often an organism reproduces during its lifetime. ​ Semelparity (one-time reproduction): Salmon reproduce once and die. ​ Iteroparity (multiple reproductions): Humans and birds reproduce multiple times. 3.​ Number and Size of Offspring ​ Trade-off between producing many small offspring or fewer large offspring. ​ Example: Mice have many small offspring, while whales have one large calf at a time. 4.​ Parental Investment ​ The amount of care and resources parents provide to their offspring. ​ Example: Birds provide significant care, while sea turtles lay eggs and leave. 5.​ Lifespan and Mortality Rate ​ How long an organism typically lives and the likelihood of dying at each stage of life. ​ Example: Tortoises have long lifespans and low mortality after they reach adulthood. New cards 43 What is the concept of life cycles? oncept of Life Cycles A life cycle describes the series of stages an organism goes through from the beginning of its life until it reproduces and dies. Life cycles vary widely among species but always involve key processes like growth, development, reproduction, and death. Key Stages of a Life Cycle 1.​ Birth or Formation ​ The starting point of life (e.g., seeds for plants, eggs for animals, cell division for single-celled organisms). 2.​ Growth and Development ​ Organisms grow and develop into adults capable of reproduction. ​ Example: Tadpoles grow into frogs, caterpillars develop into butterflies. 3.​ Reproduction ​ The process of creating offspring to pass on genetic material. ​ Reproductive strategies: Some organisms reproduce once and die (e.g., salmon), while others reproduce multiple times (e.g., birds, humans). 4.​ Aging and Death ​ The natural process of aging leads to the end of an organism’s life. Types of Life Cycles 1.​ Simple Life Cycle (e.g., humans, birds): Direct growth from birth to adulthood without drastic changes. 2.​ Complex Life Cycle (e.g., frogs, butterflies): Includes multiple stages, such as larvae and metamorphosis. 3.​ Alternation of Generations (e.g., plants, some algae): Alternates between a multicellular haploid stage (gametophyte) and a multicellular diploid stage (sporophyte). New cards 44 What is phenology, and how does it relate to climate variation? Phenology Definition Phenology is the study of the timing of biological events in plants and animals, such as flowering, migration, and reproduction, in relation to seasonal and environmental changes. Examples of Phenological Events 1.​ Plants: Timing of budburst, flowering, and leaf fall. 2.​ Animals: Migration of birds, hibernation patterns, and breeding periods. 3.​ Insects: Emergence of pollinators like bees and butterflies. How Phenology Relates to Climate Variation Phenology is closely tied to climate variation because many biological events are triggered by environmental cues like temperature, precipitation, and daylight. Changes in climate can cause shifts in these events. 1.​ Earlier or Delayed Events ​ Example: Rising temperatures may cause flowers to bloom earlier in the spring or delay hibernation in animals. 2.​ Mismatch in Timing (Phenological Mismatch) ​ Example: If plants bloom earlier but pollinators don’t emerge in time, it can affect plant reproduction and disrupt ecosystems. 3.​ Changes in Migration and Breeding ​ Example: Birds may alter their migration schedules due to changing temperature patterns, affecting breeding success. 4.​ Impact on Agriculture ​ Changes in phenology affect crop planting and harvesting times, as well as pest outbreaks. New cards 45 What are the two main modes of reproduction, and how do they differ? Define asexual reproduction and sexual reproduction. 1.​ Asexual Reproduction – Offspring are clones of the parent, produced through binary fission (in prokaryotes and protists) or vegetative propagation (in plants). Some organisms, like corals, can reproduce both sexually and asexually. ​ Advantages: Rapid reproduction, no need for a mate. ​ Disadvantages: Lack of genetic diversity makes populations vulnerable to environmental changes. 2.​ Sexual Reproduction – Involves the combination of genetic material from two parents, leading to genetic diversity. ​ Isogamy: Gametes are equal in size. ​ Anisogamy: One gamete (usually the egg) is much larger and provides nutrients for the developing embryo, while the sperm is smaller and more mobile. ​ Advantages: Increases genetic variation, which helps populations adapt to environmental changes. ​ Disadvantages: Requires more time and energy, finding mates can be difficult, and only half of an individual's genes are passed to offspring. A major evolutionary advantage of sexual reproduction is genetic recombination, which reshuffles genes to create new combinations, enhancing adaptability. New cards 46 What are the costs and benefits of asexual vs. sexual reproduction? Asexual: Rapid, no mate needed (cost: low diversity). Sexual: Increases diversity (cost: requires time, energy). New cards 47 What is fitness, and how is it measured? Fitness: An organism's ability to survive and reproduce. Measured by offspring contribution to the next generation. New cards 48 What are trade-offs in life history strategies? Define reproductive trade-off. Trade-offs in Life History Str