Advanced Biochemistry Lecture Notes PDF
Document Details
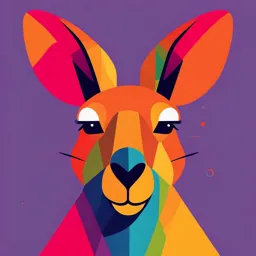
Uploaded by OpulentKrypton
University of Colombo
Dr. Duleepa Pathiraja
Tags
Summary
These lecture notes cover Advanced Biochemistry topics such as enzyme mechanisms and kinetics, microbial and plant metabolism, and photosynthesis. They are suitable for undergraduate or postgraduate Biochemistry students.
Full Transcript
C41052/CHE41052 Advanced Biochemistry Dr. Duleepa Pathiraja [email protected] 1 Course Overview (14h) 1. Mechanism of enzyme action and enzyme kinetics (6h) Introduction to enzyme catalyzed reactions Enzyme kinetics, Enzyme inhibition...
C41052/CHE41052 Advanced Biochemistry Dr. Duleepa Pathiraja [email protected] 1 Course Overview (14h) 1. Mechanism of enzyme action and enzyme kinetics (6h) Introduction to enzyme catalyzed reactions Enzyme kinetics, Enzyme inhibition Bimolecular mechanism of enzymatic reactions 2. Microbial and plant metabolism (4h) Microbial carbohydrate metabolic pathways Glyoxylate cycle acetone-butanol fermentation Distinguishing pathways of glucose utilization Metabolism of sulfur containing amino acids Synthesis of methionine and cysteine in plant and bacteria Shikimic acid pathway Microbial production of glutamate 3. Photosynthesis (4h) Light reactions Calvin cycle C3 and C4 plants 2 Organisms must replicate and catalyze chemical reactions to maintain life. Replication – Transfer of genetic material to the next generation DNA Replication Cell devision Metabolism – set of chemical reactions that sustain life Conversion of food in to energy Conversion of food into biological molecules (Carbohydrates, Proteins, lipids, Nucleaic acids) Excretion of wastes. Catalysis – Enzymes Biological reactions are catalyzed by enzymes 3 Introduction to enzyme catalyzed reactions Conversion of sucrose to CO2 and H2O in biological systems. Highly exergonic process that release energy for physiological functions. Sucrose is stable on shelf for many years. But readily broken down and consumed in living organisms. Hydrolysis of sucrose is catalyzed by enzymes. 4 Introduction to enzyme catalyzed reactions Enzymes are biological catalysts High degree of specificity for their substrates. Catalytic power is greater than that of inorganic or synthetic catalysts. Function in aqueous solutions under very mild conditions of pH and temperature. Catalyze biological reactions for breakdown nutrients (catabolism), synthesize macromolecules from their precursors (anabolism) etc. Deficiency or excessive activity can cause diseases. Enzyme activity can be used for diagnosis of certain diseases. Pharmaceuticals bind with enzymes and alter the enzyme activity. 5 Enzymes 1897 Eduard Buchner discovered that yeast extracts could ferment sugar to alcohol, proving that fermentation was promoted by molecules that continued to function when removed from cells. Frederick W. Kühne coined the term “enzymes”. In 1926, isolation and crystallization of urease by James Sumner. Urease crystals consisted entirely of protein, and he postulated that all enzymes are proteins. J. B. S. Haldane - Weak bonding interactions between an enzyme and its substrate might be used to catalyze a reaction. 6 Enzymes are proteins Most enzyme are proteins (except for catalytic RNA molecules, Ribozymes). But not all proteins are enzymes (structural, regulatory, transport etc.) Catalytic activity of a protein depends on its native structure. Catalytic activity is destroyed upon denaturation or dissociation of enzyme subunits. Heat Acids or alkali Organic solvants Chaotrophic agents 7 Enzymes are proteins 8 Enzyme classification Enzymes are biological catalysts that accelerate chemical reactions in living organisms. They are classified into six major classes based on the type of reaction they catalyze. 9 Enzyme classification Oxidoreductases (EC 1) Function: Catalyze oxidation-reduction (redox) reactions, where one molecule is oxidized (loses electrons) and another is reduced (gains electrons). Example Reactions: Transfer of electrons or hydrogen atoms between molecules. Common Enzymes: Dehydrogenases (e.g., alcohol dehydrogenase): Catalyze the removal of hydrogen atoms. Oxidases (e.g., cytochrome oxidase): Catalyze the transfer of oxygen. Example Reaction: AH2+B→A+BH2 (where A is oxidized, and B is reduced) 10 Enzyme classification Transferases (EC 2) Function: Transfer functional groups (e.g., methyl, phosphate, amino) from one molecule to another. Example Reactions: Transfer of a group like phosphate (kinases) or a sugar (glycosyltransferases) from a donor molecule to an acceptor. Common Enzymes: Kinases (e.g., hexokinase): Transfer phosphate groups. Transaminases (e.g., alanine transaminase): Transfer amino groups. Example Reaction: A−X+B→A+B−X (where X is transferred from A to B) 11 Enzyme classification Hydrolases (EC 3) Function: Catalyze the cleavage of chemical bonds through the addition of water (hydrolysis). Example Reactions: Breaking down large molecules like proteins, lipids, and nucleic acids. Common Enzymes: Proteases (e.g., trypsin): Break down proteins by hydrolyzing peptide bonds. Lipases (e.g., pancreatic lipase): Break down lipids. Nucleases (e.g., DNase): Break down nucleic acids. Example Reaction: A−B+H2O→A−OH+B−H 12 Enzyme classification Lyases (EC 4) Function: Catalyze the breaking of various chemical bonds by means other than hydrolysis and oxidation, often forming a new double bond or ring structure. Example Reactions: Addition or removal of groups (e.g., water, ammonia, carbon dioxide) to form double bonds. Common Enzymes: Decarboxylases (e.g., pyruvate decarboxylase): Remove carbon dioxide from molecules. Aldolases (e.g., aldolase in glycolysis): Cleave carbon-carbon bonds. Example Reaction: A→B+C (where a molecule is split without water) 13 Enzyme classification Isomerases (EC 5) Function: Catalyze the rearrangement of atoms within a molecule to form isomers (compounds with the same molecular formula but different structures). Example Reactions: Conversion of glucose-6-phosphate to fructose-6-phosphate in glycolysis. Common Enzymes: Racemases (e.g., alanine racemase): Catalyze the conversion between stereoisomers. Epimerases (e.g., galactose epimerase): Change the configuration of a single stereocenter. Example Reaction: A→B (where A and B are isomers) 14 Enzyme classification Ligases (EC 6) Function: Catalyze the joining of two molecules, usually coupled with the hydrolysis of a high-energy bond in molecules like ATP. Example Reactions: Joining of DNA fragments during replication or repair (DNA ligase). Common Enzymes: DNA Ligase: Joins DNA strands. Carboxylases (e.g., pyruvate carboxylase): Add carbon dioxide to form new carbon-carbon bonds. Example Reaction: A+B+ATP→A−B+ADP+Pi 15 Enzyme classification Each enzyme has a unique EC number, based on the type of reaction it catalyzes. The four digits of the EC number are structured as follows: 1.First digit: The main class (e.g., oxidoreductase = 1, transferase = 2). 2.Second digit: The subclass, specifying the type of reaction. 3.Third digit: The sub-subclass, detailing the specific group transferred or bond cleaved. 4.Fourth digit: The unique identifier of the enzyme in that sub-subclass. Enzyme – ATP glucose phosphotransferase/hexokinase EC 2.7.1.1. 2 – class - transferase 7 – subclass – phosphotransferase 1 - a phosphotransferase with a hydroxyl group as acceptor 1 - D-glucose as the phosphoryl group acceptor 16 Cofactor: Non protein chemical component required for the enzyme activity. Metal ion: inorganic ions, such as Fe2+ , Mg2+ , Mn2+ , Zn2+ Coenzyme: Complex organic or metalloorganic compounds required for the enzyme activity. 17 Prosthetic group: coenzyme or metal ion bound tightly (sometimes with a covalent bond) to the enzyme protein. Holoenzyme: Catalytically active enzyme together with its bound coenzyme and/or metal ions Apoenzyme: Protein part of the holoenzyme. 18 Enzymatic activity Under biologically relevant conditions, uncatalyzed reactions tend to be slow. Biological molecules are stable under physiological conditions (neutral-pH, mild temperature, aqueous environment). Chemical reactions that are unlikely under physiological conditions. Transient formation of unstable charged intermediates Collision of two or more molecules in the precise orientation 19 Enzymatic activity Enzymes provide a specific environment where a given reaction can occur. Enzyme-catalyzed reaction is that it takes place within the confines of a pocket on the enzyme called the active site. Surface of the active site is lined with amino acid residues. Substituent groups of these amino acid residues bind with the substrate and catalyze the chemical reaction. The molecule that is bound in the active site and acted upon by the enzyme is called the substrate. Active site can enclose the substrate and sequestering it from the solution. Binding of the substrate to the enzyme forms the enzyme-substrate complex. 20 Enzyme Catalyzed Reactions E – Enzyme S- Substrate P – Product ES – Enzyme substrate complex EP – Enzyme product complex The function of a catalyst is to increase the rate of a reaction. Catalysts do not affect reaction equilibria. 21 Enzyme Catalyzed Reactions Ground state – starting point for either forward or reverse reaction ΔGO, the standard free-energy change: under defined set of conditions temperature 298 K; partial pressure of each gas 1 atm, or 101.3 kPa; concentration of each solute 1 M). Biochemical systems commonly involve H+ concentrations far below 1 M biochemical standard free-energy change, ΔG’O, the standard free-energy change at pH 7.0; 22 Enzyme Catalyzed Reactions ΔG’O for the reaction is negative and the equilibrium favors P. A favorable equilibrium does not mean that the S → P conversion will occur at a detectable rate. There is an energy barrier between S and P: the energy required for alignment of reacting groups, formation of transient unstable charges, bond rearrangements, and other transformations required for the reaction to proceed in either direction. 23 Enzyme Catalyzed Reactions Transition state: Highest energy state at which decay to S or P state is equally probable. Transition state is not a chemical species with any significant stability. The difference between the energy levels of the ground state and the transition state is the activation energy, ΔG‡. The rate of a reaction reflects this activation energy: a higher activation energy corresponds to a slower reaction. 24 Enzyme Catalyzed Reactions Reaction rates can be increased by raising the temperature, thereby increasing the number of molecules with sufficient energy to overcome the energy barrier. Alternatively, the activation energy can be lowered by adding a catalyst. Catalysts enhance reaction rates by lowering activation energies. Enzymes are no exception. The role of enzymes is to accelerate the interconversion of S and P. The enzyme is not used up in the process, and the equilibrium point is unaffected. Reaction reaches equilibrium much faster when the appropriate enzyme is present, because the rate of the reaction is increased. 25 Enzyme Catalyzed Reactions Reaction intermediates. A reaction intermediate is any species on the reaction pathway that has a finite chemical lifetime (longer than a molecular vibration, ~1013 seconds). ES and EP complexes can be considered intermediates. ES and EP complexes occupy valleys in the reaction coordinate diagram. In an enzyme-catalyzed reaction, less stable chemical intermediates often exist. The interconversion of two sequential reaction intermediates constitutes a reaction step. 26 Enzyme Catalyzed Reactions Rate-limiting step. In a simple case, the rate-limiting step is the highest-energy point in the diagram for interconversion of S and P. When several steps occur in a reaction, the overall rate is determined by the step (or steps) with the highest activation energy – Rate limiting step. If there is no energy barrier? complex macromolecules would revert spontaneously to much simpler molecular forms. complex and highly ordered structures and metabolic processes of cells could not exist. 27 Enzyme Catalyzed Reactions Equilibrium constant – Keq For biochemical processes – K’eq 28 Rate of the reaction Concentration of the reactant(s) Rate constant (k) For the unimolecular reaction S → P, 𝑉 = 𝑘[𝑆], k – first order rate constant (s-1) If a reaction rate depends on the concentration of two different compounds, or if the reaction is between two molecules of the same compound 𝑉 = 𝑘[𝑆1][𝑆2], k – second order rate constant (M -1s-1) From the transition state theory: rate constant and activation energy → Eyring–Polanyi equation ‡ kB.T − ΔG k=.𝑒 𝑅𝑇 ℎ kB -Boltzmann constant h-Planck’s constant Activation energy is inverse and exponential. Lower activation energy, faster reaction rates 29 Mechanism of Enzyme catalyzed reaction Enzymes were structurally complementary to their substrates, so that they fit together like a lock and key Enzyme perfectly complementary to the substrate Substrate fits so tightly in the active site that it cannot reach the transition state. ES complex correspond to an energy trough 30 Mechanism of Enzyme catalyzed reaction To catalyze reactions, an enzyme must be complementary to the reaction transition state. The bound substrate must still undergo the increase in free energy needed to reach the transition state. Increase in free energy required for the conformational change is provided by the interactions (binding energy) between the enzyme and substrate in the transition state. 31 Mechanism of Enzyme catalyzed reaction Weak interactions are formed in the ES complex, but the full complement of such interactions between substrate and enzyme is formed only when the substrate reaches the transition state. The summation of the unfavorable (positive) activation energy ΔG‡ and the favorable (negative) binding energy ΔGB results in a lower net activation energy 32 Rate enhancements by enzymes Enzymes can make rate enhancements in the range of 5 to 17 orders of magnitude How? 1. Rearrangements of covalent bonds during an enzyme catalyzed reaction. Catalytic functional groups on an enzyme may form a transient covalent bond with a substrate and activate it for reaction. In many cases, these reactions occur only in the enzyme active site. Lower the activation energy (and thereby accelerate the reaction) by providing an alternative, lower-energy reaction path. 33 Rate enhancements by enzymes 2. Non- covalent interactions between enzyme and substrate. Noncovalent interactions between substrate and enzyme. Formation of ES complex. The interaction between substrate and enzyme in this complex is mediated by the same forces that stabilize protein structure, including hydrogen bonds and hydrophobic and ionic interactions Formation of each weak interaction in the ES complex is accompanied by release of a small amount of free energy that provides a degree of stability to the interaction. The energy derived from enzyme-substrate interaction is called binding energy, ΔGB. 34 Weak binding interactions between the enzyme and the substrate provide a substantial driving force for enzymatic catalysis. An enzyme must provide functional groups for ionic, hydrogen-bond, and other interactions, and also must precisely position these groups so that binding energy is optimized in the transition state. Adequate binding is accomplished most readily by positioning a substrate in a cavity (the active site) where it is effectively removed from water. This is one reason why enzymes and some coenzymes are large molecules. 35 Binding energy and reaction specificity Specificity: the ability to discriminate between a substrate and a competing molecule. Enzymes are highly specific to their substrate. Specificity is derived from the formation of many weak interactions between the enzyme and its specific substrate molecule. More than 80% of the enzymatic rate acceleration has been traced to enzyme- substrate interactions involving the phosphate group on carbon 3 of the substrate. 36 Physical and thermodynamic factors A reduction in entropy, in the form of decreased freedom of motion of two molecules in solution Binding energy holds the substrates in the proper orientation to react. Increase the number of productive collisions. The need for proper alignment of catalytic functional groups on the enzyme. Induced fit: enzyme itself usually undergoes a change in conformation when the substrate binds, induced by multiple weak interactions with the substrate. Induced fit serves to bring specific functional groups on the enzyme into the proper position to catalyze the reaction. The conformational change also permits formation of additional weak bonding interactions in the transition state. The solvation shell of hydrogen-bonded water that surrounds and helps to stabilize most biomolecules in aqueous solution. Formation of weak bonds between substrate and enzyme also results in desolvation of the substrate. Enzyme-substrate interactions replace most or all of the hydrogen bonds between the substrate and water. 37