Genetics: Cell Division and Genetic Material PDF
Document Details
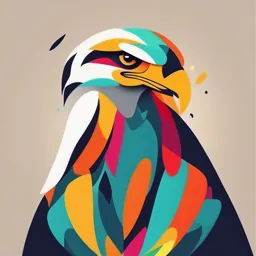
Uploaded by SecureClimax3805
Tags
Related
Summary
These notes cover cell division and genetic material, including mitosis, meiosis, DNA replication, chromosomes, and karyotypes. It also discusses sexual reproduction, gamete formation, and patterns of inheritance such as monohybrid and dihybrid crosses. The document concludes by examining complex inheritance patterns and sex-linked inheritance.
Full Transcript
Chapter 4.1: Cell Division and Genetic Material Genetics - the field of biology that studies how genetic information is passed from one generation of organisms/cells to the next The cell theory: - All living things are composed of one or more cells - The cell is the smallest unit of life -...
Chapter 4.1: Cell Division and Genetic Material Genetics - the field of biology that studies how genetic information is passed from one generation of organisms/cells to the next The cell theory: - All living things are composed of one or more cells - The cell is the smallest unit of life - New cells are created from pre-existing cells through cell division The cell cycle: Somatic cell - a plant/animal cell found in the body of an organism that is not used for reproduction All somatic cells go through the cell cycle. After they complete the cycle, they become two new cells. The cycle lasts around 12-24 hours. Why do somatic cells divide? - The growth of an organism - The reparation of tissues/organs - The replacement of dead cells Specific checkpoints in the cell cycle monitor growth to ensure the cycle continues/stops when it should. Regulation is key to prevent uncontrolled rapid growth (cancerous). Stages of the cell cycle: There are three main stages of the cell cycle: 1.Interphase - where the cell grows and intense activity occurs 2.Mitosis - where the cell’s nucleus and genetic material divide 3.Cytokinesis - where the division of the cell’s cytoplasm occurs and the creation of new cells exists Interphase: During interphase, the cell carries out its normal functions as it grows and makes copies of its genetic material. It is divided into three parts: 1.Growth 1 (G1) - the major period of growth 2.Synthesis (S) - when DNA (also called chromatin) is replicated 3.Growth 2 (G2) - involves further growth and the synthesis of molecules DNA replication: When DNA is replicated during interphase, the double helix unwinds and each strand of DNA serves as a template for a new strand. Each new double helix contains one original strand and one new strand. Mitosis: Before it divides, the cell must undergo mitosis, which is the separation of the cell’s replicated genetic material. Mitosis involves the following structures: Spindle fibers - microtubule structures that facilitate the movement of cells Centrosomes - structures that help to form spindle structures Cytokinesis: In animal cells, an indentation called a cleavage furrow forms in the cell membrane along the cell’s equator. It deepens as the cytoplasm divides equally and the cell pinches off into two cells. This is accompanied by conflicting microfilaments. In plant cells, the rigid square cell wall doesn’t pinch inward. Instead, a new structure called a cell plate forms between the daughter nuclei. Chromosomes: There is no relationship between the number of chromosomes an organism has in its cells and the complexity of the organism. For humans, there are two sets of 23 chromosomes in the somatic cells—one from the father and one from the mother. These sets are homologous, meaning they contain the same sequence of genes (traits), they are the same length, they have the same location of the centromere, and they have the same stain banding pattern. However, they contain different alleles (forms) of a gene. One of the pairs of human chromosomes (#23) are called sex chromosomes because they determine the sex of the individual. The two chromosomes (called x and y) are not homologous. XX = female and XY = male. The rest of the chromosomes (#1-22) are called autosomes. Each one has a true homologous pair. Karyotypes: Karyotype - a visual representation of a person’s complete set of chromosomes Chromosomes are collected and stained when a cell is in metaphase and so they appear as sister chromatids (X). Chromosome pairs are arranged based on length (longest to shortest) and the sex chromosomes are placed last in a karyotype. Chapter 4.2: Sexual Reproduction Sexual reproduction requires two parents and produces genetically distinct offspring that involves the fusion of a male cell with a female cell (called gametes). The cell that results from the fertilization is called a zygote. A zygote has the same number of chromosomes as a somatic cell. Each gamete only carries one set of homologous chromosomes. - Haploid (n) - a cell with one set of chromosomes - Diploid (2n) - a cell with two sets of chromosomes - The human diploid number is 2n = 46 Meiosis: Meiosis produces gametes with a haploid number of chromosomes. Genetic reproduction - produces daughter cells with half the number of chromosomes Genetic recombination - produces different combinations of alleles Meiosis has four phases: prophase, metaphase, anaphase, and telophase. It also involves two complete cycles of the four phases: meiosis I and meiosis II. Gamete formation in animals: Spermatogenesis - the process that produces sperm in male animals Oogenesis - the process that produces eggs in females In males, spermatogonia reproduce by mitosis then meiosis (starting at puberty). In females, oogonia reproduce by mitosis before birth. They begin meiosis but stop at prophase I. Each month after puberty, one cell completes meiosis, however the cytoplasm is unequally distributed (only one cell matures and not four). Multiple births: Scenario Outcome - More than one egg is - Fraternal released (non-identical) - A single zygote splits twins/siblings in the first days - Identical twins Genetic variation: During meiosis, genetic variation is ensured in two ways: - Independent assortment - Crossing over In independent assortment, gametes are created that carry different combinations of maternal and paternal chromosomes. This occurs during metaphase I when each homologous chromosome is randomly oriented towards one of the poles. This alone can produce over eight million different chromosome combinations. The number of genetically unique combinations can be calculated using 2^n where n is the haploid number for that organism. - 2^23 = 8388608 In a process called crossing over, genetic material between maternal and paternal chromosomes are exchanged. This occurs during prophase I in which sister chromatids exchange genetic material in multiple sections. Errors in chromosome structure: When chemical bonds are reformed during crossing over, errors can occur that can result in the following: Error Illustration Example disorder - The deletion - Cri-du-chat of a section syndrome of a chromosome - The - Charcot-Marie- duplication of Tooth disease a section of a chromosome - The inversion - FG syndrome of a section of a chromosome - The - Leukemia translocation of a section to a different chromosome Sometimes non-disjunction occurs where homologous chromosomes or sister chromatids don’t separate during meiosis (anaphase I or anaphase II). Non-disjunction produces gametes that have too many/too few chromosomes. Trisomy 21 (three copies of chromosome 21) is an example of non-disjunction. This results in down syndrome. Monosomy - a single chromosome copy On the left side, two gametes have one extra chromosome and two gametes have one fewer chromosome than normal. On the right side, two gametes have the usual number of chromosomes while one gamete has one extra and the other has one fewer. Prenatal genetic testing: These procedures are covered by the Ontario Health Insurance Plan. There are non-invasive tests where they test the mother’s blood for fetal proteins and sometimes use ultrasounds. And there are invasive tests such as amniocentesis and chorionic villus sampling. Chapter 4.3: Reproductive Strategies and Technologies Reproductive strategies and technologies used in agriculture: Selective breeding - the process of breeding plants and animals for desirable traits While sometimes imprecise, this strategy has produced many varieties of plants and animals. Artificial insemination - the transfer of (often processed) semen into a female’s reproductive tract Stock improves as breeders make high-quality sperm from choice males widely available. Embryo transfer - fertilizing an egg artificially and then transferring it into a recipient female Embryos can be shipped more easily than animals. Assistive reproductive technologies for humans: Artificial insemination - sperm is collected and concentrated then introduced into a woman’s vagina In vitro fertilization (IVF), immature eggs are retrieved, joined with sperm in the lab, and embryos are inserted into the woman’s uterus. This is an option for women with blocked fallopian tubes. Pre-implantation genetic diagnosis - as an additional step to IVF, one of the cells of an embryo is removed and tested for specific genetic disorders before it is implanted in the uterus Cloning: Gene cloning - manipulating DNA to produce multiple copies of a gene or another segment of DNA in foreign cells The production of the protein insulin is an example of this process, which is as follows: 1.Isolate the insulin gene segment. 2.Choose an appropriate vector, such as a bacterial plasmid. 3.Create recombinant DNA by inserting the insulin gene into the vector, using molecular agents to cut and join pieces together. 4.Treat the bacterial cells so that they take in the recombinant DNA in a process called transformation. The cells now make many copies of the gene and thus produce a large amount of the protein. 5.Harvest the insulin. Therapeutic cloning - producing genetically identical cells that are used to treat various diseases The cloned cells are then used to grow new tissues and organs. Reproductive cloning - the production of cell clones but with the aim of producing a genetically identical organism Reproductive cloning in animals is rarely successful. Both of the above methods use a process called somatic cell nuclear transfer (SCNT). In this technique, an egg cell’s nucleus is removed and replaced with the nucleus of a somatic cell of a donor. Controversy surrounds both therapeutic and reproductive cloning. Two issues arise with the process of therapeutic cloning (somatic cell nuclear transfer or SCNT) because a) the process produces stem cells that could possibly be used to create a human clone and b) the original cells can be/are used as embryonic stem cells. Scientists have found a solution to the second issue. They continue their research with all types of stem cells because of the potential impact on human health and transplant acceptance. Transgenic plant applications: Transgenic organism/genetically modified organism (GMO) - an organism that has had DNA from another species inserted into its genetic material An example of the application of this technique is to increase the nutritional value of plants such as golden rice. Transgenic animal applications: - Transgenic milk-producing animals can produce medical proteins like the human growth hormone. - Milk-producing animals can be modified to secrete silk for commercial use. - Transgenic animals could successfully serve as organ donors for humans. GMO concerns: Many people have concerns about GMOs despite thorough review processes. These concerns include: - The creation of super-weeds as species cross-reproduce, due to their herbicide genes. - Herbicide-resistant plants could encourage the use of stronger herbicides. - Not enough is known about the long-term effects of human - The amount of money spent may be greater than the overall benefit. Chapter 5.1: Patterns of Inheritance Understanding inheritance: - While people bred animals and plants for thousands of years without understanding the mechanisms of inheritance, eventually theories and explanations of how breeding worked were proposed. - The first widely accepted theory was pangenesis, proposed by Aristotle. It suggested that sperm and eggs contained tiny particles from all parts of the body. Others thought that it was only the sperm and that an entire mini human was inside of it! - By the 1800s, people settled on the idea that traits from the parents were irreversibly blended in their offspring. - None of these theories were based on scientific evidence. Gregor Mendel’s experiments: - Gregor Mendel (1822-1884), an Augustinian monk, used scientific methods to solve the mystery of how traits were inherited. Before his time at the monastery, he studied botany and mathematics, which proved invaluable to his observations. He chose to work with pea plants. Pea plants come in many varieties and show different traits. In addition, they usually self-fertilize, which allowed Mendel to start with plants that were true-breeding (same outcome traits every generation). He carefully cross-pollinated true-breeding pea plants. - Mendel started every experiment with plants that were true-breeding for a trait that exhibited a different form of the trait. He called this the parental or P generation. Offspring were called the first filial (F1) generation. These experiments were called monohybrid crosses because only one (mono) trait was monitored at a time. However, Mendel studied seven different traits in his experiments. - Example 1: P generation of male yellow-pea-producing plant and female green-pea-producing plant P generation cross results: All offspring (F1 generation) were the same seed colour: yellow, i.e., one parent’s seed colour trait seemed to disappear. This result was the same for each of the seven traits he studied. - Example 2: F1 generation of yellow-pea producing plants F1 generation cross results: In the F2 generation, some peas were yellow and some green. Mathematically, the ration was 3:1 yellow:green. This ration was the same for all seven traits that Mendel studied. The law of segregation: - Mendel concluded that there must be two hereditary “factors” for each trait. Today, we call those factors “alleles.” Recall that diploid organisms have two alleles for each gene. - He also concluded that one factor/allele is always dominant and one is recessive. In the example, yellow is dominant over green when it comes to the colour of seeds in the pea plant. - Mendel proposed the “law of segregation” to explain this: Traits are determined by the pairs of alleles that segregate during meiosis so that each gamete receives one allele. Genotype and phenotype: To express alleles easily in written form, upper and lower case letters are used. A dominant allele is represented by the first letter of the allele’s description. The recessive allele then receives the lower case of the same letter. i.e. Yellow pea allele: Y Green pea allele: y In each plant, two alleles are present so the possible combinations are: YY, Yy, or yy. This is the plant’s genotype. The actual colour of the peas is the plant’s phenotype. Chapter 5.2: Monohybrid Crosses The possibility of a certain allele packaged in a gamete is ½ since there are two alleles in a diploid cell and only one is packaged in a haploid gamete. Thus, when determining the possible outcomes of a monohybrid cross, there is ½ x ½ = ¼, or a 25% chance of each combination of alleles in the offspring. We use a grid called a Punnett square to show the law of segregation and possible cross outcomes. Hybrid crosses using Punnett Squares: Hybrids: - The result from crossing homozygous parents (monohybrid) - One parent is homozygous dominant - One parent is homozygous recessive - This test crosses results in a heterozygous F1 generation Example: Eye colour B = brown b = blue Genotype Phenotype Gametes Parent 1 BB brown eyes B or B Parent 2 bb blue eyes b or b F1 generation are all heterozygous (genotype) and all have brown eyes (phenotype). Crossing the F1 generation: Genotype Phenotype Gametes Parent 1 Bb brown B or b Parent 2 Bb brown B or b F2 generation: 3 are brown (75%) and 1 is blue (25%). This Punnett square demonstrates the possible outcomes from a cross between two parents tracing the inheritance of a single trait. This is known as a monohybrid cross. In this case, the parents are heterozygous for flower colour. The phenotype ratio is 3:1 (purple to white). Test cross - a cross between an individual of unknown genotype for a trait and an individual that is homozygous recessive for that trait Chapter 5.3: Dihybrid Crosses Mendel also designed experiments to follow the inheritance pattern of two traits to determine if the inheritance of one trait affected another, called a dihybrid cross. He crossed true-breeding plants that produced yellow, round seeds (YYRR) with true-breeding plants that produced green, wrinkled seeds (yyrr). The peas in the F1 generation all displayed the dominant trait for both traits (yellow and round). The F1 generation self-fertilized to create the F2 generation. It had a mix of four phenotypes but came close to the ratio 9:3:3:1 (yellow, round to yellow, wrinkled to green, round to green, wrinkled). Example: F1 generation: Parent 1: YyRr → Gametes: Yr, yR, YR, yr Parent 2: YyRr → Gametes: Yr, yR, YR, yr The gametes were determined by foiling. YR Yr yR yr YR YYRR YYRr YyRR YyRr Yr YYRr YYrr YyRr Yyrr yR YyRR YyRr yyRR yyRr yr YyRr Yyrr yyRr yyrr Phenotypic ratios: Yellow (Y_), round (R_) = 9 green (yy), round (R_) = 3 yellow (Y_), wrinkled (rr) = 3 green (yy), wrinkled (rr) = 1 Chapter 5.3: Following Patterns of Inheritance in Humans Geneticists collect inheritance information and use it to create a diagram called a pedigree. A pedigree is a flow chart that uses symbols to show the inheritance patterns of traits in a family over many generations. They help uncover the genotype of a particular member of a family, and they can be used to predict phenotypes and genotypes of future offspring. Autosomal inheritance is the inheritance of traits whose genes are found on the autosomes (chromosomes 1-22). These chromosomes hold normal, functioning genes (hair colour and freckles) as well as disorder genes (cystic fibrosis and Huntington's disease). An autosomal dominant disorder occurs when the disease-causing allele is dominant (A). An autosomal recessive disorder occurs when the disease-causing allele is recessive (a). When using a pedigree to study a disorder, you can determine if the pattern is autosomal dominant or autosomal recessive. Situation: Both parents are affected but the child is not. Inheritance pattern: Autosomal dominant (heterozygous parents) Situation: Two unaffected parents have a child with the disorder. Inheritance pattern: Autosomal recessive (heterozygous parents) A genetic counsellor has special training in human genetics and in counselling. A family may seek a counselor when there is a history of a genetic disorder in the family. Hints for analyzing pedigrees: 1) If the individual is homozygous recessive, then both parents MUST have at least one recessive allele (parents are heterozygous or homozygous recessive). 2) If an individual shows the dominant trait, then at least one of the parents MUST have the dominant phenotype. This one will be pretty obvious when you look at the pedigree. 3) If both parents are homozygous recessive, then ALL offspring will be homozygous recessive. Chapter 6.1: Complex Patterns of Inheritance As more statistical technologies became available, scientists realized that patterns of inheritance are more complicated than simple dominance and recessiveness. There are four complex patterns of inheritance: 1.Incomplete dominance 2.Codominance 3.Multiple alleles 4.Polygenic inheritance Incomplete dominance occurs when neither of the two alleles completely conceal the presence of the other allele. The heterozygote exhibits a phenotype somewhere between the two alleles. Example: Flowers P generation: True-breeding: white and red F1 generation: 100% of offspring appear pink F2 generation: 25% red, 50% pink, 25% white (1:2:1 ratio) Superscripts are used when the pattern of inheritance is incomplete dominance. An example of a human incomplete dominance disorder is familial hypercholesterolemia. Codominance occurs when both alleles are fully expressed. A roan animal is a visible example of this, such as a red and white roan bovine. Example: Sickle cell anemia (a human genetic disorder) Sickle cell anemia involves a misshapen red blood cell that cannot transport oxygen as efficiently as a normal red blood cell. Heterozygotes who carry the trait have some normal and some sickled red blood cells. Not only do heterozygotes who carry the trait not have sickle cell anemia, but the sickle cell allele also gives them an advantage in nature: they are resistant to malaria, a disease that affects red blood cells. Many traits in humans and other species are the result of the interaction of more than two alleles for one gene. The inheritance pattern is called multiple alleles. Example: Human blood type There are three possible alleles for the presence (or absence) of antigens on the surface of red blood cells: IA (codominant A antigen) IB (codominant B antigen) i (recessive; no antiagen) Again, superscripts are employed, as well as upper and lower case letters if there is a dominant and recessive allele present. Antigens - substances that cause the body to make an immune response against it Blood type AB - universal recipient Blood type O - universal donor Environmental effects on inheritance: In some cases, environmental factors such as temperature determine whether a gene is turned on or off. For example, the dark colour in Himalayan rabbits is due to a gene that is only active below a certain temperature. There are traits that exhibit continuous variation, where phenotypes vary gradually from one extreme to another. These include height and skin colour in humans, kernel colour in wheat, and ear length in corn. These are generally controlled by more than one gene and are called polygenic traits. Dominant alleles contribute to the phenotype while recessive alleles do not. Chapter 6.2: Inheritance of Linked Genes Alleles on the same chromosome don’t assort independently and don’t follow Mendel’s laws and patterns of inheritance. Some genes that are on the same chromosome are inherited together and are called linked genes. An example of this additional complex is found in sweet pea plants. All genes on any one chromosome are called a linkage group because they tend to be inherited together, unless crossing over interferes. Alleles for a pair of linked genes will separate less frequently if they are close to each other on the chromosome, and more frequently if they are far apart on the chromosome. Sex-linked inheritance: Thomas Hunt Morgan was a geneticist who worked with fruit flies (Drosophila melanogaster). When he crossed a male, white-eyes fly with a red-eyed female, the F1 generation showed a normal pattern of inheritance with all offspring having red eyes. When he crossed two flies from the F1 generation to create an F2 generation, he observed: - All the females had red eyes - Half the males had red eyes - Half the males had white eyes He concluded that the gene for eye colour was connected to gender and located on a sex chromosome (the X chromosome in this case). Traits that are controlled by genes on either the X or Y chromosome are called sex-linked traits. The X and Y human sex chromosomes have very little homologous DNA. The X chromosome has about 2000 genes while the Y has fewer than 100. If a disorder is X-linked dominant, affected males pass the allele to their daughters, who have a 100% chance of having the disorder. Females can pass a dominant allele to both sons and daughters, who would all have the disorder. However, most human sex-linked disorders are X-linked recessive. A son needs only one allele to be affected, while a daughter must inherit two recessive alleles to be affected. Individuals with red-green colour vision deficiency (CVD) have difficulty distinguishing between shades of red and green. CVD can be followed in a family by using a pedigree. A superscript is placed on an X or Y when the trait is sex-linked. In this case, CVD is an X-linked recessive disorder.