Lecture 9: Reproduction - male embryonic and postnatal PDF
Document Details
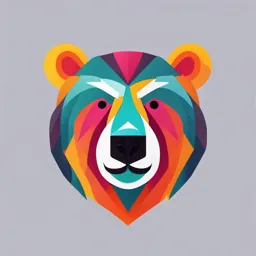
Uploaded by VeritableAmber980
Monash University
Tags
Related
- Lecture 9: Reproduction - Male Embryonic and Postnatal PDF
- Second Week Embryonic Development PDF
- A&P Reproduction, Fetal Development, and Heredity (Abridged) PDF
- Fertilization to Implantation Booklet 2 PDF
- Human Sexual Development and Reproduction Summary
- Human Sexual Development & Reproduction Summary
Summary
This document is a lecture on reproduction, focusing on male embryonic and postnatal development. It details the origin and development of the germline, including germline stem cells and their function. The lecture covers key concepts and learning objectives.
Full Transcript
🥽 Lecture 9: Reproduction - male embryonic and postnatal Part 1: Origin and development of the germline Context - reproductive/germline stem cells (GSCs) Germline stem cells produce either sperm or oocyte and are unipotent...
🥽 Lecture 9: Reproduction - male embryonic and postnatal Part 1: Origin and development of the germline Context - reproductive/germline stem cells (GSCs) Germline stem cells produce either sperm or oocyte and are unipotent GSCs are specialised stem cells responsible for producing gametes - sperm in males and oocytes (eggs) in females. Unlike multipotent stem cells, which can differentiate into multiple cell types, GSCs are unipotent, meaning they can only produce one type of cell—germ cells (sperm or oocytes). Stem cells from hematopoietic system, intestine, epidermis are multipotent This means they can differentiate into multiple cell types within their respective tissues. Eg), hematopoietic stem cells can give rise to various blood cells, including red blood cells, white blood cells, and platelets. Lecture 9: Reproduction - male embryonic and postnatal 1 “Terminally differentiated” germ cells generate the totipotent zygote – the foundation of the next generation After GSCs differentiate into mature germ cells (sperm or oocytes), these cells are considered “terminally differentiated” → ie. they have reached their final stage of development Terminally differentiated cell - one that, in the course of acquiring specialized functions, has irreversibly lost its ability to proliferate Genomic quality of differentiated germ cells is essential The genetic integrity of germ cells is crucial because they carry the DNA that will be passed on to the next generation. Any genetic defects in these cells could be inherited by the offspring, potentially leading to developmental abnormalities or genetic disorders. Terminally-differentiated cells of other high-turnover tissues are often short-lived genomic quality not as critical In tissues with high cell turnover, such as the skin, blood, and intestine, the terminally differentiated cells (e.g., skin cells, blood cells) are typically short-lived and regularly replaced by new cells. Because these cells do not contribute to the genetic makeup of the next generation, the emphasis on maintaining their genomic integrity is less critical compared to germ cells. However, defects in these cells can lead to diseases like cancer, but they won't be passed on to offspring. Epigenetic state of differentiated germ cells must be permissive for rapid reprogramming to a pluripotent state (from totipotent) After fertilisation, the epigenetic marks on the sperm and oocyte are rapidly reprogrammed to allow the zygote to become pluripotent, meaning it can differentiate into any cell type in the body. For this reprogramming to occur efficiently, the epigenetic state of the germ cells must be “permissive,” or conducive to the changes needed to reset the genome to a pluripotent state. This reprogramming is crucial for the early stages of embryonic development, enabling the formation of all the diverse tissues and organs in the body. LO: Understand the life cycle of a germ cell from specification onwards Lecture 9: Reproduction - male embryonic and postnatal 2 This leanring objective requires grasping the entire developmental process of germ cells after they have been specified as germ cells. Key Points to Include: Germ cell specification Migration to gonads Proliferation and meiosis Maturation of sperm/oocytes Epigenetic reprogramming ^^ These will be covered by Part 1 of the lecture LO: Define the origin of germ cells Germ line - the germ cell lineage that separates from the somatic cells (the rest of the body’s cells) Two types of germ cell specification: (1) Determinative (inherited factors); insects, worms, frog, fish (2) Regulative (induced by signaling factors); mammal Determinative germline Mechanism: occurs through the uneven distribution of inherited germplasm (cytoplasm containing specific mRNAs, proteins, and inhibitors) into daughter cells during early embryonic developmen Example: the early frog embyo has an “animal pole” (less yolk in cells) and “vegetal pole” (more yolk). The germ plasm is at the vegetal pole. Cells that inherit this germplasm are predetermined to become germ cells The germplasm contains molecular signals like Nanos, which mark cells destined to become part of the germline Lecture 9: Reproduction - male embryonic and postnatal 3 Regulative germline Mechanism: Unlike determinative specification, regulative germline specification involves the induction of germline fate by signals sent from neighboring cells. Examples: This process is observed in mammals, including humans and mice. Initially, cells within the early embryo (specifically the proximal posterior epiblast cells) are no different from other cells. These cells are induced to become germ cells through signals from surrounding tissues. The epiblast cells that receive these signals commit to the germline, migrate to the genital ridge, and ultimately give rise to germ cells (sperm or oocytes). The mammalian germ line is induced Germline Induction: Lecture 9: Reproduction - male embryonic and postnatal 4 In mammals, germline cells (sperm and oocytes) are not predetermined by cytoplasmic determinants, as seen in some other organisms, but are induced through signals from extraembryonic tissues. BMP (bone morphogenetic protein) from extraembryonic ectoderm, specifically BMP4 and BMP8B, act on the visceral endoderm (a layer of cells that lines the yolk sac) to initiate changes that ultimately lead to the formation of germ cells. BMP antagonists restrict BMP signaling to proximal epiblast → ensuring that the germline precursors are localized to the correct area Summary of the process of germline induction: BMP Signaling (eg. BMP4 and BMP8) : Starts from the extraembryonic tissues and influences the proximal epiblast to specify germline cells. Gene activation & repression: Blimp1 (also known as PRDM1): crucial transcription factor that plays a role in repressing somatic cell programs in the germline. Its activation ensures that cells destined to become germ cells do not differentiate into somatic (non-germline) cell types. PRDM14: Another important transcription factor involved in germline specification. It works alongside Blimp1 to promote the germline identity. Lecture 9: Reproduction - male embryonic and postnatal 5 Activation of pluripotency “germ cell” genes Oct4: A key factor in maintaining pluripotency, meaning the ability of cells to differentiate into various cell types. It remains switched on in germ cells to sustain their potential to become different cell types. SOX2: Part of the pluripotency network, it works with Oct4 and other factors to maintain the undifferentiated state of cells. Nanog: Another important pluripotency factor, it helps maintain the germline cells in an undifferentiated state. Nanos3: Involved in germline development and is also part of the pluripotency network. ^^ These molecular events give rise to primordial germ cells (PGCs) → PGCs migrate into the early forming gonads (future ovaries and testes) PGCs migrate to the gonads PGCs specified in express posterior proximal epiblast (PPE) → express BLIMP, PRDM14 → Become transcriptionally inactive (chromatin changes ) → Migrate via hindgut to gonads Lecture 9: Reproduction - male embryonic and postnatal 6 Molecular events marking early germline development Lecture 9: Reproduction - male embryonic and postnatal 7 During germline development, a series of molecular markers and transcription factors (like Blimp1, PRDM14, SOX2, Stella, Nanos3, and Nanog) are sequentially activated to establish and maintain the germline identity Concurrently, significant epigenetic reprogramming occurs, including reductions in global DNA methylation and changes in histone modifications, which are essential for resetting the epigenetic landscape of the germline cells and ensuring proper gene expression for their future development into sperm or oocytes. Life cycle of a germ cell 1. Early Germ Cell Development: Migration and Specification: Germ cells migrate to the genital ridge (genital ridge) after their initial specification and commitment. Pro-Repotency Genes Up-Regulated: These include genes like Blimp1, PRDM14, and others that support the germ cell identity. Lecture 9: Reproduction - male embryonic and postnatal 8 Somatic Genes Down-Regulated: This helps to ensure that germ cells maintain their distinct identity and do not adopt somatic cell functions. Global DNA Methylation Changes: Global demethylation (reduction of 5-methylcytosine) facilitates the erasure of epigenetic imprints from the somatic state and prepares the cells for reprogramming into either male or female epigenetic patterns. Epigenetic Reprogramming: Male vs. Female Epigenetic Patterns: Germ cells are epigenetically programmed differently depending on their eventual sex. This reprogramming is essential for establishing the proper epigenetic environment for either spermatogenesis (in males) or oogenesis (in females). 2. Puberty and Meiosis: For Females: Oogenesis: Female germ cells (oogonia) enter meiosis during embryogenesis but pause at prophase I. Extended Meiosis: They resume meiosis at puberty and complete the process only upon fertilization. This means that oocytes are arrested at various stages of meiosis until they are fertilized. For Males: Spermatogenesis: Male germ cells begin meiosis at puberty and continue to produce sperm throughout life. Continuous Production: Unlike females, male germ cells continuously produce sperm through a process that begins at puberty and lasts throughout the individual's life. Lecture 9: Reproduction - male embryonic and postnatal 9 LO: Understand how male and female germ cells differ Bipotential Nature of Primordial Germ Cells: Shared Origin: Primordial germ cells (PGCs) start as a bipotential cell type, meaning they have the potential to become either sperm or eggs depending on the signals they receive. Expression of Pluripotency Markers: Throughout their early development, PGCs express pluripotency markers like Oct4n Nagog, Sox2, indicating their ability to differentiate into various cell types. This pluripotency is maintained even after the cells migrate to the gonads. Lecture 9: Reproduction - male embryonic and postnatal 10 Migration and Gonadal Colonization: Migration: PGCs migrate to the gonadal ridge, where they will eventually differentiate into sperm or eggs. During migration, they continue to express pluripotency markers. In the Gonads: Male Gonads: In the testis, PGCs enter a mitotic arrest and later differentiate into spermatogonia. The process of spermatogenesis begins at puberty. Female Gonads: In the ovary, PGCs enter meiosis and arrest at prophase I. They resume meiosis only during puberty and complete it upon fertilization. Lecture 9: Reproduction - male embryonic and postnatal 11 Primordial germ cells undergo sex specific germline development once they enter the developing testis and ovary Male Gonad Development: SRY Gene Activation: The presence of the Y chromosome activates the SRY gene. SOX9 and FGF9: SRY induces SOX9, which then promotes FGF9 expression. This signaling cascade drives the differentiation of PGCs into male germ cells and leads to the formation of seminiferous cords in the testis. Outcome: This results in spermatogenesis and the development of male reproductive structures. Female Gonad Development: Ovarian Signaling: In the absence of the SRY gene, ovarian development is driven by different factors. FoxL2, Beta-Catenin, and Wnt: These factors drive the expression of retinoic acid and STRI8. Retinoic Acid and STRI8: In the ovary, retinoic acid and STRI8 initiate meiosis and promote female germ cell differentiation. Outcome: This process results in the formation of oocytes and the development of the ovarian follicles. Lecture 9: Reproduction - male embryonic and postnatal 12 Similar Signaling Pathways, Different Timing: Shared Pathways: Both males and females utilize similar signaling pathways, such as those involving retinoic acid and STRI8, but these are activated at different stages of development. In Males: Retinoic acid and STRI8 are involved in meiotic progression starting at puberty. In Females: These factors drive meiosis from early embryonic stages. Germ cells in the developing testis Indifferent Gonad: Similar to the ovary, the early testis starts as an indifferent gonad with bi-potential germ cells. Formation of Seminiferous Cords: As the testis develops, it forms seminiferous cords. These structures are early forms of tubules and are characterized by the organization of germ cells (gonocytes) in their center. Lecture 9: Reproduction - male embryonic and postnatal 13 The gonocytes are the precursor cells within these seminiferous cords. They are surrounded by Sertoli cells, which are essential for nurturing and supporting the developing germ cells. Seminiferous Tubules: Later in development, the seminiferous cords evolve into seminiferous tubules, which develop a lumen (central cavity). These tubules are where spermatogenesis occurs, eventually leading to the production of sperm. LO: Understand the timing of meiosis in males vs females, and the role of retinoic acid vs Fgf9 FGF9 and Retinoic Acid provide antagonistic signals that regulate male and female germline development Ovary: Retinoic acid (RA) increase Stra8 expression ⇒ induces meiotic arrest Testis: Fibroblast growth factor- 9 (Fgf9) decrease Stra8 expression ⇒ promotes mitotic arrest (arrest in G0) Fgf9 is required for male germ cell survival in mice Lecture 9: Reproduction - male embryonic and postnatal 14 Production and Role: FGF9 is produced by Sertoli cells in the testis. Its production is stimulated by the expression of SRY and SOX9 genes, which are critical for male gonadal development. Effects on Germ Cells: FGF9 plays a crucial role in promoting male fate. It prevents the germ cells from undergoing meiosis and helps maintain their pluripotent state, which is essential for their development into sperm later in life. Inhibition of Meiosis: FGF9 opposes retinoic acid, a signaling molecule that promotes meiosis. By inhibiting retinoic acid's action, FGF9 ensures that germ cells in the male gonad do not enter meiosis prematurely. FGF9 Knockout Studies: Lecture 9: Reproduction - male embryonic and postnatal 15 Testis: In the absence of FGF9 (e.g., FGF9 knockout models), the testis shows severe deficiencies. The Sertoli cells are present, but the germ cells are non-viable and die. Ovary: In contrast, the absence of FGF9 does not significantly affect the ovary. Germ cells in the ovary can survive and continue their development even without FGF9, which aligns with the fact that FGF9 is more critical for male germ cell development. Role of Fgf9 in male fate Inhibits Meiosis: By opposing retinoic acid, FGF9 prevents premature meiosis in male germ cells. Lecture 9: Reproduction - male embryonic and postnatal 16 Maintains Pluripotency: FGF9 helps maintain the expression of pluripotency markers, ensuring that germ cells remain in a state conducive to future sperm production. Promotes Male Fate: FGF9 reinforces male developmental pathways, ensuring that the germ cells in the testis develop according to male- specific patterns. Retinoic Acid: Function in Female Germ Cells: In contrast to FGF9, retinoic acid promotes meiosis and is critical for the development of female germ cells. It helps initiate meiosis in female germ cells, leading to the formation of oocytes. Retinoic acid also provides signals that regulate the post-natal male germline Postnatal Development: Increased Retinoic Acid: As the male mouse approaches puberty, retinoic acid levels increase. This rise in retinoic acid helps to initiate the early stages of meiosis in the germ cells, which is a critical step towards sperm production. Peak STRA8 Expression: STRA8 is expressed in male germ cells shortly after birth, peaking around 10 days postpartum. This timing is important because it marks the beginning of the transition towards meiosis, preparing the germ cells for eventual spermatogenesis. Without STRA8, the germ cells cannot progress properly into meiosis, which is necessary for fertility. Regulation: CYP26B1 helps fine-tune the levels of retinoic acid to maintain the appropriate developmental signals for either male or female germ cell development. LO: Understand epigenetic reprogramming in early development EG cells = Embryonic Germ cells derived from primordial germ cells of embryonic gonads (before E15.5 in mouse) Lecture 9: Reproduction - male embryonic and postnatal 17 NOT something that occurs in vivo → they are what we call a pluripotent stem cell, a germline pluripotent stem cell that we've taken out of the body and put into culture Have properties of stem cells when cultured with specific factors Express pluripotency markers (e.g., Oct4) Germ cell development and epigenetic reprogramming Overview of Epigenetic Reprogramming: Definition: Epigenetic reprogramming involves the erasure and remodeling of epigenetic marks, such as DNA methylation and histone modifications, which regulate gene expression. Purpose: This process ensures that the new organism starts with a clean slate of epigenetic information, allowing for the development of a new individual with appropriate epigenetic programming for pluripotency and differentiation. Phases of Epigenetic Reprogramming: (1) Pre-Implantation Embryo: Epigenetic Reprogramming in Early Development: After fertilization, the embryo undergoes significant global changes in DNA methylation. Initially, DNA methylation levels drop globally, and then remethylation occurs as development progresses through various stages, from the blastocyst to the implantation phase. Methylation Dynamics: In the early stages of the embryo, methylation levels are low. As the embryo develops, these levels increase, facilitating the differentiation of somatic tissues. (2) Gametogenesis: Erasure and Reprogramming: During gametogenesis (the formation of sperm and eggs), almost all of the DNA methylation patterns inherited from the parents are erased. This ensures that the genetic information passed to the offspring is reset and not biased by parental epigenetic marks. Differentiation in Males and Females: After the initial erasure, the patterns of DNA methylation and histone modifications are Lecture 9: Reproduction - male embryonic and postnatal 18 differentially remodeled in male and female germ cells. In Males: Methylation patterns are gradually reestablished, reaching their peak around birth. In Females: Methylation remains relatively low until much later in development, with full remethylation occurring closer to ovulation. Significance of Epigenetic Reprogramming: Pluripotency and Differentiation: Reprogramming is crucial for establishing a pluripotent state in the zygote, allowing for subsequent differentiation into various cell types and tissues. Germline Specificity: The distinct timing and patterns of remethylation in male and female germ cells ensure that each sex-specific developmental program is properly initiated. Part 2: Reproductive stem cells - male LO: Understand the stages of spermatogenesis Spermatogenesis - the process of producing male gametes (sperm) The five stages of spermatogenesis: (1) spermatogonia, (2) primary spermatocytes, (3) secondary spermatocytes, (4) spermatids, and (5) spermatozoa Key Stages of Spermatogenesis 1. Spermatogonial Stem Cells: Lecture 9: Reproduction - male embryonic and postnatal 19 Location: These cells are located at the basement membrane of the seminiferous tubules. Function: They undergo self-renewal to maintain a pool of stem cells. Some spermatogonia differentiate into primary spermatocytes, while others remain as stem cells. 2. Spermatogonial Phase: Process: Spermatogonia, which are diploid cells (having two sets of chromosomes), divide by mitosis to produce more spermatogonia or differentiate into primary spermatocytes. Outcome: This phase establishes the initial population of cells that will undergo meiosis. 3. Primary Spermatocyte: Process: Primary spermatocytes are diploid cells that enter meiosis I. Outcome: During meiosis I, primary spermatocytes divide to form two secondary spermatocytes, each of which has half the chromosome number (haploid). 4. Secondary Spermatocyte: Process: Secondary spermatocytes undergo meiosis II, a mitotic division, leading to the formation of spermatids. Outcome: This phase results in haploid cells (spermatids) from the secondary spermatocytes. 5. Spermatid Phase (Spermiogenesis): Process: Spermatids undergo transformation into spermatozoa (mature sperm). This process includes: Acrosome Formation: Development of a cap-like structure that contains enzymes to help penetrate the egg. Flagellum Formation: Formation of a tail that provides motility. Nuclear Compaction: Condensation of the DNA in the nucleus for a streamlined structure. Outcome: Mature, motile sperm cells with a head (containing the genetic material), mid-piece (containing mitochondria for energy), Lecture 9: Reproduction - male embryonic and postnatal 20 and tail. 6. Spermatozoa Maturation and Release: Process: Mature sperm are released into the lumen of the seminiferous tubules and then transported to the epididymis for further maturation. Outcome: Sperm acquire full motility and the capability to fertilize an egg Stages Across the Lifespan Embryonic Stage → Spermatogonial Stem Cell Renewal: In the embryo, spermatogonial stem cells are primarily undergoing self- renewal to establish the future stem cell pool. Childhood → Maintenance of Spermatogonial Population: During childhood, spermatogonia are present and proliferate, but meiosis and spermatogenesis are not actively occurring. Puberty Onset → Activation of Spermatogenesis: At puberty, spermatogonial stem cells begin the process of differentiation. One daughter cell from each mitotic division maintains the stem cell population, while the other progresses through meiosis and spermiogenesis. Adulthood → Continuous Sperm Production: Throughout adulthood, spermatogenesis is a continuous process, with millions of sperm being produced daily. Lecture 9: Reproduction - male embryonic and postnatal 21 LO: Understand the origin and definition of spermatogonial stem cells (SSC) Definition: spermatogonial stem cells (SSCs) are the most primitive spermatogonia in the testis and have an essential role to maintain highly productive spermatogenesis by self-renewal and continuous generation of daughter spermatogonia that differentiate into spermatozoa Origin of spermatogonial stem cells (1) Primordial Germ Cells (PGCs) and Gonocytes: In the embryonic testes, primordial germ cells (PGCs) migrate to and settle in the interior of the seminiferous cords, where they become known as gonocyte Gonocytes are the precursors to spermatogonia and will eventually give rise to sperm cells (2) Proliferation and Mitotic Arrest: Up to embryonic day 16.5 in mice, gonocytes proliferate as mitotic (M)-prospermatogonia. After this period, they enter a state of mitotic arrest and are referred to as T1-prospermatogonia. During this arrest, cell division ceases, and these cells remain in a quiescent state until after birth. Lecture 9: Reproduction - male embryonic and postnatal 22 (3) Postnatal Development: Following birth, the T1-prospermatogonia resume proliferation and migrate to the basement membrane region of the seminiferous tubule Once in the basement membrane region, they differentiate into T2- prospermatogonia. (4) Formation of Spermatogonial Stem Cells: T2 prospermatogonia give rise to As spermatogonia (stem cell population) Spermatogonial Stages and Differentiation 1. Spermatogonial Stem Cells: Type A Spermatogonia: These are the stem cells located at the periphery of the seminiferous tubules. They have the potential to produce sperm throughout an animal's life. Proliferation: In mice, these cells are highly prolific, producing around 10^9 sperm per day during the animal’s reproductive life. 2. Stages of Differentiation: Undifferentiated Stages: Includes A single, A paired, A aligned (4, 8, 16), and A1 spermatogonia. These stages represent various levels of spermatogonial differentiation. Differentiated Stages: Progresses through A2, A3, A4, and B spermatogonia. These stages then enter meiosis, progressing through primary and secondary spermatocytes to become spermatids. 3. Hierarchy of Differentiation: The spermatogonial stem cells go through a complex series of stages and divisions. This hierarchy is necessary to produce the mature sperm cells, with each stage contributing to the development of the next. Lecture 9: Reproduction - male embryonic and postnatal 23 Markers of spermatogonial stemcells As spermatogonia differentiate, there is a shift in marker expression. GFRα1 and PLZF are associated with earlier stages of spermatogonia while NGN9 and C-kit are markers of later stages, helping to track the differentiation process LO: Understand the progressive differentiation of spermatogonia Lecture 9: Reproduction - male embryonic and postnatal 24 Type A spermatogonia - stem cells within the seminiferous tubules that have the ability to self-renew and produce more spermatogonia or differentiate into other types of spermatogonia Type A spermatogonia can give rise to two types of daughter cells: Type A Spermatogonia: Continue to act as stem cells, maintaining the stem cell population. Type B Spermatogonia: These are derived from Type A spermatogonia and will eventually differentiate into primary spermatocytes. Primary Spermatocytes: They undergo meiosis to form secondary spermatocytes and eventually spermatids, leading to the formation of mature sperm. Blood-Testis Barrier 1. Protection from the Immune System: Sertoli Cells: These cells are crucial in forming the blood-testis barrier, which is essential for protecting developing germ cells from the immune system. Barrier Function: Sertoli cells create a physical barrier between the blood supply and the developing germ cells. This barrier prevents immune cells from accessing the germ cells. 2. Importance of the Barrier: Immune Tolerance: During embryogenesis, spermatogonial stem cells are recognized as "self" by the immune system. However, during puberty, new cell types (e.g., post-meiotic germ cells) are produced. These cells can be recognized as foreign by the immune system if not properly protected. Consequences of Immune Attack: If the immune system were to detect these developing germ cells as foreign, it would mount an immune response against them, leading to their destruction and resulting in infertility. Lecture 9: Reproduction - male embryonic and postnatal 25 After birth, the spermatogonial stem cells (SSCs) play a critical role in establishing and maintaining fertility SSCs / As spermatogonia either → 1) self renew & produce additional SSCs to maintain the stem cell population or 2) Producing differentiated spermatogonia that initiate spermatogenesis but do not self-renew. Proliferation phase: 6 days in mouse; several months in primates (incl. human) The proliferation phase in spermatogenesis refers to a period during which spermatogonial stem cells (SSCs) and their immediate progeny undergo rapid cell division. This phase is critical for establishing and maintaining the stem cell pool and for initiating the process of spermatogenesis. Potential outcomes of SSC divisions: Lecture 9: Reproduction - male embryonic and postnatal 26 Symmetric Self-Renewal: SSCs divide to produce two SSCs, preserving the stem cell pool. Symmetric Differentiation: SSCs divide to produce two differentiated spermatogonia, depleting the stem cell pool. Asymmetric Division: SSCs divide to produce one SSC (self-renewal) and one differentiated spermatogonia (which will progress through spermatogenesis). OVERALL - As spermatogonia cells / SSCs have TWO fates: Self-renewal OR spermatogenesis STUDY - AIM: to demonstrate that spermatogonial stem cells have the capacity to self-renew and reestablish spermatogenesis even in a testis that is depleted of germ cells Background: male fertility relies on continuity of spermatogenesis throughout life, provided by self-renewal & differentiation of Spermatogonial Stem Cells (SSCs) Methodology: LacZ+ Gene Labeling: Researchers utilized SSCs that have been genetically modified to contain the LacZ+ gene. This gene codes for Lecture 9: Reproduction - male embryonic and postnatal 27 β-galactosidase, which can be detected using a staining process that turns blue or another color when β-galactosidase is present. Cell Isolation and Injection: SSCs expressing the LacZ+ gene were isolated and then injected into the testes of recipient mice that had been depleted of their own germ cells. Monitoring and Analysis: After the injection, researchers observed the testis of these mice for the presence of β-galactosidase activity, indicating successful repopulation by the LacZ+ SSCs. Findings: Successful Repopulation: The testes of recipient mice that received the LacZ+ SSCs showed clear staining for β-galactosidase, confirming that the injected SSCs were able to repopulate the testis. Sperm Production: The repopulated testes were capable of producing sperm, demonstrating that the LacZ+ SSCs were functional and contributed to normal spermatogenesis. Visual Evidence: The LacZ+ staining provided direct visual evidence of the presence and activity of SSCs within the testis, allowing researchers to confirm their successful integration and function. Significance: Self-Renewal and Functionality: The experiment provided evidence that spermatogonial stem cells can self-renew and reestablish spermatogenesis, even in a testis that had been previously depleted of germ cells. Potential for Fertility Treatments: This research has important implications for fertility treatments, as it demonstrates that SSCs can potentially restore fertility in individuals with damaged or depleted testes. Utility of LacZ+ Gene: The LacZ+ gene was a crucial tool for tracking and visualizing SSCs, allowing researchers to monitor their integration and contribution to spermatogenesis. Lecture 9: Reproduction - male embryonic and postnatal 28 Markers and Selection: The presence of LacZ+ staining enabled researchers to identify and confirm the successful repopulation of the testis by these cells. Proportion of LacZ+ SSC in the transplant determines extent of LacZ+ spermatogenesis Lecture 9: Reproduction - male embryonic and postnatal 29 LO: Define the spermatogonial stem cell niche and key factors involved The spermatogonial stem cell niche – extrinsic factor in regulating SSCs The SSC niche is the specialised microenvironment within the testis where SSCs reside and receive support for their maintenance and function. Sertoli Cells: These are the key cells in the niche that provide essential support to SSCs. Provide many signals to maintain SSC niche - extrinsic factors GDNF & FGF2 Other cell types also contribute to the niche (e.g., Leydig and Myoid cells that are present between the cords in the interstitium) Lecture 9: Reproduction - male embryonic and postnatal 30 Experimental Manipulation of the Sertoli cells in the SSC niche: Experiments have shown that increasing the number of Sertoli cells in mice leads to an enhanced SSC niche. This results in increased spermatogenesis, meaning more sperm is produced. Conversely, if the Sertoli cell number is reduced, the niche becomes less supportive, leading to a decrease in spermatogenesis. GDNF promotes SSC self renewal GDNF (Glial Derived Neurotrophic Factor): a growth factor derived from Sertoli cells acts as a paracrine factor for SSC survival High levels of GDNF favour SSC self renewal, lower levels favor differentiation into B spermatogonia This balance is crucial for maintaining both the stem cell pool and the production of sperm Experiment: the role of GDNF established by mouse trangenics Targeted deletion of GDNF or its receptor → impaired spermatogenesis GDNF heterozygous mice (those with only one functional copy of the GDNF gene) ⇒ significant disruption in the testicular structure is observed, with a loss of the SSC pool and only Sertoli cells remaining by eight weeks postnatal development. Lecture 9: Reproduction - male embryonic and postnatal 31 Other cellular components and factors of the GSC niche FGF2 (Fibroblast Growth Factor 2): Produced by Sertoli cells, Leydig cells, and differentiating germ cells, FGF2 works alongside GDNF to promote SSC self-renewal. This synergy is crucial for maintaining the SSC population. CSF1 (Colony Stimulating Factor 1): Produced by Leydig cells and peritubular myoid cells (smooth muscle cells surrounding seminiferous tubules), CSF1 supports germline stem cell function within the niche. CXCL12: This chemokine plays a role in the "homing" of SSCs to their niche, ensuring that they stay within the supportive environment necessary for their function. Signaling Pathways: The factors mentioned above (GDNF, FGF2, CXCL12) activate signaling pathways within the cytoplasm of SSCs, leading to changes in gene expression in the nucleus. These changes ultimately determine whether the SSCs will self-renew, differentiate, or maintain their position within the niche. Lecture 9: Reproduction - male embryonic and postnatal 32 Aging and decline of the SSC niche Aging and the SSC Niche: As mice (and likely humans) age, the niche that supports SSCs deteriorates. This niche is the specialized environment within the testes that is crucial for maintaining SSC self-renewal and supporting spermatogenesis. With aging, the ability of the niche to support SSC self-renewal declines. This leads to a loss of germ cells (cells that give rise to sperm) and degeneration of the seminiferous epithelium, the tissue lining the seminiferous tubules where sperm is produced. Germ Cell Loss and Niche Deterioration: The passage mentions that while older men can still father children, as exemplified by Mick Jagger fathering a child at 72, there is still a loss of germ cells over time. This suggests that fertility may decline with age due to the deteriorating niche, even if some reproductive capacity remains. Experiments with aging mice have shown that when germ cells from older mice are transplanted into the testes of younger mice, there is evidence of germ cell loss and a decline in stem cell function. This Lecture 9: Reproduction - male embryonic and postnatal 33 transplantation experiment demonstrates that the aging niche is less capable of supporting SSCs. Reduced GDNF Levels: GDNF (Glial cell line-Derived Neurotrophic Factor), which is critical for SSC survival and self-renewal, is found in reduced levels in aging, infertile males. This reduction contributes to the decline in SSC function and spermatogenesis with age. Serial Transplantation Experiments: The passage describes an experiment where germ cells are serially transplanted from one mouse to another at regular intervals (every three months) into young recipients. This process successfully maintains stem cell function for over three years, which is significant since male mice typically lose fertility by around 18 months. This experiment suggests that the decline in spermatogenesis with age is primarily due to the deterioration of the niche, not an intrinsic loss of SSC function. By placing SSCs in a younger, healthier niche, their function can be preserved much longer than in an aging niche. LO: Understand that both extrinsic and intrinsic factors regulate SSCs Intrinsic Factors Controlling SSC Renewal: Focus on PLZF Role of PLZF in SSC Renewal: Lecture 9: Reproduction - male embryonic and postnatal 34 PLZF (Promyelocytic Leukemia Zinc Finger): PLZF is a transcription factor that plays a crucial role in the regulation of spermatogonial stem cell (SSC) renewal. Transcription factors like PLZF are proteins that bind to specific DNA sequences to control the transcription of genetic information from DNA to mRNA. In the context of SSCs, PLZF is essential for maintaining the balance between stem cell renewal and differentiation. Function of PLZF: PLZF regulates the expression of genes involved in SSC renewal. It acts by inhibiting the pathways and genes that drive the differentiation of SSCs into sperm. Instead, PLZF promotes the self-renewal of SSCs, ensuring that the stem cell pool is maintained over time. Impact of PLZF Mutation on SSCs: PLZF Knockout Mice: In experimental models where the PLZF gene is knocked out (i.e., the PLZF protein is not produced), there is a significant impact on the SSC population. These mice exhibit progressive germ cell loss and infertility. The underlying issue is that without PLZF, SSCs are unable to self-renew effectively. Loss of Self-Renewal: In PLZF knockout mice, SSCs continue to divide but fail to produce new stem cells. Instead, all the daughter cells produced from these divisions enter the differentiation pathway to become sperm. This results in the gradual depletion of the SSC pool because there is no replenishment of stem cells. Mechanism of Action: Inhibition of Differentiation Pathways: PLZF normally acts to inhibit multiple targets and pathways that are required for SSC differentiation. By inhibiting these pathways, PLZF ensures that SSCs maintain their stem cell identity and do not prematurely differentiate into sperm. Lecture 9: Reproduction - male embryonic and postnatal 35 Increased Differentiation Commitment: Without PLZF, the inhibition of differentiation pathways is lost, leading to increased commitment of SSCs to differentiate into sperm. This unchecked differentiation means that the stem cell population is eventually exhausted, leading to infertility as no new SSCs are produced. PLZF as an SSC Marker: SSC Marker: PLZF is used as a marker for identifying SSCs because its presence indicates the cells are in a state of self-renewal and have the potential to continue producing new stem cells. When PLZF is expressed, it signals that the SSCs are functioning normally and maintaining their stem cell characteristics. LO: Be aware of the heterogeneity model of SSC renewal Rodent and primate GSCs; conserved regulatory mechanisms Lecture 9: Reproduction - male embryonic and postnatal 36 While there are differences in the structure and terminology of spermatogonial stem cell (SSC) differentiation between mice and humans/primates, the core process and regulatory mechanisms are conserved MICE SSCs progress through stages labeled as A-singles, A-paired, A- aligned, and B-spermatogonia. HUMANs/PRIMATES The stages are termed A-dark, A-pale, and B-spermatogonia Despite these differences, both species follow a similar hierarchical progression from SSCs to differentiated cells, regulated by conserved molecular markers like GFRα1, PLZF, NGN3, and C-kit. This conservation suggests that insights from mouse studies can be applied to understanding human spermatogenesis. Translating basic GSC biology to human therapy Techniques allowing GSC manipulation can have multiple therapeutic potentials Lecture 9: Reproduction - male embryonic and postnatal 37 Cancer therapies (high-dose chemotherapy, whole-body irradiation) and myeloablative conditioning therapy for bone marrow transplantation are associated with a high infertility risk Cryopreservation of sperm samples prior to therapy is available to post- pubertal but not pre-pubertal males Restoration of male fertility after therapy might involve cryopreservation of GSC-containing testis samples prior to therapy and subsequent transplantation The ability to identify GSCs through use of specific markers and expand GSCs contained within small testis biopsies with in vitro culture can be vital for development of effective therapies Therapeutic options for preserving male fertility Deriving multipotent stem cells from SSCs ES-like cells can be derived from mouse SSCs Cultured SSCs can transition into multipotent stem cells three germ layers Potential uses: study mechanisms of stem cell differentiation Lecture 9: Reproduction - male embryonic and postnatal 38 in humans, personalised regenerative medicine (making tissues, organs) Lecture 9: Reproduction - male embryonic and postnatal 39