Lecture 15: Neural Stem Cells, MS, and Parkinson's PDF
Document Details
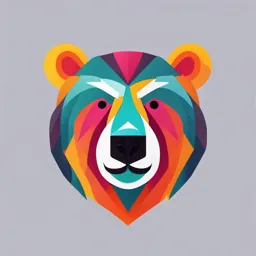
Uploaded by VeritableAmber980
Monash University
Tags
Summary
This document provides an overview of neural stem cells, MS, and Parkinson's disease. It covers the developmental origins of various structures within the nervous system, including cell types and their functions.
Full Transcript
🥽 Lecture 15: Neural Stem cells, MS, and Parkinson’s LO: The developmental origin of structures that give rise to the nervous system Central Nervous System - forms entirely from the neural tube Components:...
🥽 Lecture 15: Neural Stem cells, MS, and Parkinson’s LO: The developmental origin of structures that give rise to the nervous system Central Nervous System - forms entirely from the neural tube Components: Brain Brainstem Cerebellum Spinal Cord Cell types: Neurons: Primary functional units of the CNS. Glial Cells: Oligodendrocytes: Produce myelin. Astrocytes: Contribute to the blood-brain barrier. Lecture 15: Neural Stem cells, MS, and Parkinson’s 1 Involved in maintenance functions, such as potassium regulation. Ependymal Cells: Line the ventricles. Microglia: Innate immune cells of the CNS. Have several transcriptionally and functionally distinct classes. Peripheral Nervous System - forms entirely from the neural crest and cranial placode Components: Peripheral nerves (arising from the spinal cord). Contain axons of: Motor neurons, Sensory neurons, Autonomic neurons Sensory Ganglia: Clusters of cells outside the CNS. Contain sensory neurons and glial cells. Located in: Cranial region, Areas associated with the spinal cord Autonomic Ganglia: Contain autonomic neurons and glial cells. Located in the periphery. House motor neurons that supply smooth muscle Specific derivation: The neural crest, which gives rise to: Most of the autonomic nervous system. Peripheral nerves. Sensory neurons in the spinal and cranial ganglia. The cranial placodes, which contribute to: Cranial sensory ganglia. Parasympathetic ganglia. Lecture 15: Neural Stem cells, MS, and Parkinson’s 2 Key Swellings/Primary Vesicles and their Derivatives: Secondary Primary Vesicle Neural Derivatives Cavity Vesicle Cerebral hemispheres Lateral Prosencephalon Telencephalon Thalamus, ventricles (forebrain) Diencephalon hypothalamus, retina, Third ventricle other structures Mesencephalon Cerebral Mesencephalon Midbrain (midbrain) aqueduct Rhombencephalon Metencephalon Pons, cerebellum Part of fourth (hindbrain) Myelencephalon Medulla ventricle Part of fourth Lecture 15: Neural Stem cells, MS, and Parkinson’s 3 Secondary Primary Vesicle Neural Derivatives Cavity Vesicle ventricle, part of central canal Ventricular System Vesicles of the neural tube persist as the ventricular system. Components: Lateral ventricles, Third ventricle, Cerebral aqueduct, Fourth ventricle, Central canal Radial Unit Hypothesis Suggests that stem cells in the ventricular zone produce neurons that populate a column of cortical tissue - explaining how the cerebral cortex develop its columnar structure (1) Neural stem cells in the ventricular zone (VZ) produce neurons. (2) These neurons migrate along radial glial fibres, extending from the VZ to the outer cortical surface. (3) Neurons organize into columns, forming the basic structural units of the cortex. Lecture 15: Neural Stem cells, MS, and Parkinson’s 4 (*) Additional cells, like interneurons and glial cells, may migrate in from other brain regions to integrate into these columns. Zones of the Developing Cortex 1. VZ - Ventricular Zone Innermost layer near the ventricles. Contains proliferative neural stem cells that give rise to neurons and glial cells. 2. IZ - Intermediate Zone Located between the VZ and cortical plate (CP). Acts as a migratory pathway for neurons traveling to their final destinations. 3. SP - Subplate Transitional zone beneath the cortical plate. Temporarily houses neurons and supports initial connections during development. 4. CP - Cortical Plate Precursor to the future cortex. Destination for neurons migrating from the VZ, where they form layers of the mature cerebral cortex. 5. MZ - Marginal Zone Outermost layer of the developing cortex, near the pial surface. Contains axons and sparse neurons, including Cajal-Retzius cells, which guide cortical organization. Lecture 15: Neural Stem cells, MS, and Parkinson’s 5 Radial Glial Cells and Cortical Development Radial glial cells extend from the ventricular surface to the pial surface (apical to basal). Functions of radial glial cells: 1. Self-renewal via cell division. 2. Production of neurons that migrate along their fibres. 3. Transition into astrocytes, later contributing to the blood-brain barrier. Modes of Division in Radial Glial Cells 1. Vertical Plane Cleavage (Symmetric Division) Early in development. Produces two identical daughter cells. Drives the expansion of the ventricular zone. 2. Horizontal Plane Cleavage (Asymmetric Division) Later in development. Produces one radial glial cell and one basal progenitor (intermediate progenitor). Basal progenitors divide to produce neurons that colonize the cortex. Lecture 15: Neural Stem cells, MS, and Parkinson’s 6 Lecture 15: Neural Stem cells, MS, and Parkinson’s 7 Interkinetic Nuclear Migration (IKNM): Radial glial cells oscillate their nuclei between the ventricular surface and the pial surface during the cell cycle. Division occurs at the ventricular surface. Basal progenitors lack attachment to the pial surface and do not undergo IKNM. Outer Radial Glial Cells (oRGCs) Characteristics: 1. Attach only to the pial surface, not the ventricular surface. 2. Divide to produce daughter cells that migrate and contribute to cortical growth. 3. Found in the outer subventricular zone (OSVZ). Humans have significantly more oRGCs than other species like mice, which contributes to the greater expansion and complexity of Lecture 15: Neural Stem cells, MS, and Parkinson’s 8 the human cortex. Subventricular Zone (SVZ) and Outer Subventricular Zone (OSVZ) The SVZ can be subdivided into: 1. Inner SVZ (ISVZ). 2. Outer SVZ (OSVZ): Expanded in humans, highly populated by oRGCs. Both zones contribute progenitor cells that colonize and expand the cortex. Spatial and Temporal Relationship Between Nucleus Position and Cell Cycle Phase Radial glial stem cells demonstrate a strong relationship between their nucleus position and the cell cycle phase: 1. G1 Phase: The cell nucleus migrates upward toward the margin between the inner and outer ventricular zone. Lecture 15: Neural Stem cells, MS, and Parkinson’s 9 2. S Phase: At the margin, the nucleus doubles its DNA in preparation for division. 3. G2 Phase: The nucleus migrates downward toward the ventricular surface. 4. M Phase: Once at the ventricular surface, the cell divides. 5. G0 Phase: Cells exiting the cell cycle migrate away. Cells that become basal progenitors move into the subventricular zone, where they can continue dividing. Zones of Proliferation 1. Ventricular Zone (VZ) and Subventricular Zone (SVZ): Key areas for the accumulation of proliferating cells. 2. Subventricular Zone (SVZ): Contains basal progenitors that divide and contribute to the cortical layers. Cortical Colonisation Cells exiting the ventricular zones migrate to the cortical layers in an "inside-out" pattern: Newborn cells migrate past older cells to colonize the outer layers. Exception: The outermost cortical layer, which forms early in development Eg) in the diagram - older green cells already present in deeper layers, younger red cells mirgate outward to form new layers, except for the outermost layer, which devepops first. Lecture 15: Neural Stem cells, MS, and Parkinson’s 10 LO: The relationship between neural stem cell division time and differentiation Division Rates of Radial Glial and Basal Progenitors Apical Progenitors/Radial Glial Cells: Divide faster than basal progenitors. Exhibit a shorter cell cycle length during early development. Basal Progenitors: Divide slower than apical progenitors. Differentiation is influenced by the length of the cell cycle. Outer Radial Glial Cells: Similar pattern to apical progenitors, dividing faster than their basal progenitor counterparts. Link Between Cell Cycle Length and Differentiation Fast Divisions (Short Cell Cycle Length): Early in development, result in progenitors with broad developmental potential. Slower Divisions (Extended Cell Cycle Length): Lecture 15: Neural Stem cells, MS, and Parkinson’s 11 Lead to production of basal progenitors or differentiated neurons. Ultimately generate postmitotic cells that leave the proliferative zone. Critical Phase in Cell Cycle Length Regulation: Changes primarily affect the G1 phase. Some evidence suggests the G2 phase may also vary, but the impact is minor compared to G1. Experimental Manipulations of Cell Cycle 1. Speeding Up the G1 Phase: Promotes expansion of progenitor pools. 2. Slowing Down the Cell Cycle: Increases the likelihood of differentiation into neurons. Leads to a depletion of progenitor pools. Importance of Controlled Cell Cycle Dynamics Tight Regulation Is Essential: Avoids premature depletion of progenitor pools. Prevents overproduction of progenitors late in development, ensuring proper cell type balance. Lecture 15: Neural Stem cells, MS, and Parkinson’s 12 Measuring cell cycle kinetics: Cumulative labelling with thymidine analogues Thymidine Analogues (e.g., BrdU, EdU): Substances that substitute for thymidine during DNA synthesis in the S phase. Only cells in S phase incorporate the tracer. Used to measure cell cycle dynamics in vivo, particularly in the cortex for neuronal production. How Cumulative Labelling Works 1. Tracer Administration: Continuous delivery of thymidine analogues to tissues. Cells in S phase take up the tracer and continue dividing. 2. Saturation Point: Cells accumulate tracer over time until saturation is achieved in the proliferative zone. This process is graphically represented as a curve. 3. Key Assumptions: All quiescent cells (G0 phase) exit the proliferative zone. No G0 cells re-enter the cell cycle after exiting the zone. Application to Glial Lineages Lecture 15: Neural Stem cells, MS, and Parkinson’s 13 Measuring glial cell pose difficulties as they are dispersed throughout the tissue rather than localised anatomically Progeny in G0 cannot be distinguished from dividing cells in the tissue Oligodendrocyte Precursor Cells (OPCs) Anatomy and Function: Evenly distributed throughout the cortex. Divide and differentiate into oligodendrocytes, which: Produce myelin for saltatory conduction. Nourish axons. Essential for survival; lineage deletion is incompatible with life. Population Dynamics: Comprise approximately 5% of cortical cells. Present and dividing throughout life. Cumulative Labelling in OPCs Findings: Cell cycle length slows significantly over time: From 6–8 hours to 80–100 hours. Lecture 15: Neural Stem cells, MS, and Parkinson’s 14 Nearly all cells divide at any given instant. Discrepancies in Observations In Vivo vs. In Vitro: In Vivo: Cell cycle length slows with age. In Vitro: Cell cycle length is consistently shorter (30–70 hours). Single-Cell RNA Sequencing Findings: In adults: ~3% express proliferation markers. In juveniles: ~15% express proliferation markers. Human vs. Rodent Data: Human data suggest most cell production occurs early, with a significant decline in later years. Measuring cell cycle dynamics without key assumptions required Ie. measuring cell cycle dynamics without needing to satisfy the two key assumptions → All quiescent cells (G0 phase) exit the proliferative zone + No G0 cells re-enter the cell cycle after exiting the zone. Double Labeling Protocol: Step 1: First Injection: Inject a thymidine analogue, like BrdU (Bromodeoxyuridine), which gets incorporated into DNA during S phase. Step 2: Second Injection: After a set interval (e.g., 2 hours), inject a second analogue, Edu (5-ethynyl-2'-deoxyuridine), into the tissue. Cells that are still in S phase will incorporate both tracers. Step 3: Labeling Results: Cells that have exited S phase will only show the first tracer (BrdU). Cells still in S phase will show both tracers (BrdU and Edu). The ratio of BrdU and Edu incorporation can be used to calculate the duration of S phase and cell cycle dynamics. Lecture 15: Neural Stem cells, MS, and Parkinson’s 15 LO: That expansion of the outer subventricular zone with increased stem cell diversity results in a wider range of cortical subtypes The brain develops as a tube-like structure with signaling gradients running from ventral to dorsal, and rostral to caudal (i.e., front to back, top to bottom). Lecture 15: Neural Stem cells, MS, and Parkinson’s 16 Morphogen gradient help pattern the developing tube and guide cell production Cell Types Produced from Different Ventricular Regions: Ventricular Surface: Different regions along the ventricular surface produce different types of cells (neurons and glia). This patterning continues into adulthood, where the regions maintain distinct cell types. Ageing Effects: Lecture 15: Neural Stem cells, MS, and Parkinson’s 17 As development progresses into adulthood, the cells that remain active (called B cells, or adult stem cells) no longer look like the original radial glial cells. These B cells can still produce neurons, but their appearance and functionality differ from those in earlier stages of development Expansion of the Subventricular Zone in Humans: Human Development: The outer subventricular zone (OSVZ) significantly expands during human brain development, which is unique to human development compared to other species like mice. Expansion of the OSVZ: The expansion of the outer subventricular zone plays a key role in generating a larger cortex and increased cortical cell numbers. Lecture 15: Neural Stem cells, MS, and Parkinson’s 18 This expansion results in increased complexity of cortical cells in humans. Mouse vs. Human Development: Mouse (E12): Early on, progenitor cells (marked in green) and basal progenitors (marked in yellow) are seen. Human (early development): Sox2 marks the radial glial cells, while TBR2 marks the intermediate progenitors, indicating differentiation of cells along this axis. The original radial unit hypothesis evolve with developmental changes: Early development is dominated by a continuous radial scaffold. Later stages involve a discontinuous scaffold, reflecting changes in cortical growth and organization while maintaining a columnar growth pattern. Evidence ^^: Lecture 15: Neural Stem cells, MS, and Parkinson’s 19 Crystal Labelling Technique: crystals applied to different surfaces of the human brain at various developmental stages. Findings from Outer Surface Labelling: Early Development: Crystals applied to the outer brain surface label cells that extend radially, spanning from the ventricular zone (VZ) to the pia (outer surface). This represents the full radial unit where cells migrate up and down the scaffold formed by RGCs. Later Development: Labelling becomes restricted to cells closer to the outer radial glial cells (oRGs). These cells attach to blood vessels instead of spanning the entire radial scaffold. Findings from Inner Surface Labelling: Early Development: Crystals applied to the ventricular surface label cells throughout the full radial scaffold, from the VZ to the pia. Later Development: Labelling is restricted to cells terminating within the ventricular zone, indicating a shift in the scaffold's organization. Structural Shift During Development: Early Stages: The scaffold formed by RGCs is continuous, supporting columnar organization throughout the cortical layers. Later Stages: The scaffold becomes discontinuous, with a split between: Outer radial glial cells (oRGs) supporting the outer regions. Lecture 15: Neural Stem cells, MS, and Parkinson’s 20 Cells terminating within the ventricular zone supporting the inner regions. LO: The sites of adult neurogenesis Sites of Adult Neurogenesis: 1. Lateral Ventricles: Stem cells reside along the ventricular wall, specifically in the subventricular zone (SVZ). Typical arrangement: Radial glial-like cells (B1 cells): Extend processes to blood vessels and cerebrospinal fluid (CSF). Give rise to transient amplifying cells, which differentiate into: Neuroblasts (neurons). Glial cells. Line the ventricular surface as ependymal cells. Lecture 15: Neural Stem cells, MS, and Parkinson’s 21 2. Dentate Gyrus (Hippocampus): Similar organization of radial glial-like cells supporting neurogenesis. Key role in memory formation and learning. Evidence of Adult Neurogenesis: 1. Rodent Studies: Labelling techniques like BrdU or EdU demonstrate neurogenesis in mice and other rodents. 2. Human Studies: BrdU Experiments: Cancer patients administered low-dose BrdU for monitoring cell proliferation. Postmortem analysis revealed BrdU-positive neurons co- expressing neuronal markers. Carbon-14 Dating: Levels of Carbon-14 (spiked during nuclear bomb testing) incorporated into DNA during cell division were analyzed. Provides evidence of newly generated neurons correlating with the individual's exposure timeline. 3. Functional Evidence in Rodents: Adult neurogenesis supports: Formation of complex types of memory. Myelination plasticity (adaptive myelination). 4. Challenges in Human Studies: Opposing studies: Criticism due to poor tissue quality and inconsistent protocols for applying marker panels. Consensus: Neurogenesis occurs at a low rate but is still present in adult human brains. Lecture 15: Neural Stem cells, MS, and Parkinson’s 22 5. Limitations of Using Neural Stem Cells: Modelling Disease: Limited capacity to replicate the complexity of human neurogenesis. Therapeutic Targets: Challenges in: Harvesting and maintaining stem cells. Ensuring precise integration into damaged brain areas. LO: The potential, and limitations, of using stem cells to study disease processes, or as therapeutic targets for future clinical application Multiple Sclerosis (MS) and Myelin Regeneration Overview of Multiple Sclerosis (MS) MS is an autoimmune disease where the immune system attacks the myelin sheath, which: Lecture 15: Neural Stem cells, MS, and Parkinson’s 23 Insulates axons and enables fast saltatory conduction. Provides metabolic and trophic support to axons. Impact of Myelin Loss: Demyelinated axons become compromised and may degenerate irreversibly, worsening functional and prognostic outcomes. Oligodendrocytes and Myelin Regeneration Myelin is produced by oligodendrocytes. Oligodendrocyte Precursor Cells (OPCs): Present in tissue and capable of producing new myelin post- demyelination. However, this process is inefficient in humans, making it a promising therapeutic target. Key Study on Stem Cell Contributions to Remyelination Study Overview: Investigated the role of stem cells and OPCs in remyelination. Methodology: 1. Demyelination Injury: Induced via a chemical agent. 2. Green Fluorescent Protein (GFP) Labelling: GFP turned on in stem cells (not OPCs) before the demyelinating lesion using a tamoxifen-based system with Cree ERT2. Tracked GFP-positive cells during the remyelination process. Findings: Stem Cells: Migrated to the injury site and produced thick myelin. GFP-positive myelin was thicker and of better quality compared to GFP-negative myelin. OPCs: Also contributed to remyelination. Lecture 15: Neural Stem cells, MS, and Parkinson’s 24 Myelin produced was thinner and less robust compared to that produced by stem cells. Genetic Manipulation to Enhance Myelination Studies have shown: Genetic modifications of stem cells can favor oligodendrocyte production. Targeting key transcription factors like OLIG2 enhances myelin production. Recent Breakthroughs: Demonstrated potential for remyelination even in the spinal cord by: Overexpressing OLIG2 in ependymal cells lining the central canal. Resulting in these cells contributing to myelin regeneration. Using Stem Cells in Research and Therapy Lecture 15: Neural Stem cells, MS, and Parkinson’s 25 Applications of Stem Cells 1. Understanding Development and Disease: Efficient methods for generating different cell types from human stem cells. Study normal development and disease processes by modifying genes linked to specific diseases. 2. Cell Replacement Therapy: Create human cells for transplantation to replace damaged or lost cells. Challenges of Targeting Stem Cells in the Brain Risk of developing tumorigenic cells when manipulating stem cells in situ. Alternative strategies: Generate cells externally and transplant them. Use induced pluripotent stem cells (iPSCs) or embryonic cells, with iPSCs preferred due to ethical considerations. Organoids as a Model System Organoids mimic brain tissue and are derived from stem cells. Applications: Lecture 15: Neural Stem cells, MS, and Parkinson’s 26 Study cellular biology and disease mechanisms. Test compounds promoting oligodendrocyte differentiation. Investigate demyelination and repair mechanisms in vitro. Key Achievements in Organoid Research Myelinating Organoids: Collaboration with Samantha Barton (Monash alumna, Edinburgh researcher). Created organoids containing neurons, astrocytes, and myelinating oligodendrocytes. Differentiation protocol: Neutralisation using Smads and retinoic acid. Organoids exhibit oligodendrocytes capable of producing myelin. Applications: Testing compounds, studying cell lineage growth, and repair mechanisms. Introduction of Microglia into Organoids: Microglia derived from the yolk sac, distinct from neuroepithelial origin. Separate generation of microglia and their integration into organoids. Created organoids with neurons, astrocytes, oligodendrocytes, and microglia. Potential to study immune-related mechanisms in diseases like MS. Limitations of Organoid Models 1. Transcriptional Discrepancies: Differences in gene expression between organoid cells and primary tissue. 2. Lack of Cellular Architecture: Organoids lack the cortical layering seen in human brains. Lecture 15: Neural Stem cells, MS, and Parkinson’s 27 Efforts are underway to pattern organoids toward cortical structures. 3. Absence of Blood Vessels: Organoids lack vascularisation, although work is ongoing to introduce blood vessels by transplantation into animals. 4. Cellular Stress: Organoid neurons exhibit stress markers not present in primary tissue. Stem Cells in Parkinson’s Disease Therapy Overview of Parkinson's Disease (PD) Cause: Loss of midbrain dopaminergic neurons projecting to the basal nuclei. Impact: Leads to severe movement disorders. Progression: By the time symptoms appear, 70-80% of dopaminergic neurons are already lost. Methods of Producing Dopaminergic Neurons 2 methods of deriving dopaminergic neurons - differ in timing of delivery of growth factors, and which growth factors used: 1. Floor Plate Method: Uses hedgehog and GSK signaling to differentiate cells into dopaminergic neurons. 2. Rosette-Derived Method: Similar to methods used for generating neural cells in organoids. Produces dopaminergic neurons that show better survival rates and functional outcomes. Lecture 15: Neural Stem cells, MS, and Parkinson’s 28 Results from Mouse Models Transplantation: Dopaminergic neurons derived from both methods were transplanted into PD mouse models. Outcomes: Rosette-derived neurons (labelled red in images) exhibited greater stability and survival. Mice demonstrated improved motor function, as assessed using the rotarod test (measures balance and coordination). Lecture 15: Neural Stem cells, MS, and Parkinson’s 29