Topical Drugs and Skin Anatomy PDF
Document Details
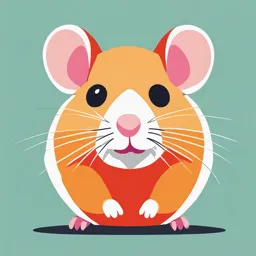
Uploaded by EncouragingTurkey
Tags
Summary
This document provides an overview of topical drug application, covering the reasons for topical application and different dosage forms. It also details skin anatomy, specifically the epidermis and its layers, with a focus on the stratum corneum, including its composition and role as a barrier. It also discusses the importance of the various layers of the skin.
Full Transcript
3.1 Topical Drugs: Reasons for Topical Application ○ Protecting injured skin from the environment. ○ Treating various skin conditions like eczema and psoriasis. ○ Relieving joint pain, as seen with diclofenac gel (Voltaren). ○ Syste...
3.1 Topical Drugs: Reasons for Topical Application ○ Protecting injured skin from the environment. ○ Treating various skin conditions like eczema and psoriasis. ○ Relieving joint pain, as seen with diclofenac gel (Voltaren). ○ Systemic drug delivery via transdermal patches, such as nicotine and fentanyl patches. Dosage Forms for Topical Administration ○ Liquids: Solutions, lotions, tinctures, collodions, and medicated shampoos. ○ Semisolids: Ointments, creams, pastes, and gels. ○ Solids: Powders and sticks. ○ Others: Aerosols, foams, dressings, tapes, and plasters. Skin Anatomy and Physiology ○ The skin, the largest organ, varies in thickness and weighs about 3-4 kilograms in adults. ○ Protective Barrier: The skin serves as a barrier against various external factors, including microorganisms, chemicals, radiation (like UV rays), electrical shocks, and mechanical injuries. ○ Physiological Roles: Regulates temperature through sweating, excretes substances, and plays a role in drug absorption. ○ Complex Structure: The skin is intricate both physiologically and biochemically. Skin Layers and Appendages ○ The skin comprises three primary layers: the epidermis, the dermis, and the subcutaneous fat layer. ○ It also includes appendages, such as sweat glands (apocrine and eccrine) and hair follicles. The Epidermis in Detail Composition: The epidermis, the outermost layer, is a stratified squamous epithelium constantly renewing itself. ○ Its thickness varies across different body areas. ○ It mainly consists of keratinocytes, cells containing the protein keratin. ○ Other cell types interspersed within the epidermis include: Langerhans cells: Macrophages involved in the skin's immune defense. Melanocytes: Produce melanin, protecting against UV radiation and contributing to skin color. Merkel cells: Involved in fine touch sensation. Layers of the Epidermis: ○ Stratum corneum: The outermost layer, acting as the primary protective barrier. ○ Stratum lucidum: A thin layer found mainly on the palms and soles. ○ Stratum granulosum: Crucial for producing lipids contributing to the skin's barrier properties. ○ Stratum spinosum: Found throughout the body. ○ Stratum basale (or stratum germinativum): The deepest layer responsible for generating new keratinocytes. ○ Keratinocytes: ○ Make up 80% of the epidermis. ○ Named for their high content of keratin, a structural protein providing strength and toughness to skin, hair, and nails. ○ Organized into layers, or strata, which contribute significantly to the skin's barrier properties. ○ Classified as either viable (living) or non-viable (dead). Viable epidermis includes all layers except the stratum corneum. Non-viable epidermis solely consists of the stratum corneum, composed of dead cells. Focus on the Stratum Corneum Primary Barrier: The stratum corneum is the most critical barrier to drug penetration, with its structure and composition playing a key role in this function. Brick and Mortar Structure: ○ The stratum corneum is organized like a wall, with corneocytes (dead keratinocytes) acting as bricks and intercellular lipids serving as mortar. ○ ○ Corneocytes: The end product of keratinocyte differentiation, flat, enlarged, overlapping, and interspersed with lipid sheets. Dead cells lacking a nucleus and organelles. Contain 10-20% water, a crucial factor in preventing dryness and maintaining skin health. Rich in keratin and filaggrin, structural proteins providing the stratum corneum's skeleton. Contain low molecular weight polar compounds, primarily polar amino acids, collectively known as the Natural Moisturizing Factor (NMF). NMF attracts water from the underlying dermis, maintaining hydration. ○ Intercellular Lipids: Composed of ceramides, free fatty acids, and cholesterol, mimicking the composition of CeraVe moisturizing products. Produced by the stratum granulosum. Crucial for holding corneocytes together, limiting permeation of substances (both inward and outward), and preventing excessive transepidermal water loss. Desmosomes: ○ Specialized junctions acting like spot welds to bind corneocytes together. ○ Contribute to the structural integrity and barrier function of the stratum corneum. ○ Breakdown of desmosomes can lead to excessive desquamation, resulting in skin flaking. ○ Continuous Renewal: ○ Corneocytes slough off after about 14 days in the stratum corneum, a process referred to as molting. ○ Skin shedding is continuous, with individual cells being replaced by new cells from the basale layer. ○ Turnover time varies across different body regions (e.g., 6 days on the forehead, 21 days on the back of the hand). Deeper Layers and Appendages: The Dermis: ○ Located beneath the epidermis and significantly thicker (about 40 times). ○ Composed of a connective tissue matrix, providing support and strength to the epidermis. ○ Responsible for skin structure and elasticity; alterations in the dermis lead to wrinkles and scars. ○ Contains nerves, appendages (sweat glands and hair follicles), and blood and lymphatic vessels. ○ Drugs reaching the dermis can be absorbed into systemic circulation via these blood and lymphatic vessels. ○ Subcutaneous Fat: ○ The deepest layer, primarily composed of adipose tissue. ○ Can act as a reservoir for certain drugs, especially lipophilic ones, potentially delaying absorption or serving as a depot for slow release. ○ Skin Appendages: ○ Include: Sweat glands: Eccrine: Widely distributed and responsible for thermoregulation via sweating. Apocrine: Secrete into hair follicles. Sebaceous glands: Associated with hair follicles. Produce sebum, a lipid mixture forming a film on the skin surface. Sebum provides waterproofing, lubrication, and contributes to the acid mantle. The Acid Mantle: ○ An acidic environment on the skin surface with a pH ranging from 4.5 to 6.2. ○ Created by fatty acids present in sebum. ○ Protective Functions: Antimicrobial: Inhibits the growth of certain pathogens. Orderly Desquamation: Ensures proper shedding of individual skin cells. Lipid Lamellae Formation: Supports the creation of the protective lipid layers between corneocytes. ○ pH Dependence: The functions of the acid mantle are pH-dependent, relying on the optimal activity of specific enzymes. ○ Disruptions in the acid mantle's pH can impair these functions, potentially leading to issues like flaky skin or increased permeability. ○ Hair Follicles: ○ Present throughout the body except for lips, palms, soles, and certain areas of the sex organs. ○ Like sweat glands, hair follicles can act as pathways for drug penetration, bypassing the stratum corneum to some extent. Drug Transport Mechanisms Topical Drug Action: ○ Some topical drugs act on the skin surface (e.g., Vaseline, Neosporin, DEET-containing insect repellents, moisturizers, exfoliants). ○ Others must penetrate various skin layers to reach their target sites. ○ Examples of drugs targeting different skin layers: Stratum Corneum: Exfoliants targeting clogged hair follicles. Appendages: Antiperspirants targeting sweat glands, antimicrobials for follicular infections, and depilatories. Deeper Layers: Topical corticosteroids for anti-inflammatory effects. Dermis: Drugs requiring transdermal delivery for systemic absorption. Drug Permeation Steps: ○ Drug application to the skin. ○ Drug dissolution and release from the dosage form (e.g., cream, ointment). ○ Entry into appendages or penetration through the stratum corneum and deeper layers. Drug Permeation Challenges: ○ The skin's primary function is protection, creating a significant barrier to drug penetration. ○ Drug permeation is typically slow, taking hours to permeate the epidermis, especially through the stratum corneum. ○ Damaged skin allows for faster drug penetration, and specialized formulations can temporarily disrupt the stratum corneum to enhance permeation. Routes of Drug Permeation: ○ Transepidermal: The primary route, involving passage through the epidermal layers, including the stratum corneum. ○ Transappendageal: A less significant route due to the limited surface area covered by appendages (hair follicles and sweat glands). Allows for bypassing the stratum corneum to some extent, potentially leading to faster diffusion. Transepidermal Transport Most important route Stratum Corneum as the Major Barrier: ○ Drugs must cross the stratum corneum and viable layers to reach the dermis. ○ The stratum corneum presents the most significant obstacle due to its brick and mortar structure. Pathways Through the Stratum Corneum: ○ Transcellular: Passage through corneocytes, requiring permeation across protein-lipid cell membranes. A relatively polar pathway due to the partially hydrated protein matrix within corneocytes. ○ Paracellular: The dominant route for most drugs, involving movement around corneocytes and through the lipid lamellae. Requires navigating multiple layers of lipid sheets, contributing to the slow permeation rate. A relatively non-polar pathway due to the prevalence of lipids. ○ Lipophilicity Favored: ○ The stratum corneum's lipophilic nature favors the permeation of lipophilic drugs. ○ Lipophilicity is a key consideration for drug selection and formulation development for topical applications. Viable Layers: ○ Once a drug crosses the stratum corneum, it usually diffuses rapidly through the viable epidermal layers. ○ Viable layers, especially compared to the stratum corneum, don't offer significant resistance to drug penetration. Systemic Absorption: ○ Upon reaching the dermis, systemic absorption of the drug typically occurs quickly. ○ Transappendageal Transport Appendages as Bypasses: ○ Sweat glands and hair follicles allow drugs to circumvent the stratum corneum to some degree, potentially facilitating faster entry into the viable epidermis and dermis. Limited Contribution: ○ Despite bypassing the stratum corneum, transappendageal transport is a minor pathway for drug delivery. ○ The reason for this limited contribution is the small surface area covered by appendages compared to the total skin surface. Regional Variations: ○ The density of appendages varies across body regions, influencing the extent of transappendageal drug transport. ○ Areas with a higher concentration of appendages may exhibit faster drug absorption. Factors Influencing Drug Permeation: Biological Factors: ○ Interpatient Variability: Permeability can differ tenfold between individuals at the same site. ○ Sources of Variability: Region of the body: Epidermal thickness and lipid composition variations affect permeability. This variation is particularly important for transdermal patches, which must be applied to specific, manufacturer-approved sites for optimal drug delivery. Skin condition: A healthy epidermis is crucial for barrier function. Diseased, damaged, or compromised skin can significantly alter drug penetration. For example, individuals with eczema have a less effective skin barrier. Drug metabolism: Viable epidermis contains drug-metabolizing enzymes like CYPs and esterases, leading to a cutaneous first-pass effect. This effect is generally less pronounced than hepatic first-pass metabolism. Examples of cutaneous metabolism: Nitroglycerin: Up to 15-20% can be metabolized in the skin. Tazarotene: A prodrug for psoriasis, metabolized by skin esterases to its active form. Drug binding: Binding to components within the stratum corneum (proteins and lipids) can impact drug availability. Drug binding can delay the onset of action by slowing down drug release and penetration. It can also create a drug reservoir, prolonging drug effects even after removing the drug source (e.g., a transdermal patch). Patient's age: Premature infants have an underdeveloped stratum corneum, making them highly susceptible to drug penetration. Extreme caution is needed when applying medications to their skin. Aged skin can thicken and become less hydrated, making the effects on permeation less predictable. These changes can be further complicated by alterations in dermal microcirculation, affecting drug removal. Physical-Chemical Factors (Modifiable by Formulation): ○ Hydration state of the skin: Normal stratum corneum water content is low (10-20%) but essential for its function. Stratum corneum can absorb significant amounts of water, up to 75%, leading to swelling and softening. This water uptake occurs within the cells and interlamellar spaces. Hydration typically enhances drug permeation by expanding the pathways within the stratum corneum. ○ Occlusion: Blocking transepidermal water loss (TEWL), achieved through occlusive dressings, greasy ointments, or patches, increases skin hydration and drug permeation at the site of application. Occlusion expands the pathways within the stratum corneum for drug penetration by trapping moisture. ○ Drug properties: Lipophilic (non-polar) drugs are favored over hydrophilic (polar) drugs due to the stratum corneum's lipid-rich nature. Non-ionized drug forms, being more lipophilic, permeate more readily than ionized forms. ○ Dosage form properties: Drug release: The dosage form must effectively release the drug for it to be absorbed. Occlusion: Different dosage forms vary in their occlusive properties (e.g., Vaseline is highly occlusive, while lotions are less so). Occlusive properties impact skin hydration and drug permeation. 3.2 Topical Drug Delivery Dosage forms are classified as liquids, semisolids, and solids. Semisolids Ointments are semisolid preparations intended for external application to the skin or mucus membranes. ○ They usually, but not always, contain a medicinal substance. ○ Ointments are classified based on their interaction with water: Hydrocarbon (Oleaginous) bases are very greasy and insoluble in water. They are anhydrous and do not absorb water. Examples include: White petrolatum USP , a highly refined mixture of saturated hydrocarbons. The most well-known brand is Vaseline. White ointment USP , similar to white petrolatum, but with added wax (typically beeswax) for stiffness and smoothness. Therapeutic properties: Form an adherent, continuous, greasy, and water-repellent film. Good occlusiveness (block transepidermal water loss). Emollient effect (softens the skin). Enhance drug permeation. Prolonged contact with the skin, increasing drug residence time. Good protection. Patient acceptance: Greasy and may feel unpleasant. Not water washable. Can stain clothing. Absorption bases are greasy, anhydrous, and can absorb water due to the presence of surfactants. They typically form water-in-oil emulsions when water is incorporated. Examples include: Lanolin , a natural absorption base extracted from sheep wool. Hydrophilic petrolatum USP , commercially known as Aquaphor. Contains petrolatum, mineral oil, mineral wax, and wool wax alcohol (the main surfactant). Therapeutic properties: Form an adherent, greasy film. Moderately occlusive. Moderately good emollient and protective properties. Remain on the skin for a prolonged period. Can enhance drug delivery. Patient acceptance: Greasy and not water washable. Emulsion bases come in two types: Water-in-oil, where oil is the external phase. They absorb less water than anhydrous absorption bases because they already contain water. Examples include: Hydrous lanolin , anhydrous lanolin with added water (20-30%). Eucerin , similar to Aquaphor but with added water and preservatives. Therapeutic properties: Somewhat occlusive, but less so than anhydrous or hydrocarbon bases. Moderately good emollient and protective properties. Less ability to block transepidermal water loss. Patient acceptance: Somewhat greasy, but not as greasy as anhydrous absorption bases. Not easily water washable, but easier to wash off than Vaseline. More pleasant to apply than anhydrous absorption bases. Oil-in-water , where water is the external phase. They can absorb varying amounts of water and can be transformed into lotions by adding and mixing water. Water washable. Often considered the classic creams. Examples include: Hydrophilic ointment USP , commercially known as vanishing cream base. Therapeutic properties: Upon application, the water evaporates, leaving a thin, transparent film. High concentration of drug film enhances drug delivery. Less occlusive than water-in-oil emulsion bases and do not block transepidermal water loss. Less protective and emollient. Patient acceptance: Non-greasy and easy to apply. Highly acceptable. Water-soluble bases are uncommon and made of polyethylene glycols (PEGs). They feel greasy but are water soluble and non-occlusive. Examples include: Mupirocin ointment , formerly known as Bactroban, contains PEG 400 and a higher molecular weight PEG. Patient acceptance: Acceptable. Water washable and greaseless. Can be irritating to wounds. Pastes are ointments containing a high percentage (20-50%) of fine powder. ○ The base is usually petrolatum. ○ Examples of powders used in pastes: Zinc oxide. Talc. Starch. Bentonite. ○ Examples include: Boudreaux's Butt Paste , a skin protectant used for diaper rash. ○ Therapeutic properties: Form a thick, protective film upon application. Less greasy and occlusive than the corresponding base without powder. Absorptive. More protective and stiff. ○ Application: Applied as a thick film, unlike other topical dosage forms. Liquids Lotions are fluid emulsions or suspensions intended for external application. ○ The continuous phase is aqueous. ○ Examples include: Calamine lotion. Clotrimazole lotion. Selenium sulfide lotion. Cetaphil , an oil-in-water emulsion marketed as a lipid-free lotion. A good moisturizing lotion and general-purpose lotion vehicle. ○ Therapeutic properties: Upon application, the continuous phase evaporates, leaving a thin film of powder or liquid. Non-occlusive. Evaporation cools and soothes the skin. Fluidity allows for quick application over large areas and facilitates application to challenging areas like hairy or intertriginous areas. ○ Patient acceptance: Highly acceptable. Water washable, non-greasy, and easy to apply. ○ Stability: Similar to oral suspensions and emulsions, they can experience physical instability (separation, settling), chemical instability, and microbiological instability. Topical solutions are generally aqueous vehicles, sometimes containing alcohol. ○ Examples include: Burrow's solution. Dakin's solution (diluted sodium hypochlorite). Tinctures use alcohol or hydroalcoholic vehicles. ○ Example: Iodine tincture. Gels: An In-Depth Study Guide Gels are a significant category of semi-solid topical dosage forms. Here's a detailed examination of gels based on the provided source: Definition: Gels are defined as semi-solid systems where a liquid phase is confined within an interconnected polymeric matrix. This matrix can originate from natural or synthetic gums, with the term "gum" in pharmacy often signifying a polysaccharide. Composition: ○ Liquid Phase: Typically, the liquid phase is water. However, it can also contain miscible co-solvents like alcohols such as ethanol or isopropyl alcohol. ○ Drug: The drug is usually either suspended or dissolved within this liquid phase. ○ Gelling Polymers: The polymers responsible for gel formation are frequently the same as those employed as suspending agents in oral suspensions, only in higher concentrations to achieve the desired gel-like consistency. Examples include: Carbomer Tragacanth Alginic Acid Cellulose Derivatives (e.g., methylcellulose, carboxymethylcellulose) Characteristics: Most gels are designed to be both water-soluble and water-absorbing. Examples: ○ Hand Sanitizer Gel (classic example) ○ Benzoyl Peroxide Gel (acne treatment) ○ NSAID Gels (e.g., Voltaren gel for joint pain) ○ Testosterone Gel ○ Benzomycin Gel (acne treatment) ○ Clindamycin Phosphate Gel (acne treatment) Patient Acceptance and Therapeutic Properties: ○ Application: Upon applying a gel, the solvent evaporates, leaving behind a porous matrix film. This film allows for drug diffusion. ○ Drug Delivery: This porous matrix is highly efficient in releasing the drug, making gels advantageous for drug delivery. ○ Penetration Enhancement: Solvents like ethanol can further enhance drug penetration into the skin. ○ Potency: Due to efficient drug release and potential penetration enhancement, gels often exhibit greater potency compared to other dosage forms with the same drug concentration. Additional Notes: The source emphasizes that not all gelling polymers are polysaccharides. The example provided, hand sanitizer gel, uses a carbomer, which is not a polysaccharide. While the classic gel is clear, many gels, such as benzoyl peroxide gel, are not. The source also points out that gels are versatile and can be formulated to deliver various types of drugs. Collodions are liquid preparations of pyroxylin (a cotton derivative) dissolved in a solvent containing alcohol, ether, and acetone, with or without added drug. ○ Upon application, the solvent evaporates, leaving an occlusive, film-like residue of pyroxylin that can seal small wounds and protect surrounding tissue. ○ Example: Wart treatment. Castor oil can be added as a plasticizer to soften the pyroxylin and ensure it stays in place. Other Topical Dosage Forms Aerosols are blends of drug and propellant, functioning as delivery systems for solutions, suspensions, powders, and emulsions. ○ They allow for spray application without rubbing, making them useful for areas where rubbing would be irritating (e.g., sunburns). ○ Examples include: Antifungal spray powders. Sunburn products like Solarcaine. Plasters are a solid or semisolid adhesive mass spread on a backing material, intended for prolonged external application. ○ ○ They are similar to patches but can be cut to the shape of the area being treated. ○ Example: Salicylic acid plaster (e.g., Mediplast) used for plantar warts and calluses. Wet dressings are aqueous solutions applied as a dressing (e.g., gauze, linens) soaked in the solution. ○ ○ The solutions are typically astringents (stop bleeding or secretions by precipitating proteins) or antiseptic agents. ○ Benefits: Cleanse wounds of exudates. Help maintain drainage of infected wounds. Promote wound healing. Repeated application and removal can dry the skin and remove exudates and secretions. ○ Example: Aluminum acetate. Foams are gas-propelled liquids or solids administered as aerosols, similar to shaving cream in consistency. ○ ○ Benefits: Gentle and easy to spread without rubbing. Non-runny. ○ Drawback: Tends to break down quickly. Excipients in Topical Dosage Forms Antioxidants prevent rancidity in hydrocarbon-containing dosage forms. ○ Examples include: Butylated hydroxytoluene (BHT). Butylated hydroxyanisole (BHA). Vitamin E (alpha-tocopherol). Antimicrobial preservatives prevent microbial growth in water-containing dosage forms. ○ Examples include: Benzalkonium chloride. Parabens. Benzoic acid. Waxes increase stiffness and reduce tackiness in ointments and creams. ○ Examples include: Beeswax. Cetyl esters wax. Cetyl alcohol. Moisturizers hydrate the skin and are classified as: ○ Occlusive , which inhibit transepidermal water loss: Petrolatum is highly occlusive, reducing transepidermal water loss by about 99%. Aquaphor is also occlusive, though less so than petrolatum. Dimethicone and other silicones are non-oleaginous occlusive agents, often used in oil-free moisturizers. ○ Humectants , which are hygroscopic and attract water to hydrate the skin: They are often added to creams and lotions to enhance moisturizing capacity. They can work within the skin, augmenting the skin's hygroscopic capacity, or on the skin surface, absorbing atmospheric moisture (dependent on humidity). At high humidity, they draw water from the atmosphere into the skin, but in arid environments, they can draw water from the skin, leading to a drying effect. Examples include: Propylene glycol. Glycerin. Sorbitol. Urea. Alpha hydroxy acids (AHAs, e.g., lactic acid). These agents can also function as penetration enhancers by hydrating the skin. Co-solvents enhance drug solubility and prevent precipitation as the dosage form dries. ○ Examples include: Propylene glycol is commonly used in creams to prevent drug precipitation and enhance permeation. Glycerin is a good co-solvent because it does not evaporate easily. Ethanol evaporates quickly but can enhance penetration. Practical Considerations for Topical Medications Application: ○ A thin film is usually sufficient, with the exception of diaper rash pastes. ○ The fingertip unit is a method for estimating dosage, representing the amount dispensed from a tube reaching from the fingertip to the first crease of the index finger (approximately 0.5 grams). ○ The rule of hand estimates the amount of product needed for a thin film application to both sides of the hand (one hand area) as one fingertip unit. General advice for application: ○ Cleanse and dry the area to be treated. ○ Apply shortly after bathing or showering when the stratum corneum is hydrated. ○ Apply moisturizers within 3 minutes of bathing or showering. ○ Cleanse hands before and after application. ○ Use gentle rubbing motions to apply creams and ointments, apply lotions as drops and massage lightly, and rub gels gently and completely. Precautions: ○ Avoid drug transfer to sensitive areas, such as the eyes, by cleansing hands. ○ Exercise caution when applying to thin-skinned areas (e.g., face, back of hands), as the thinner stratum corneum allows for greater drug penetration. ○ Intertriginous areas (where skin touches skin) are naturally occlusive, potentially increasing drug potency, so use caution. ○ Avoid applying medicated products to large areas, especially in children, as it can lead to increased drug penetration and systemic absorption. 4.3 Vaginal Drug Delivery Vaginal drug delivery is typically self-administered. The drug is intended to distribute throughout the vaginal cavity. There are two main purposes of vaginal drug delivery: ○ Local effects: This is the most common purpose. Some examples of local effects include: Vaginal infections Lubrication Contraception ○ Systemic absorption: While the vagina is a good route for systemic absorption, there aren't many products designed for this purpose. However, there is ongoing research in this area. Dosage Forms for Vaginal Drug Delivery Liquids: These can include douches. Semisolids: This category encompasses: ○ Creams ○ Gels ○ Ointments ○ Foams ○ These dosage forms will be covered in detail in topical drug delivery lectures. Solids: This is one of the more common dosage forms, particularly suppositories. ○ Students will be making vaginal suppositories in the spring. ○ Other solid forms can include compressed tablets shaped like suppositories. ○ Other terms for vaginal suppositories include: Pessary (more common in Europe) Ovule Insert Films: ○ Similar to oral and buccal films, the drug is evenly distributed within a rapidly disintegrating polymer sheet. ○ The polymer is usually a cellulose derivative. Anatomy and Physiology of the Vagina Length: The vagina is approximately 6 to 10 centimeters long, extending from the cervix. Mucosa: This mucus membrane is vital as it's the primary site of drug absorption. ○ Like the mouth and rectum, it is coated with mucus, which keeps the epithelium moist. ○ It's composed of a stratified squamous epithelium, making it more similar to skin than the GI tract. This means the drug needs to pass through multiple cell layers for absorption. Rugae: These folds in the vaginal wall offer several advantages: ○ Increased surface area: This can enhance drug absorption if systemic effects are desired. ○ Product retention: The folds help keep the drug product in place. Microflora: The vagina has a natural microflora that's essential for a healthy mucosa. This microflora should not be disrupted by drug formulations. Epithelium: The stratified squamous epithelium of the vagina has several key features: ○ The outermost layer consists of flattened squamous cells, much like the skin. ○ The stratum corneum, like that of the skin, is made up of dead corneocytes and intercellular lipids. ○ Key difference from skin: The intercellular lipids in the vaginal stratum corneum do not form an impermeable layer. This makes the vaginal stratum corneum more permeable than the skin's, allowing for easier drug passage. Vaginal Fluid Limited amount: Similar to the rectum, the vagina has a limited amount of fluid. Complex composition: Vaginal fluid is a mixture of various components, including: ○ Cervical secretions ○ Transudates from between cells ○ Exfoliated cells ○ Leukocytes Low pH: The vaginal fluid is typically acidic due to the presence of lactobacilli. ○ These bacteria convert glycogen into lactic acid, contributing to the acidic environment. ○ The acidic pH has a bacteriostatic effect, which is crucial for a healthy vaginal environment. ○ Important for formulation: Manufacturers need to consider the acidic pH when designing vaginal drug products to avoid disrupting this natural defense mechanism. Enzymes: Vaginal fluid contains enzymes that may impact the stability of certain drugs. Formulators need to be aware of these enzymes and their potential interactions with the drug being administered. Systemic Absorption from the Vagina Limited surface area: While the rugae do increase surface area, the vagina has a relatively limited surface area compared to the GI tract, which can limit systemic absorption. No hepatic first-pass effect: Like buccal administration, drugs absorbed through the vagina bypass the hepatic portal system, avoiding first-pass metabolism in the liver. This makes it an advantageous route for drugs with a high first-pass effect. Variability: The vaginal mucosa can vary significantly between patients, affecting drug absorption. Factors influencing this variability include: ○ Amount and composition of vaginal fluid ○ Consistency of vaginal fluid ○ Menstrual cycle ○ Age (pH is generally higher in prepubescent girls, postmenopausal women, and during certain stages of the menstrual cycle) ○ Epithelial thickness (thinner after menopause) Good absorption for some drugs: Studies show that certain drugs, even large molecules that struggle to penetrate the skin, can be well-absorbed vaginally. ○ This is because the vagina lacks the continuous intercellular lipid barrier found in the skin. Limited systemic absorption for most current products: Several factors contribute to this: ○ The multi-layered stratified squamous epithelium, while more permeable than skin, still poses a barrier. ○ Short residence time of many vaginal products, limiting absorption time. ○ Significant interpatient variability in absorption due to differences in the vaginal mucosa. Considerations for Vaginal Drug Product Design Product pH: ○ Formulators should consider the natural acidic pH of the vagina and its importance in infection resistance. ○ Ideally, products should be formulated to match the vaginal pH. ○ However, pH adjustments might be necessary for drug solubility or stability. In such cases, a weak buffer should be used to allow vaginal fluids to readjust the pH after administration. Microbial presence: ○ Products must be free from any microorganisms, including yeast and molds, to prevent infections and disruption of the natural microflora. Residence time: ○ Products should remain in the vagina long enough for adequate drug distribution. ○ Factors affecting residence time include: Rheology (viscosity): More viscous products are less likely to leak out. Level of insertion: High insertion typically leads to better retention. Dosage form: Solid dosage forms should liquefy, disintegrate, or melt within minutes. Distribution: ○ Ideally, the product should spread evenly throughout the vaginal cavity. ○ Liquids and foams tend to spread better than disintegrating tablets or suppositories. Leakage: Products should be designed to minimize leakage. Administration aids: Most vaginal products come with applicators to facilitate high insertion. Examples of Vaginal Drug Products Inserts (suppositories): ○ While suppository formulations are usually simple, some, like IMVEXXY, can have complex formulations with multiple excipients. Creams: ○ Example: Tramizol vaginal cream, which contains benzoyl alcohol (likely as a preservative). Ointments: ○ Often have simple formulations. ○ Example: An ointment containing butylated hydroxyanisole (BHA) as an antioxidant. Suppositories with fatty acid base: A classic type of suppository. Gels: ○ Can be formulated with gelling agents like carbomer (also known as carbopol). ○ Bioadhesive gels, containing excipients like polycarbophil, can enhance residence time by adhering to the vaginal mucosa. Films: ○ Example: VCF contraceptive film, a fast-dissolving polymer film containing Nonoxynol-9. General Administration of Vaginal Suppositories The sources provide general advice on administering vaginal suppositories. Important: Always refer to the manufacturer's specific information for the particular product being used, as administration instructions can vary. The information on general administration will be covered in the exam. 4.4 Ophthalmic Drug Delivery Ophthalmic drug delivery primarily involves applying drugs to the eyes, with eye drops being the most common method. While designed for local effects, some drugs applied to the eye can enter systemic circulation. Indications for Topical Ophthalmic Products Topical ophthalmic products are commonly used for a range of conditions: Glaucoma: Treatment of increased intraocular pressure. Infections: Antibacterial, antiviral, and antifungal products for primarily external eye infections. Inflammatory Conditions: Corticosteroids and NSAIDs for external and internal inflammation. Dry Eye: Over-the-counter (OTC) and medicated eye drops. Alternative Delivery Methods Intraocular Injection: Directly into the eye for faster, higher, and more deeply distributed ocular drug levels, particularly to reach the retina. Subconjunctival Injection: Injection into the conjunctiva, similar to a subcutaneous injection. Topically applied drugs typically do not reach the retina in adequate concentrations. Anatomy and Physiology of the Eye Understanding the eye's structure is crucial for comprehending drug delivery. Eye Wall Layers The eye wall consists of three concentric layers: 1. Outermost Layer: Conjunctiva and sclera (white part of the eye). ○ The cornea, a transparent layer, is the primary site for drug penetration into the eyeball. 2. Middle Layer: Uveal tract, comprising the choroid, ciliary body, and iris. ○ The uveal tract is an important target for many ophthalmic drugs. 3. Innermost Layer: Retina (back of the eye). ○ Topically applied drugs typically struggle to reach the retina in sufficient concentrations. Humors of the Eye The inside of the eye is divided by the lens into two humors: ○ Aqueous Humor: Located in front of the lens. ○ Vitreous Humor: Located behind the lens. Segments of the Eye The eye is also divided into anterior and posterior segments: ○ Anterior Segment: In front of the lens, contains the aqueous humor. ○ Posterior Segment: Behind the lens, contains the vitreous humor. Chambers of the Aqueous Humor The iris divides the aqueous humor (in the anterior segment) into: ○ Anterior Chamber: In front of the iris. ○ Posterior Chamber: Behind the iris. The aqueous humor is continuously produced by the ciliary bodies and flows from the posterior chamber to the anterior chamber, exiting through the pupil and draining into systemic circulation via the canal of Schlemm. Surrounding Structures Conjunctiva: Thin, transparent, vascularized mucous membrane extending from the edge of the cornea across the sclera and the inner surface of the eyelids, forming conjunctival sacs. ○ Eye drops are typically instilled into these conjunctival sacs. Lacrimal Gland: Produces tears, which play a crucial role in removing foreign material. ○ Tears flow through the puncta (small openings) into the lacrimal canaliculi, then to the lacrimal sac, and finally drain into the nasal cavity via the lacrimal duct. Nasolacrimal Apparatus This drainage system removes tears: ○ Tears drain through the canaliculi, lacrimal sac, and into the nasal cavity via the lacrimal duct. ○ From the nasal cavity, tears are transported to the nasopharynx and eventually swallowed. Excessive tearing can overflow from the nasal cavity. Tear Drainage Mechanism Tear drainage relies on gravity and a pumping mechanism facilitated by blinking. ○ Blinking (about 15-20 times per minute) helps pump tears through the nasolacrimal apparatus into the nasal cavity. Target Areas for Topical Ophthalmics Topical ophthalmic drugs primarily target: Preocular Structures: Conjunctiva and eyelids, often for infections or inflammatory conditions. Corneal Tissues: For treating corneal issues such as infections or pain. ○ The cornea is highly sensitive due to numerous nerve endings. Tissues and Receptors in the Anterior Segment: Includes iris muscles, ciliary epithelium, and ciliary muscle. Challenges Reaching the Posterior Segment Reaching tissues in the posterior segment (e.g., the retina) with topically applied drugs is highly unlikely due to various barriers. Drug Absorption and Distribution Transcorneal Absorption The primary route for topical ophthalmics to reach intraocular tissues is transcorneal absorption (through the cornea). ○ While drug passage can occur through the sclera (white of the eye), absorption through the cornea dominates. Corneal Structure The cornea is a complex, optically transparent tissue covered by a tear film. Tear Film Layers The tear film comprises three crucial layers: 1. Mucoid Layer: Promotes adhesion of the aqueous tear fluid and keeps the cornea wettable. ○ The eyeball is a mucous membrane that needs to stay moist. 2. Tear Fluid: A solution of inorganic electrolytes, proteins (including enzymes and immunoglobulins), and glucose. 3. Outer Lipid Layer: Composed of wax and cholesterol esters that reduce tear fluid evaporation. Role of Tear Film in Drug Delivery The drug concentration in the tear film drives passive diffusion through the cornea. Passive diffusion is the dominant mechanism for corneal permeation. Corneal Layers The cornea has three main layers relevant to drug absorption: 1. Epithelium: Outermost layer, primarily lipophilic. 2. Stroma: Middle layer, primarily hydrophilic and composed of collagen fibers. 3. Endothelium: Innermost layer, a single cell layer. Corneal Epithelium as a Barrier The corneal epithelium, continuous with the conjunctiva but with varying thickness (5-6 layers at the center to 8-10 layers at the periphery), is the main barrier for drug passage through the cornea. Squamous cells with tight junctions on the surface, uniquely possessing microvilli that increase surface area and potentially aid absorption. Being primarily lipophilic, the epithelium favors the penetration of lipophilic drugs. The unionized form of a drug penetrates better than the ionized form. Enzymes present in the epithelium (esterases, peptidases, and proteases) can metabolize some drugs. Stroma and Endothelium The stroma, composed of collagen fibers and mostly water, acts as a hydrophilic barrier. However, lipophilic drugs that have passed the epithelium generally pass through the stroma without major issues. The endothelium, a single cell layer, is more permeable than the epithelium (about 200 times) and poses a lesser barrier to drug penetration. Factors Influencing Transcorneal Absorption Lipophilicity and Ionization: Drugs with a moderate octanol-water partition coefficient (balancing lipophilicity and hydrophilicity) and a favorable ionization state at physiological pH are best absorbed. Concentration Gradient: A higher drug concentration in the tear film results in faster penetration due to passive diffusion. Epithelial Integrity: Damaged epithelium enhances drug absorption. Time to Peak Concentration: It takes approximately 20-60 minutes for a topically applied drug to reach peak concentrations in the aqueous humor. Overall Efficiency: Typically, less than 5% of an instilled eye drop dose crosses the cornea. Drug Loss Before Corneal Penetration Several factors contribute to drug loss before it can reach the aqueous humor: Spillage: The lower conjunctival sac can only accommodate approximately 30 microliters. Any excess volume spills over. Blinking: Blinking increases spillage and promotes drug removal through the nasolacrimal apparatus. ○ Blinking reduces the residual volume to about 10 microliters. Nasolacrimal Drainage: Drugs forced through the puncta by blinking and gravity are removed via the nasolacrimal drainage system, eventually being swallowed. Multiple Drops: Administering multiple drops consecutively can lead to significant loss of the second drop. Drug Fate After Corneal Penetration Distribution and Elimination Drugs that cross the cornea primarily reach the aqueous humor in the anterior chamber. The drug concentration in the anterior chamber is significantly lower than the applied concentration due to previous losses. Reaching the posterior segment is generally insignificant, limiting the treatment of posterior segment conditions with topicals. Protein Binding and Metabolism Some lipophilic drugs can bind to melanin in the iris, which can slow drug effects or act as a depot for sustained release. Drugs may also bind to proteins in tears. Ophthalmic metabolism can occur, with enzymes in the aqueous humor metabolizing certain drugs. ○ Prodrugs are designed to be activated by enzymes in the eye. Examples include dipivalyl epinephrine (converted to epinephrine) and latanoprost (converted to prostaglandin F2α). Drugs primarily exit the eye by following the aqueous humor turnover, exiting through the canal of Schlemm or entering uveal blood vessels and systemic circulation. 4.5 Ophthalmic Drug Delivery Study Guide The main goal of ophthalmic products is to treat the eye, not to be absorbed systemically. However, systemic absorption can occur. The clinical significance of this absorption varies based on factors like drug potency and strength. There are several pathways for drugs to enter systemic circulation, including: ○ Nasolacrimal Drainage: This is a major pathway where drops entering the puncta drain through the nasolacrimal system into the nasal cavity. ○ Absorption in Nasal Structures: Once in the nasal cavity, absorption can occur in the nasal cavity itself, the nasopharynx, the lacrimal sac, and even the cheeks through mixing with saliva. ○ GI Tract Absorption: Drugs reaching the nasopharynx can be swallowed, leading to absorption in the gastrointestinal tract. ○ Conjunctival Absorption: The conjunctiva, with its rich blood supply, is more permeable than the cornea and offers a large surface area for drug absorption. ○ Absorption via Trabecular Meshwork: Drugs entering the eye can drain through the trabecular meshwork and subsequently enter the bloodstream. Over 50% of an instilled ophthalmic dose can be systemically absorbed. Except for drugs absorbed via the GI tract, the absorbed drug bypasses the hepatic first-pass effect. Key considerations in designing topical ophthalmic dosage forms include sterility, pH, osmolarity, and viscosity. Sterility is paramount for ophthalmic products , similar to injectable drugs, as contamination can lead to serious consequences, including blindness. ○ Pseudomonas aeruginosa is a particularly dangerous organism that can cause corneal ulcers, potentially leading to blindness. ○ Sterility testing is mandatory for commercially manufactured ophthalmics. Antimicrobial preservatives are required in all multi-dose eye drops to maintain sterility. Single-use eye drops do not require preservatives. ○ Benzalkonium Chloride (BAK) is the most common preservative due to its effectiveness, especially against Pseudomonas aeruginosa. BAK, being a surfactant, can enhance corneal penetration but also carries a risk of corneal epithelium damage at high concentrations. ○ Alternatives to BAK include: Polyquad (polyquaternium 1), which is considered less sensitizing and does not penetrate the cornea well. Thimerosal, a mercury-based preservative with a long history of use, but its use has declined due to hypersensitivity concerns. Oxidizing agents, like sodium perborate, are newer preservatives that act by oxidizing microbes. The ideal pH of an ophthalmic product should match that of the tear film (around 6.6-7.8). pH values outside this range can cause irritation, tearing, and blinking, leading to drug removal from the eye surface. ○ Buffers are included in formulations to adjust pH for stability, solubility, and patient comfort. Common buffers include acetate, phosphate, citrate, and borate. Similar to pH, maintaining isotonicity (osmolarity similar to tears, approximately 300 mOsm/kg) is crucial for patient comfort. Deviations from this range can irritate the eye, prompting tearing and blinking, which remove the drug. ○ While most products aim for isotonicity, some are intentionally hypertonic. For instance, 5% sodium chloride is used to treat corneal edema by drawing fluid away from the cornea. ○ Tonicity modifiers, such as sodium chloride, mannitol, and dextrose, are added to adjust osmolarity. Viscosity plays a role in ophthalmic formulations by influencing residence time and drug diffusion. ○ Increased viscosity can prolong contact time and reduce drainage rate, potentially enhancing bioavailability. However, excessive viscosity can irritate the eye, hinder drug diffusion, and cause blurred vision. ○ Common viscosity modifiers include glycerin, cellulose derivatives, polyvinyl alcohol, polyethylene glycols (PEGs), and carbomers. ○ Cellulose derivatives and polyvinyl alcohol are frequently used in lubricating eye drops or artificial tears. ○ High viscosity agents can cause transient blurring and crust formation upon drying. Liquid ophthalmics, primarily solutions, are the most common type. Other forms include suspensions and emulsions. ○ Solution formulations involve dissolving the drug and excipients, followed by sterilization. Sterilization methods include heat or filtration through a 0.2 micron membrane filter. ○ Suspensions contain finely micronized drug particles (typically less than 10 microns) to minimize irritation and promote rapid dissolution. They are used for poorly soluble drugs or to enhance drug stability. Suspensions cannot be filter sterilized as this would remove the drug particles. Instead, sterile drug crystals are aseptically mixed with a sterile vehicle. Suspensions, though not providing a depot effect, mix less rapidly with tears and can reside in the conjunctival sac, allowing for prolonged drug release. ○ Emulsions are less common and are employed for lipophilic drugs. The drug is dissolved in the oil phase, and surfactants are used for emulsification and stabilization. Common excipients in ophthalmic products: ○ Antioxidants: Sodium metabisulfite and its related sulfites are frequently used. Consider patient sensitivity to sulfites. ○ Chelating Agents: EDTA sodium is commonly included, serving dual roles: limiting oxidation by chelating metal ions and enhancing the activity of BAK against Pseudomonas aeruginosa. ○ Surfactants: Polysorbate 80 and tyloxapol are examples. They function as wetting agents in suspensions, emulsifiers in emulsions, and spreading agents to enhance drop coverage on the eye surface. Most liquid ophthalmics are packaged in dropper bottles called droppers. These are typically sterilized and come in various standard volumes. Semisolid ophthalmics include ointments and gels. ○ Ointments, often petrolatum-based, provide prolonged contact time due to their slow clearance rate (0.5% per minute). Mineral oil is often added to reduce the viscosity of petrolatum. Sterilization of the ointment base can be achieved through heat and sterile filtration. Ointments are frequently used post-surgery, for instance, antibiotic ointments like bacitracin to prevent infection. The major disadvantage is blurred vision, which can last longer than with viscous drops. ○ Gels, formulated using gelling agents like carbomers and cellulose derivatives, also offer prolonged contact time. Examples include products like Timoptic-XE, a gel-forming solution that increases contact time and allows for once-daily dosing. Administration of Ophthalmic Products: ○ General guidelines for eye drop administration: Thoroughly wash hands. Shake suspensions well before use. Tilt head back and create a pouch by pulling down the lower eyelid. Instill one drop into the conjunctival sac. Gently close the eye and keep it closed for 30 seconds. Avoid blinking. Applying gentle pressure to the inner corner of the eye for 30 seconds can minimize nasolacrimal drainage and systemic absorption, taste, and drainage. Avoid rubbing, wiping, or squeezing the eye. Replace the cap without touching the dropper tip. ○ Additional tips: Wait at least 5 minutes before applying another drop, whether of the same or a different medication. If using fast-acting and long-acting formulations together, apply the fast-acting one first, followed by the long-acting one after at least 5 minutes. Apply eye drops at least 10 minutes before eye ointments. Unless directed otherwise, remove contact lenses before instilling eye drops. Store eye drops according to product instructions, as some may require refrigeration. Monitor for signs of contamination and discard multi-dose products within 30 days of opening. ○ Instructions for eye ointment administration: Wash hands thoroughly. Hold the tube securely and tilt head back. Pull down the lower eyelid to create a pouch and gently squeeze a ribbon of ointment into the conjunctival sac. Close the eye for 1-2 minutes and roll the eyeball in all directions to distribute the ointment. Wipe away excess ointment and replace the cap without touching the tip. Warn the patient about potential blurred vision. 3.6 Intranasal Drug Delivery There are two main respiratory drug delivery approaches: nasal cavity and lungs (pulmonary drug delivery). The focus of this study guide is the nasal cavity, a complex and promising route for drug administration. Products delivered through the nasal cavity can be for local or systemic purposes. ○ Examples of local delivery: Nasal decongestants. Glucocorticoids (e.g., Flonase). ○ Examples of systemic delivery: Vitamin B12. Pain medications. Anatomy and Physiology of the Nose The two main functions of the nose are olfaction (smelling) and conditioning inspired air. The most important anatomical structures for intranasal drug delivery are the turbinates, located within the nasal cavity. Regions of the Nasal Cavity The nasal cavity has three regions: vestibular, respiratory, and olfactory. Vestibular Region The vestibular region consists of the nasal vestibule and the valve. Its main function is to filter and baffle air. The vestibular region is lined with long hairs and pseudostratified epithelia (skin-like). The nasal valve connects the vestibule to the nasal cavity and is a fairly narrow passageway. Respiratory Region The respiratory region is composed of three turbinates: inferior, middle, and superior. The inferior turbinate communicates with the nasal lacrimal ducts. The middle turbinate communicates with several sinuses. The superior turbinate has less physiological significance compared to the other two. Functions of the respiratory region: ○ Direct, heat, and humidify incoming air before it reaches the lungs. ○ Increase surface area , which can enhance aerosol deposition and drug absorption. ○ Promote turbulence , which can increase particle impaction and protect the lungs from unwanted substances. The respiratory region is the major site for drug absorption and action. Olfactory Region The olfactory region is located above the respiratory region, beneath the brain. Characteristics of the olfactory region: ○ Very thin layer and barrier between it and the brain. ○ Roof of the nasal cavity. ○ Small surface area. ○ Poorly ventilated , resulting in less spray reaching this area compared to the respiratory region. Due to its proximity to the brain, the olfactory region has the potential for drug delivery to the brain. Nasal Mucosa Respiratory Region Epithelium The anterior one-third of the respiratory region contains various types of epithelia: ○ Squamous. ○ Transitional. ○ Pseudostratified columnar. The posterior two-thirds of the respiratory region is composed of ciliated pseudostratified columnar epithelia. Goblet cells are present and produce mucus. Mucociliary Clearance Cilia play a crucial role in mucociliary clearance, a major mechanism for removing particles and drugs from the nasal cavity. Cilia sweep mucus and trapped substances backward, eventually leading to swallowing. Undissolved drugs are particularly susceptible to mucociliary clearance. The presence and fluidity of mucus are vital for ciliary function. Increased mucus viscosity, as seen in conditions like the common cold, can slow down ciliary movement and clearance. Mucociliary clearance is a significant physiological factor affecting drug absorption. Other Components of the Nasal Mucosa Mucus has various functions, including: ○ Protecting and hydrating the epithelium. ○ Trapping and transporting inhaled substances. Mucus is continuously secreted and swallowed. Average mucus pH is around 6.3 (slightly acidic). Enzymes are present in the nasal mucosa and contribute to mucociliary clearance. Transporters , such as efflux systems like p-glycoprotein, are expressed in the nasal epithelium and can transport substances out. Blood Supply The nasal epithelium is highly vascularized. Capillaries are fenestrated (have holes), allowing for the passage of large molecules. Drugs that pass through the epithelium can easily enter the bloodstream. There is no hepatic portal system , meaning no hepatic first-pass effect for drugs absorbed through the nasal cavity. Advantages of Intranasal Drug Delivery Avoidance of GI acid and enzymatic degradation , making it suitable for drugs that are unstable in the GI tract. No hepatic first-pass effect. Potential for high rate and extent of absorption due to: ○ Good vasculature. ○ Relatively permeable epithelium. ○ Fenestrated capillaries. Absorption of relatively large molecules , including peptides, which cannot easily penetrate other epithelia. Alternative route for vaccinations due to the presence of nasal-associated lymphoid tissue (NAALT). ○ Immunization in the nasal cavity can provide immunity to other mucous membranes. Disadvantages of Intranasal Drug Delivery Increased intranasal metabolism is possible if the drug is a substrate for enzymes present in the nasal mucosa. Complications can arise from pre-existing nasal conditions , such as: ○ Rhinitis. ○ Hay fever. ○ Asthma. ○ Nasal polyps. These conditions can affect drug deposition, absorption, and clearance. Environmental factors , such as humidity and temperature, can influence drug delivery: ○ Arid environments can dry up secretions. Medications , particularly anticholinergic drugs and vasoconstrictors, can affect nasal secretions and absorption: ○ Anticholinergic drugs can dry secretions. ○ Vasoconstrictors like Afrin can reduce systemic bioavailability by constricting blood vessels. Considerations for Product Design pH Solutions with a pH range of 4 to 8 minimize damage to the mucociliary apparatus. Ciliary beat frequency is best maintained at a pH of 7 to 10. Formulating at a lower pH (e.g., 5) can potentially increase residence time by slowing mucociliary clearance, but it's essential to consider buffer capacity. Osmolarity Isotonic fluids are preferred to minimize irritation , but a wide range is acceptable for nasal sprays. Sprays have a less dramatic effect on overall osmolarity compared to drops. Viscosity High viscosity can slow mucociliary clearance , increasing residence time. However, excessive viscosity can hinder drug release. Viscosity-enhancing excipients are often used, especially for suspensions. Preservation Antimicrobial preservatives are typically necessary to prevent microbial growth in repeatedly used nasal drops and sprays. Benzalkonium chloride is a commonly used preservative, though there have been concerns about its potential toxicity. Drug Deposition Sprays are the dominant type of intranasal product and are mainly deposited in the anterior region of the nasal cavity. Drops generally provide greater coverage throughout the nasal cavity but are cleared more rapidly. Sprays Most nasal sprays are either metered dose or single-dose mechanical sprays of aqueous formulations. Metered dose sprays deliver a predetermined, accurate volume of drug formulation using metering chambers. Particle size is crucial : ○ Large particles (8-20 microns) are necessary to prevent bypassing the nasal cavity. Spray volume is determined by the metering chamber, typically around 100 microliters. Bottle design facilitates delivery through the nasal valve. Interaction with saliva can lead to taste perception , which can be minimized with proper technique (tilting the head forward and down). Priming Many nasal sprays require priming, which involves spraying into the air or a tissue several times to fill the metering chamber. Priming ensures an accurate dose by preventing partial filling of the chamber. Single-use medications often do not require priming. Product Examples Diazalasine (Afrin) : a nasal decongestant that is bitter-tasting. Corticosteroids : often formulated as suspensions with cellulose derivatives and surfactants. Narcan : a single-use opioid overdose treatment that does not require priming. Migraine relief medications : often single-dose and do not require priming. Nascobal (cyanocobalamin) : a systemic medication for vitamin B12 deficiency, typically in single-day, 8-spray bottles without preservatives. Flumist : a single-use vaccine without preservatives. Administration General steps for administering nasal sprays are available on websites like the safe medication site. It's essential to follow specific recommendations for each product. 3.7 Reasons for Delivering Drugs to the Lungs Local Effect: The main reason for pulmonary drug delivery is to achieve a localized effect in the lungs. This is particularly relevant for conditions like asthma and COPD, where medications act directly on the airways to alleviate symptoms. Examples include: ○ Asthma medications: Beta-agonists (bronchodilators), glucocorticoids (anti-inflammatories), antimuscarinics (reduce secretions), and mast cell stabilizers. ○ COPD medications: Mucolytics like N-acetylcysteine and deoxyribonuclease to reduce mucus viscosity, particularly in cystic fibrosis. ○ Neonatal Respiratory Distress Syndrome: Treatment with exogenous pulmonary surfactant. ○ Infections: Medications like pentamidine for Pneumocystis jirovecii pneumonia and ribavirin for RSV. Systemic Effect: The lungs offer a promising route for systemic drug delivery due to their large surface area and the absence of hepatic first-pass metabolism. This means that drugs absorbed through the lungs bypass the liver, leading to higher bioavailability. ○ Examples of medications delivered systemically via the lungs include: Afrezza: A dry powder inhaler (DPI) of recombinant human insulin for diabetes. Adesu: A DPI of loxapine for schizophrenia. INBRIJA: A DPI of levodopa for Parkinson’s disease. Methods of Pulmonary Drug Delivery Intratracheal Instillation: This method involves directly administering the drug solution into the trachea for distribution to the lungs. It is primarily used for delivering pulmonary surfactant to neonates with respiratory distress syndrome. This method requires specific patient positioning to ensure proper drug distribution within the lungs. Aerosol Inhalation: This is the most common method, involving inhalation of aerosolized drug particles through the mouth for deep penetration into the lungs. This method is the primary focus of the source material. Aerosol inhalation can also be administered via a tracheostomy, where the device is attached to the trachea, directly delivering the drug to the lungs. Types of Oral Aerosols Propellant-driven: The most dominant type historically, these are the classic “aerosol” inhalers. They rely on a pressurized propellant to deliver the drug in a fine mist. Mechanical energy-driven: A newer type of inhaler that uses mechanical energy to generate the aerosol. Dry powder inhalers (DPIs): DPIs deliver the drug in a dry powder form, requiring the patient to inhale with sufficient force to disperse the powder into the lungs. These became prominent due to the phasing out of CFC propellants in metered dose inhalers. Nebulizers: Nebulizers use air or ultrasonic waves to create a cloud of drug particles that is inhaled by the patient. Anatomy and Physiology of the Respiratory Tract Tracheobronchial Tree: The lungs are comprised of a branching network of airways called the tracheobronchial tree. This tree starts with the trachea, which branches into progressively smaller airways: the bronchi, bronchioles, and finally, the alveoli. Alveoli: These tiny air sacs are the primary site of gas exchange in the lungs. The alveoli have a large surface area, which is advantageous for systemic drug absorption. Pulmonary Epithelium: The cells lining the airways are called the pulmonary epithelium. The epithelium in the upper airways and tracheobronchial tree is ciliated, meaning it has tiny hair-like structures called cilia. The cilia move in a coordinated fashion to propel mucus upwards, a process known as the mucociliary escalator. Role of Mucus in the Lungs Protection: The mucus in the lungs, similar to the nasal cavity, serves as a protective barrier. It traps foreign particles like dust, bacteria, and drug crystals, preventing them from reaching the delicate lung tissue. Hydration: Mucus also helps to keep the lung lining moist, preventing dehydration of the epithelial cells. Mucociliary Escalator: The mucus layer is constantly moved upwards by the cilia, carrying trapped particles towards the pharynx, where they are swallowed. This mechanism, known as the mucociliary escalator, is crucial for clearing the lungs of debris and foreign substances. Alveolar Macrophages Defense Mechanism: The alveoli contain specialized immune cells called alveolar macrophages, which act as scavengers. They engulf and remove foreign particles that reach the alveoli, including bacteria, dust, and undissolved drug crystals. Particle Removal: Macrophages contribute to particle removal by migrating towards the mucociliary escalator, carrying engulfed particles upwards to be eliminated from the lungs. Disposition of Aerosols in the Lungs Aerosol Cloud: All pulmonary inhalation devices generate a respirable cloud of particles, either solid drug crystals or droplets containing the drug. Deposition Sites: The location where the aerosol particles deposit in the lungs depends on several factors, including particle size, velocity, and the patient's breathing pattern. Gamma Scintigraphy: This imaging technique uses radioactive markers to visualize aerosol deposition in the lungs, illustrating the distribution of inhaled particles. A typical image will show significant deposition in the mouth, followed by the lungs, and then the stomach (due to swallowing). Fate of Deposited Drug Oropharynx (Mouth): Drug deposited in the mouth can be absorbed locally or swallowed, entering the gastrointestinal (GI) tract. Once in the GI tract, the drug is subject to intestinal and hepatic first-pass metabolism, potentially reducing its systemic availability. Tracheobronchial Region: Drug deposited in the mucus lining of the airways can be cleared by the mucociliary escalator, permeate the epithelium (mainly via passive diffusion) and be systemically absorbed, or be engulfed by macrophages. Alveoli: Drug reaching the alveoli can be absorbed systemically (bypassing hepatic first-pass metabolism), engulfed by macrophages, or exhaled. Mechanisms of Aerosol Deposition Inertial Impaction: Occurs when larger or faster-moving particles cannot follow the curves of the airways and collide with the airway walls, typically in the upper airways or mouth. Sedimentation: As airflow slows down deeper in the lungs, particles settle out of the airstream due to gravity. Diffusion: The smallest particles (< 1 micron) move randomly in the airstream and deposit on airway surfaces through diffusion. Aerosol-Related Factors Affecting Particle Deposition Particle Size: The size of aerosol particles, measured as aerodynamic diameter, is a crucial factor influencing deposition. Smaller particles generally penetrate deeper into the lungs. ○ Particles > 10 microns deposit in the oropharynx (mouth and throat). ○ Particles 5-10 microns deposit in the upper tracheobronchial tree. ○ Particles 1-5 microns are ideal for deep lung penetration, reaching the bronchioles and alveoli. ○ Submicron particles (< 1 micron) can reach the alveoli but are prone to exhalation. Particle Velocity: Higher particle velocity increases the likelihood of inertial impaction in the upper airways. Patient-Related Factors Affecting Deposition Breathing Pattern: ○ Rapid, shallow breathing: Favors deposition in the upper tracheobronchial tree. ○ Slow, deep breathing: Promotes deeper penetration into the lungs. Breath-Holding: Holding the breath after inhalation allows time for sedimentation and diffusion, enhancing deposition and minimizing exhalation of particles. Disease State: Lung diseases like asthma, COPD, and emphysema can alter airway caliber and airflow patterns, impacting particle deposition. ○ Narrowed airways (e.g., in asthma) and altered lung architecture (e.g., in emphysema) can lead to increased turbulence and impaction. Efficiency of Pulmonary Drug Delivery Low Efficiency: Pulmonary drug delivery is generally characterized by low efficiency, with a significant portion of the inhaled dose ending up in the GI tract (estimated at 80-95% but varies depending on the product). Factors Contributing to Low Efficiency: ○ Suboptimal aerosol generation: Particles outside the ideal size range, leading to impaction in the mouth and throat. ○ Fast release velocity: Favors impaction in the upper airways. ○ Suboptimal inhalation technique: Rapid inhalation increases the likelihood of impaction. Types of Pulmonary Delivery Devices Metered Dose Inhalers (MDIs): ○ Pressurized canisters: Contain drug, liquefied propellant, and minimal excipients. ○ Metering valve: Delivers a precise dose upon actuation. ○ Actuator (mouthpiece): Depresses the valve stem and directs the aerosol into the mouth. ○ Propellants: Typically hydrocarbon-based (e.g., HFA 134a). Act as the power source for aerosol generation and the vehicle for the drug. Properties like boiling point, vapor pressure, density, stability, and solvent power are crucial for device design and performance. ○ Aerosol Release Mechanism: High pressure inside the canister forces the liquid out upon actuation. Rapid evaporation of the propellant creates a fine mist of droplets containing the drug. ○ Formulations: Solutions: Drug dissolved in the propellant, may require co-solvents (e.g., ethanol) to enhance solubility. Suspensions: Drug particles dispersed in the propellant, common due to the limited solubility of many drugs in hydrocarbon propellants. Disadvantages: Physical instability (settling, aggregation). Excipients: Surfactants (e.g., oleic acid, soy lecithin) for dispersion, lubrication, and prevention of particle adhesion; co-solvents may be used to solubilize excipients. Dry Powder Inhalers (DPIs): ○ Rose in popularity as an alternative to MDIs with the phasing out of CFC propellants. Nebulizers: ○ Produce a cloud of drug particles via air jet or ultrasonic waves. 4.8 Metered Dose Inhaler (MDI) Study Guide Loss of prime ○ Loss of prime refers to propellant and drug loss from the metering valve of an MDI. ○ The propellant can leak from the metering chamber back into the canister over time or due to the shock of being dropped. ○ Loss of prime affects the dose delivered to the patient. ○ Priming an inhaler involves spraying 2 to 4 sprays into the air to refill the chamber. ○ Priming is typically recommended: For new inhalers. After a long period of nonuse. After dropping the inhaler. After cleaning the mouthpiece. ○ Always follow the manufacturer's specific advice for priming. Loss of dose ○ Loss of dose occurs when the aerosol dose is less than the labeled claim. ○ Causes of loss of dose: Loss of prime Heterogeneous system (suspension) ○ In inhaler suspensions: The propellant is lipophilic and typically denser than the drug. The drug typically rises to the top (creaming). ○ Shaking the inhaler immediately before use is crucial to ensure a uniform dose. Clogging ○ The actuator or valve stem can become clogged due to residue from the suspension. ○ Surfactants in the formulation can help minimize clogging. Inhalation aids (spacers) ○ Spacers help overcome challenges associated with MDIs: Difficulty coordinating actuation with inhalation. Fast aerosol velocity leading to deposition in the back of the throat. Poor lung targeting. ○ Spacers provide space between the inhaler and the patient's mouth. ○ Using a spacer: The inhaler is attached to the spacer. Actuation occurs into the spacer. The patient immediately inhales slowly and deeply. ○ How spacers work: Allow time and space for evaporation, reducing particle size. Reduce aerosol velocity, leading to less impaction. Make it easier to coordinate actuation with inhalation. Reduce oropharyngeal deposition. Improve lung targeting. ○ Effects of using a spacer: Create a more respirable cloud. Reduce particle size due to propellant evaporation. Reduce aerosol velocity. Make it easier for the patient to use the MDI. Reduce oropharyngeal deposition. Improve lung targeting. ○ Drawbacks of using a spacer: Significant loss of aerosol product within the device. ○ Features of spacers: Valves (inspiratory and expiratory) Flow indicator sound Antistatic coating ○ Types of spacers: Mouthpiece Mask (typically for children) Breath-actuated pressurized metered dose inhaler (BAI) ○ BAIs automatically trigger actuation when the patient inhales. ○ Benefits of BAIs: Eliminate the need for coordinating actuation. Actuated with a relatively low inspiratory rate. Typically, do not require spacers. ○ Example: Redhaler Does not require priming. Respimat ○ Key difference: Respimat uses an aqueous liquid formulation and is propellant-free. ○ It is a mechanical energy-driven, multidose liquid MDI. ○ The drug solution is contained in a cartridge that is inserted into the Respimat device. ○ Preservative (Benzalkonium Chloride) is typically included due to the aqueous formulation and multidose nature. ○ Aerosol generation: The liquid formulation is pushed through nozzles by a spring mechanism. ○ Characteristics of the aerosol: Soft and slower compared to pressurized MDIs. Does not require a spacer. Dry Powder Inhaler (DPI) Study Guide DPIs dispense dry powder into the lungs using the patient's inhaled breath. The patient's inspiratory effort provides the force for powder flow. DPI formulations typically consist of: ○ Micronized drug (1-5 microns) ○ Carrier powder Challenges with DPIs: ○ Cohesion and adhesion of micronized drug particles ○ Particles sticking to each other or the device Carrier powders: ○ Larger particles that carry the smaller drug particles. ○ Help separate drug particles to prevent sticking. ○ Improve powder flow and drug particle dispersibility. ○ Examples: lactose, glucose (lactose is more common). How DPIs work: ○ Powder mixture (carrier + drug) is inhaled through a screen. ○ Larger carrier particles impact the throat and are swallowed. ○ Respirable drug particles reach the lungs. Types of DPIs: ○ Unit dose DPIs: Drug formulation is contained in a prefilled hard gelatin capsule. The capsule is loaded into the device and punctured before inhalation. Examples: HandiHaler (Spiriva) INBRIJA Afrezza (insulin) ○ Multiple unit dose DPIs: Several unit doses are loaded into the device. Examples: Diskus Drug + lactose in blisters Wixela Inhub Ellipta Can contain two drugs in separate blisters for simultaneous delivery. Example: Breo Ellipta (fluticasone and vilanterol). ○ Multiple dose DPIs: Less common today. Drug is stored in a bulk reservoir. Doses are metered into an inhalation chamber. Example: Flexhaler More sensitive to humidity than individually packaged doses. Important factors for DPI use: ○ Inspiratory flow rate: The patient's inhalation force is the driving force for powder delivery. Adequate inspiratory flow is necessary for optimal drug delivery. DPIs may not be suitable for patients with weak inspiratory flow. ○ Humidity: Moisture absorption can: Increase particle size (sticking). Decrease deagglomeration. Reduce drug respirability. Patients should avoid breathing into DPIs to prevent moisture contamination. Nebulizers Study Guide Nebulizers deliver medication as an aerosolized mist. Administration: ○ Through a mouthpiece, ventilation mask, or tracheostomy. ○ During normal breathing. Treatment time: ○ Typically 7-10 minutes but can vary depending on the product. Types of nebulizers: ○ AirJet nebulizers: Use compressed air forced through an orifice to create negative pressure. The negative pressure draws liquid from the product, creating an aerosol. Smaller particles are inhaled, while larger particles impact a baffle and drop back into the fluid. Types of AirJet nebulizers: Conventional (e.g., t-NEB) Continuously produce aerosol. Aerosol can be lost during exhalation. Breath-enhanced (e.g., PARI) Produce aerosol based on the patient's inspiratory flow. Minimize aerosol waste. ○ Ultrasonic nebulizers: Use piezoelectric crystals that vibrate when electrically excited. The vibrations create geysers at the liquid surface, producing an aerosol. Advantages: Highly portable General considerations for nebulizers: ○ Aerosol particle size can vary between nebulizers, affecting lung deposition. ○ Nebulizer solutions are typically FDA-approved for use with specific nebulizers. ○ Efficiency of aerosol delivery is low (1-10% reaches the lungs). ○ Dead volume remains in the nebulizer cup. ○ Significant aerosol loss can occur during exhalation. ○ Protein drugs may denature due to shear forces. ○ Variability in particle size exists within and between brands. ○ Switching between nebulizer brands or types is often not recommended. Nebulizer formulations: ○ Aqueous ○ May contain co-solvents to improve drug solubility. ○ Ideally isotonic but not critical due to the large surface area of the lungs. ○ pH should be above 5 to avoid bronchospasm. ○ Limited buffer capacity to allow lung fluids to take over. ○ Additives must be chosen carefully. ○ Preservatives may be included for multi-use products. ○ Sterile Patient populations for oral inhalation devices: ○ Pressurized MDIs: 7 years and older without a spacer 3 years and older with a spacer ○ DPIs: 3 or 4 years and older (capable of forceful inhalation) ○ Nebulizers: Suitable for all ages (coordination is not required)