Advanced Drug Delivery Systems PDF
Document Details
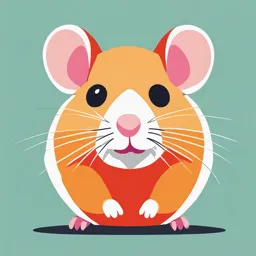
Uploaded by EncouragingTurkey
Tags
Summary
This document describes advanced drug delivery systems, exploring temporal and spatial control methods. It discusses the benefits of these systems for patient safety, efficacy, and convenience, and types of drug delivery systems.
Full Transcript
3.9 Advanced Drug Delivery Advanced drug delivery , also known as therapeutic systems, aims to optimize drug delivery. There are two main approaches to optimizing drug delivery: ○ Temporal control : Controlling the timing of drug delivery. For example, this can be achieve...
3.9 Advanced Drug Delivery Advanced drug delivery , also known as therapeutic systems, aims to optimize drug delivery. There are two main approaches to optimizing drug delivery: ○ Temporal control : Controlling the timing of drug delivery. For example, this can be achieved by using extended release, controlled release, or pulsatile release products. ○ Spatial control : Targeting the drug to the site where it is needed most. Why Advanced Drug Delivery? Pharmaceutical companies develop advanced drug delivery systems for two main reasons: ○ Benefits to the patient Safety and efficacy: Advanced drug delivery systems can help to improve patient safety and efficacy by providing a steady blood level of the drug, rather than the spikes that can occur with immediate-release products. Convenience and compliance: Advanced drug delivery systems can also improve patient convenience and compliance by reducing the frequency of dosing. For example, some advanced drug delivery systems allow for once-a-day, once-a-month, or even once-every-five-years dosing! ○ Repatenting drugs When a patent on a drug expires, pharmaceutical companies may develop extended-release versions of the drug in order to extend their patent protection. This can allow them to continue to generate revenue from the drug. The Ideal Drug Delivery System The ideal drug delivery system would have the following characteristics: ○ It would deliver the drug at the perfect time over the entire treatment period. ○ It would deliver the drug only to the site of action. In reality, it is difficult to achieve these ideals. However, advanced drug delivery systems can approach these. ideals Types of Advanced Drug Delivery Systems There are many different types of advanced drug delivery systems. Some of these systems are mechanical, such as pumps. However, most advanced drug delivery systems are based on polymers. These systems can be used to deliver drugs via most routes of administration, including IV, oral, transdermal, subdermal, intrauterine, and ophthalmic. Carrier Particles Carrier particles , such as liposomes and microspheres, can also be used in advanced drug delivery systems. These particles can be used to: ○ Control drug release ○ Target the drug to a specific site Microspheres were briefly introduced when discussing Afrezza, the inhaled insulin therapy, in the context of pulmonary drug delivery. ○ There are a number of microsphere products on the market. ○ Another way to target drugs is to attach them to antibodies. ○ Antibodies can bind to specific targets in the body. ○ By attaching a drug to an antibody, it is possible to target the drug to the specific cells that express the target. Other proteins , such as albumin, can also be used to target drugs. ○ One product on the market uses albumin to target a drug to cells that naturally take up albumin. ○ In general, the particulate approach is mainly used to target drugs. However, microspheres can also be used to prolong drug release. Polymers in Advanced Drug Delivery As mentioned earlier, polymers are a critical component of most advanced drug delivery systems. Polymers are large molecules that are made up of repeating units. They can be natural or synthetic. In advanced drug delivery systems, polymers are used to: ○ Control the rate of drug release ○ Target the drug to a specific site Polymers can be thought of as a meshwork or sponge with pores. ○ The drug is embedded within these pores. ○ The drug must travel through the pores in order to be released from the system. ○ The rate of drug release is controlled by the size of the pores and the thickness of the polymer. Polymers are currently used in most routes of administration. There are many different types of polymers, each with its own unique properties. ○ For example, some polymers have different porosities (number of pores). ○ Some polymers have different pore sizes. ○ Some polymers can swell when they come into contact with body fluids. This swelling can cause the pores to increase in size, which can lead to faster drug release. ○ Some polymers are bioerodible, meaning that they can be broken down by the body. This allows for the development of implants that do not need to be removed. ○ Mass Transfer Across Polymers Mass transfer is the movement of molecules from one place to another. In the context of advanced drug delivery, mass transfer refers to the movement of drug molecules through a polymer. The rate of drug release from a polymer system is determined by the rate of mass transfer across the polymer. The rate of mass transfer across a polymer is described by the following equation: Flux = Area * Permeability * Concentration Gradient Where: ○ Flux: The rate of transport through the polymer. The faster the flux, the faster the drug release. ○ Area: The area of the polymer that is in contact with the environment. The larger the area, the faster the drug release. ○ Permeability: A measure of how easily the drug can pass through the polymer. Permeability is a function of the drug-polymer interaction. The more permeable the polymer, the faster the drug release. ○ Concentration Gradient: The difference in drug concentration between the inside and outside of the polymer. The greater the concentration gradient, the faster the drug release. ○ Patterns of Drug Release There are three main mathematical models that are used to describe drug release from polymer systems: ○ Zero Order Release: The release rate is constant over time. This means that the drug is released at a steady rate until the device is empty. This is considered true controlled release. Zero order is achieved when the concentration gradient is constant, which can be achieved with a reservoir system. Zero-order release plots as a flat line on a graph of release rate vs time. ○ First Order Release: The release rate is proportional to the amount of drug remaining in the device. This means that the release rate decreases over time. This type of release is generally not important for controlled release applications. First-order release plots as a curve that decreases over time on a graph of release rate vs time. ○ Square Root of Time Release: The release rate is linear with the square root of time. This means that the release rate decreases over time, but at a slower rate than first-order release. This type of release was developed by a pioneer in the field of controlled release named Higuchi. The Higuchi equation describes this type of release. Square root of time release occurs in matrix systems. Square root of time release plots as a curve that decreases over time on a graph of release rate vs. time, but is flatter than the first-order release curve. Types of Polymer Systems Used for Controlled Release Diffusion Devices: In these systems, diffusion through the polymer determines the rate of drug release. Diffusion devices can be either reservoir systems or matrix systems. ○ ○ Reservoir Systems: In these systems, the drug is contained in a saturated solution that is surrounded by a polymer membrane. The drug diffuses through the membrane and into the surrounding environment. A key aspect of reservoir systems is that the drug concentration within the reservoir remains constant. This is because as drug diffuses out of the reservoir, more drug dissolves from the crystals that are present in the saturated solution. Reservoir systems typically exhibit zero-order release kinetics. This is because the concentration gradient across the membrane remains constant over time. Examples of reservoir systems include: Patches IUDs Implantable rods Oral products ○ ○ Matrix Systems: In these systems, the drug is uniformly dispersed throughout a polymer matrix. The drug diffuses out of the matrix and into the surrounding environment. Matrix systems typically exhibit square root of time release kinetics. This is because the path length that the drug must diffuse through the matrix increases over time as the drug is released from the outer layers of the matrix. As drug is released from the outer parts of the matrix, an empty matrix is formed. This empty matrix is referred to as a "ghost matrix." The ghost matrix expands over time as more drug is released. Examples of matrix systems include: Patches Implants Oral products Solvent Controlled Systems ○ Osmotic Systems: In these systems, osmotic pressure is used to control the rate of drug release. Osmotic systems typically consist of a drug compartment that is surrounded by a semipermeable membrane. The membrane is permeable to water, but not to the drug. When the system is placed in an aqueous environment, water is drawn into the drug compartment by osmosis. This creates pressure inside the compartment, which forces the drug out through a small opening, such as a laser-drilled hole. Osmotic systems typically exhibit near-zero-order release kinetics. This is because the osmotic pressure inside the compartment remains relatively constant over time. Examples of osmotic systems include: Implants Oral products ○ Swelling Systems: In these systems, the drug is dispersed in a polymer matrix that swells when it comes into contact with body fluids. The swelling of the matrix causes the pores in the matrix to increase in size, which increases the rate of drug release. There are not many products that use swelling systems. Examples of swelling systems include: Oral products Intravaginal products ○ Chemical Controlled Systems (Bioerodible Polymers): In these systems, the drug is dispersed in a polymer matrix that degrades over time in the body. The degradation of the polymer releases the drug. ○ ○ Bioerodible polymers are typically degraded by hydrolysis, a chemical reaction that involves the breaking of bonds using water. ○ The degradation products of bioerodible polymers are biocompatible, meaning that they are not harmful to the body. ○ The biggest advantage of bioerodible polymers is that they do not need to be removed from the body. ○ The drug can be released from bioerodible polymers by both diffusion and degradation of the matrix. ○ Examples of bioerodible polymer systems include: Intracranial implants Oral products 3.10 Drug Delivery Routes and Technologies Depot Injectables Depot injectables are a long-standing drug delivery technology, primarily administered subcutaneously or intramuscularly. Suspensions: ○ One of the oldest technologies involves using slowly dissolving suspensions formulated in water or oil. ○ The slow dissolution of drug crystals in these suspensions prolongs drug release, as the drug must be in its molecular form to be absorbed into the bloodstream. ○ Products like 3-month depot injectables provide extended drug release using this simple formulation. Prodrugs: ○ Prodrugs are inactive compounds that are metabolized in the body to release the active drug moiety. ○ For depot injections, prodrugs are typically lipophilic, meaning they are fat-soluble and dissolve slowly , prolonging drug release. ○ The lipophilic nature of these prodrugs allows them to remain in the oily depot or subcutaneous/muscle tissue, gradually releasing the active drug over time. ○ Examples include Depo-Testosterone, a long-acting testosterone prodrug, and Invega Sustenna (Paliperidone palmitate), a long-acting antipsychotic with 1-month, 3-month, and 6-month versions. ○ Invega Sustenna incorporates a palmitate ester, a long hydrocarbon chain, making it highly lipophilic and resulting in slow dissolution and hydrolysis, ultimately releasing the active paliperidone. Bioerodible Microspheres Bioerodible microspheres represent a more advanced form of depot injectables. ○ Mechanism: ○ They consist of drug particles uniformly dispersed within a biodegradable polymer matrix. ○ Drug release occurs through two mechanisms: drug diffusion through the polymer and the gradual breakdown of the polymer itself. Lupron Depot: ○ A well-established example is Lupron Depot, a microsphere formulation of a gonadotropin-releasing hormone agonist. ○ It is composed of a copolymer of polylactic acid and polyglycolic acid, which break down into lactic , naturally occurring compounds in the body. acid and glycolic acid ○ Different versions of Lupron Depot provide 1-month, 3-month, and 4-month release durations. ○ Atrigel Delivery System The Atrogel delivery system is another biodegradable polymer-based technology that offers extended drug release. Mechanism: ○ The drug is dissolved in a biocompatible solvent and injected as a liquid. ○ Upon contact with aqueous fluids in the body, the liquid solidifies, forming a drug-containing matrix at the injection site. SUBLOCADE: ○ SUBLOCADE (buprenorphine) is an example of a drug utilizing the Atrogel system. ○ It is indicated for opioid use disorder in patients stabilized on transmucosal buprenorphine. ○ SUBLOCADE is injected subcutaneously in the abdomen and provides a 1-month release of buprenorphine from the solidified polymer depot. ○ The Atrogel system is biodegradable and breaks down into lactic acid and glycolic acid. ○ Liquid Crystal Nanotube This innovative and simple system represents one of the newest technologies discussed in the lecture. Somatuline Depot: ○ Somatuline Depot (lanreotide) utilizes this technology and is indicated for conditions like acromegaly and carcinoid syndrome. ○ The formulation is polymer-free and relies on the self-assembly of the lanreotide peptide into nanotubes , hollow tubes with nanometer dimensions. ○ ○ Upon injection, a depot of nanotubes forms, slowly releasing the medication over 4 weeks. ○ The nanotubes contain the majority of the medication, providing sustained release, while a small amount of free lanreotide provides an initial dose. Implants Implants are devices placed subcutaneously for long-term drug delivery, taking advantage of the characteristics of subcutaneous tissue. Subcutaneous Tissue Characteristics: ○ Rich in fat ○ Poor in blood perfusion and nerves, minimizing reactivity and discomfort ○ Relatively low reactivity to foreign matter, enabling prolonged implant retention Types: ○ Polymer implants: Biodegradable or non-biodegradable polymers used to control drug release. ○ Mechanical implants: Primarily titanium-based devices that use pumps to deliver medication. Polymer Implants Membrane-Controlled Reservoir Systems: ○ Characterized by a rate-controlling membrane surrounding a drug reservoir in a saturated solution. ○ Provide zero-order drug release, resulting in a consistent and predictable release profile over time. ○ Typically require surgical insertion and removal. ○ Nexplanon: Nexplanon is a contraceptive implant that exemplifies this system. It consists of a single implant containing the contraceptive medication embedded in a solid polyethylene vinyl acetate polymer matrix. The matrix primarily provides stiffness for ease of insertion and palpation. A rate-controlling membrane surrounding the matrix ensures zero-order release of the drug, providing up to 3 years of contraception. Nexplanon contains barium sulfate, making it radio-opaque and detectable by X-ray. ○ Other Examples: Hysterelin Acetate Implant (Suprellin LA) is another membrane-controlled reservoir system used for central precocious puberty. It is a non-biodegradable implant that releases gonadotropin-releasing hormone over a year. It contains histrelin acetate. Bioerodible Matrix Implants: ○ These implants are biodegradable, eliminating the need for surgical removal. ○ Drug release occurs through diffusion through the polymer and the gradual degradation of the polymer. ○ Example: A bioerodible matrix implant exists for the delivery of a contraceptive medication. This tiny implant is inserted into the abdomen and provides 1-month or 3-month drug release. Water penetrates and hydrolyzes the polymer. The implant is composed of polylactic acid and polyglycolic acid, which break down into lactic acid and glycolic acid. Gliadel Wafer: ○ The Gliadel wafer is a unique biodegradable intracranial implant used for the localized delivery of the chemotherapeutic agent carmustine. ○ It is implanted into the cavity left after tumor resection to target residual cancer cells. ○ The wafer consists of carmustine dispersed in a bioerodible matrix composed of compressed microspheres. ○ The polymer matrix slowly degrades, releasing carmustine locally into the surrounding brain tissue. ○ This localized delivery provides a 1000-fold higher carmustine concentration at the tumor site compared to intravenous administration, minimizing systemic exposure and potential side effects. ○ Mechanical Implants: Implantable Infusion Pumps Mechanism: ○ These implants use pumps to deliver medication under positive pressure. ○ They consist of a pumping mechanism, a drug reservoir, and a catheter surgically implanted with its tip at the target site. ○ ○ The device is typically housed in a subcutaneous pocket, often in the subclavian fossa or abdomen. ○ Spatial and Temporal Control: Implantable infusion pumps offer both spatial and temporal control over drug delivery. Spatial control: Achieved by the specific placement of the catheter tip, ensuring drug delivery to the desired location. Temporal control: Achieved by the pump's ability to deliver medication at a controlled rate over time. Examples: ○ SynchroMed 3: The SynchroMed 3 is an example of an implantable infusion system. It utilizes a peristaltic pumping mechanism where rotating cams squeeze the tubing, propelling the drug from the reservoir through the catheter into the patient. The reservoir can be refilled through a septum. SynchroMed 3 is approved for specific medications and indications. ○ Intrathecal Pump for Spasticity: An intrathecal pump, with its catheter tip placed in the spinal cord, is used for delivering medication to manage spasticity associated with multiple sclerosis. ○ Intrahepatic Artery Infusion Pump: The Entera 3000 is an example of a pump designed for intrahepatic chemotherapy. It delivers medication directly to the hepatic artery, targeting liver tumors. This targeted approach results in higher drug concentrations at the tumor site while minimizing systemic exposure. Programmability: ○ Some implantable pumps, like the SynchroMed 3, can be programmed externally to allow for patient-controlled analgesia (PCA) within physician-specified limits. ○ This feature grants patients a degree of control over their pain management while ensuring safe and appropriate dosing. ○ External Pumps External pumps, as their name suggests, are devices worn outside the body to deliver medication. Mechanism: ○ Similar to implantable pumps, external pumps utilize positive pressure to deliver medication in fluid form. ○ They can be categorized as electronic pumps or stored energy disposable pumps. ○ Stored energy disposable pumps: Rely on mechanisms like spring-loaded systems, gas pressure (propellants), or elastic rebound to generate the force for drug delivery. Types: ○ Hospital Infusion Pumps: These are commonly used in hospital settings for intravenous drug administration. They allow for precise control over infusion rates and can be programmed for various delivery modes, including continuous infusion, intermittent infusion, and bolus dosing. Examples of hospital infusion pumps are shown in the source material. ○ Ambulatory Infusion Pumps: Smaller and more portable, ambulatory infusion pumps are designed for home infusion therapy or outpatient use. ○ Patient-Controlled Analgesia (PCA) Pumps: PCA pumps allow patients to self-administer pain medication within predefined safety limits set by healthcare professionals. The pumps are programmed with parameters like bolus dose, maximum dose, background infusion rate, and a lockout interval to prevent overdosing. PCA pumps provide patients with greater control over their pain management and improve their overall comfort. ○ Insulin Pumps: Insulin pumps have witnessed remarkable technological advancements in recent years. Modern insulin pumps often integrate with continuous glucose monitoring (CGM) systems to provide automated and personalized insulin delivery based on real-time glucose levels. These pumps offer precise basal insulin delivery and allow for bolus dosing as needed, mimicking the function of a healthy pancreas. Examples include the Dexcom G7 CGM system and the MiniMed 780g system, which combines an insulin pump with a CGM component. ○ Elastomeric Infusion Pumps: These are stored energy disposable pumps that utilize an elastic membrane, often referred to as a "balloon," to deliver medication. The balloon is filled with the drug fluid, and as it contracts, it forces the fluid out through a catheter and into the patient. A flow restrictor controls the rate of drug delivery. Elastomeric pumps are commonly used for ambulatory settings due to their simplicity and portability. While they offer temporal control over drug delivery, they are generally not as accurate as electronic pumps, and the rate of delivery is predetermined by the specific pump selected. Spatial Control and Targeted Drug Delivery Spatial control in drug delivery refers to the ability to target medication to specific sites within the body, enhancing therapeutic efficacy and minimizing off-target effects. Particulate Drug Carriers: ○ Particulate systems, including liposomes, albumin nanoparticles, and antibodies, are used to achieve spatial control in parenteral drug delivery. ○ These carriers encapsulate or conjugate drugs, protecting them from degradation, prolonging their circulation time, and facilitating their delivery to target tissues. Liposomes Structure: ○ Liposomes are spherical vesicles composed of a phospholipid bilayer, resembling tiny, empty cells. ○ They have an aqueous interior and a lipid bilayer membrane, allowing for the encapsulation of both hydrophilic and lipophilic drugs. ○ Hydrophilic drugs reside within the aqueous interior, while lipophilic and amphiphilic drugs are intercalated within the lipid bilayer. Mononuclear Phagocyte System (MPS) Evasion: ○ Liposomes, being particulate matter, are susceptible to uptake by the MPS, particularly macrophages, which are responsible for clearing foreign substances from the body. ○ To avoid rapid clearance by macrophages, stealth liposomes have been developed. ○ Stealth liposomes: Incorporate polyethylene glycol (PEG) chains on their surface. The PEG chains create a hydrated layer around the liposome, effectively cloaking it from macrophage recognition, prolonging its circulation time. Examples: ○ Doxil (pegylated liposomal doxorubicin): Used for treating Kaposi's sarcoma in HIV patients. The liposomal formulation prolongs doxorubicin's half-life and enhances its localization to tumor sites. Pegylation reduces doxorubicin's cardiac toxicity, a common side effect of the conventional formulation. ○ DaunoXome (liposomal daunorubicin): Another liposomal chemotherapeutic agent used for Kaposi's sarcoma. ○ Vyxeos (liposomal daunorubicin and cytarabine): A liposomal formulation containing two chemotherapeutic agents for treating leukemia. The liposomal encapsulation allows for a fixed molar ratio delivery of the drugs to leukemia cells. ○ Ambisome (liposomal amphotericin B): An antifungal medication formulated in liposomes to reduce its nephrotoxicity. Amphotericin B, being highly lipophilic, incorporates into the liposome's lipid bilayer. Applications: ○ Liposomes are used in a variety of applications beyond the examples mentioned above, including the delivery of other chemotherapeutic agents, antivirals, and gene therapies. Albumin Nanoparticles Abraxane (paclitaxel protein-bound particles): ○ Abraxane utilizes albumin nanoparticles as drug carriers. ○ Paclitaxel, a highly lipophilic chemotherapeutic agent, is bound to albumin, eliminating the need for the toxic excipients (cremophor EL and alcohol) used in the conventional formulation. ○ This results in a better-tolerated formulation without the need for premedication. ○ Unique Characteristic: Abraxane is a suspension of albumin-bound paclitaxel nanoparticles that can be administered intravenously despite being a suspension. ○ This is possible due to the tiny size of the nanoparticles, which are well below the threshold for causing embolisms. ○ Abraxane may also exhibit some degree of tumor targeting due to the presence of albumin receptors on tumor cells. Antibody-Drug Conjugates (ADCs) Mechanism: ○ ADCs combine the targeting capabilities of antibodies with the cytotoxic potential of anticancer drugs. ○ The antibody component specifically binds to antigens present on target cells, delivering the attached drug directly to those cells. Example: ○ Besponsa (inotuzumab ozogamicin): An ADC indicated for a type of leukemia. The antibody component, inotuzumab, targets the CD22 antigen found on leukemia cells. Upon binding, the ADC is internalized, releasing the cytotoxic drug ozogamicin inside the cancer cell, leading to cell death. Key Concepts: The lecture emphasizes the ongoing evolution of drug delivery technologies. Parenteral drug delivery offers diverse approaches for achieving spatial and temporal control over drug release. Depot injectables provide extended drug release through slow dissolution or prodrug strategies. Bioerodible microspheres combine drug diffusion with polymer degradation for controlled drug release. The Atrogel system forms a drug-releasing matrix in situ. Liquid crystal nanotubes represent a novel, polymer-free approach to sustained drug delivery. Implants offer long-term drug delivery, with polymer implants relying on membrane control or bioerodibility, while mechanical implants utilize pumps. External pumps provide controlled drug delivery in various settings, including hospitals and ambulatory care. Spatial control is achieved through targeted drug delivery using particulate systems like liposomes, albumin nanoparticles, and antibody-drug conjugates. These systems enhance drug efficacy and minimize off-target effects. 3.12 Oral Routes of Administration and Advanced Drug Delivery Technologies The terminology for oral drug delivery products has evolved over time, but the FDA currently uses two main terms: extended release and delayed release. Extended release products are formulated to make the drug available over a longer time. Delayed release products release the drug at a time other than immediately after administration. A classic example of a delayed release product is an enteric-coated product. Many companies specialize in extended release or delayed release technologies and partner with larger pharmaceutical manufacturers to bring these products to market. Types of Oral Controlled Release Systems There are several approaches for oral controlled release, including: ○ Matrix systems (dissolving and non-dissolving) ○ Gastro retentive systems ○ Coated particles ○ Osmotically controlled systems ○ Ion exchange systems ○ Delayed release (enteric-coated) systems Matrix Systems Non-dissolving matrices will pass through the body and end up in the feces. ○ These matrices may dissolve or pass through the body intact. Classic matrix systems typically release the drug at a rate proportional to the square root of time. Wax matrix systems are composed of lipid wax with the drug embedded within. ○ These systems are processed into granules and compressed into tablets. ○ Examples include potassium chloride extended-release tablets (e.g., Klor-Con, Micro-K) and Slow-K. ○ The purpose of wax matrix systems for medications like potassium chloride and iron is not to provide a prolonged level of medication, but to prevent GI irritation by preventing high local concentrations. ○ Another example of a wax matrix system product is Slow Fe, an iron supplement designed to reduce local irritation. Swelling erodible matrix systems combine swelling and bioerodible properties. ○ They utilize hydrophilic polymers (e.g., hydroxypropyl methylcellulose HPMC or hypromellose) that form a swelling matrix upon contact with body fluids. ○ Both components of the system are bioerodible and leave no residue in the GI tract. ○ The Geomatrix system is an example of a swelling erodible matrix system. It is a multilayer tablet with a drug matrix core that hydrates and swells over time, increasing surface area. It also has modulating barriers that control the rate of hydration, allowing the drug to diffuse through for a prolonged effect. Paxil CR is an example of a product that utilizes the Geomatrix system. Gastro Retentive Systems Gastro retentive systems are designed to keep the dosage form in the stomach for an extended period. This allows for drug release in the stomach, ensuring it is ready for absorption in the small intestines. Different technologies are employed, including swelling, high density, and floating systems. ○ Swelling systems are a common type, where a swelling polymer prevents the dosage form from passing through the pylorus (HPMC). ○ The AccuForm system is an example, utilizing swelling polymers like hypromellose to create a large gel-like substance that stays in the stomach for 8-10 hours while slowly releasing medication. Examples of medications that use the AccuForm system include Metformin GR, Glucophage XR , and Fortamet. Coated Particle Systems Coated particle systems involve coating drug particles ranging in size from microcrystals to granules. These systems can use dissolving coats, non-dissolving coats (membranes), or a combination of both. Dissolving coat systems consist of drug pellets or granules surrounded by a coat that slowly dissolves, controlling the rate of drug dissolution and prolonging the medication's effect. ○ ○ An example is a system where pellets are created by coating a drug solution onto inner beads made of sugar and starch, followed by a coating of slowly dissolving material like wax or polymer. ○ The release rate is controlled by the coating thickness, with a mixture of beads with different thicknesses and uncoated beads providing an initial dose and a prolonged steady release pattern. ○ ○ A proprietary name for this type of system is Expansial, developed by GlaxoSmithKline. Dexedrine Spansules and Compazine Spansules are examples of products that utilize the Expansial system. Ornade Spansules are another example, characterized by their colorful beads with varying coating thicknesses. Combination non-dissolving/dissolving coat systems are a more recent and sophisticated approach. ○ Elan, a company specializing in controlled release systems, developed the SODAS (Spheroidal Oral Drug Absorption System). ○ This system involves uniform spherical beads with a multilayered structure of drug and excipients, where release is controlled by polymer coatings. ○ The polymers can be water-soluble, water-insoluble, or pH-responsive, and combinations of these can be used. ○ ○ Ritalin LA and Concerta are examples of products that utilize the SODAS system. Chronotherapy Chronotherapy is a type of temporal control that delivers medication at the optimal time for the drug's purpose, aligning with the patient's biological rhythms. For example, Verelan PM, using the CODAS (Chronotherapeutic Oral Drug Absorption System), delays the release of verapamil for 4-5 hours to provide blood level control during sleep when symptoms like angina or congestive heart failure may worsen. Membrane-Controlled Systems Membrane-controlled pellets involve tiny pellets surrounded by a rate-controlling membrane, typically resulting in zero-order drug release. ○ Toprol XL , a scored tablet containing membrane-controlled pellets of metoprolol succinate, is an example. ○ ○ While most extended-release products should not be crushed or broken, Toprol XL can be broken because the pellets that control release remain intact. Membrane matrix hybrid systems combine the features of membranes and matrices, with the membrane primarily controlling release. ○ Elan developed the IPDAS (Intestinal Protective Drug Absorption System), which uses tiny beads composed of a drug micro matrix surrounded by a rate-limiting semipermeable membrane. ○ The membrane typically plays the primary role in controlling release while the matrix provides a drug reservoir. ○ ○ Naprelan , containing naproxen, is an example of a product that utilizes the IPDAS system. ○ The immediate release component of this system helps reduce local concentrations and GI side effects common with NSAIDs. Osmotically Controlled Systems Osmotically controlled systems utilize a tablet with two compartments: an osmotic drug core and a polymeric push compartment. ○ Alza developed the GITS (Gastrointestinal Therapeutic System), where the osmotic component draws fluid in through a rigid semipermeable membrane. ○ The tablet cannot expand, but the expanding polymer in the push compartment forces the drug suspension out of a laser-drilled hole. ○ This results in near zero-order drug release. ○ Procardia XL , an example of this system, is a plastic tablet with a laser-drilled hole for drug release. Variations of the GITS system exist, including those with a drug-containing coating for an immediate release component and those with two different drug concentrations inside for ascending drug delivery. ○ Concerta , a methylphenidate extended-release product, has a coating for an initial dose and two internal compartments with different concentrations for an ascending release pattern over a prolonged period. Another variation includes an intervening layer that prevents water penetration for 4-5 hours, leading to a delayed release and chronotherapeutic effects. ○ Verelan PM is an example, designed for bedtime dosing with drug release occurring in the morning. Ion Exchange Systems Ion exchange systems utilize resins with charged functional groups that bind to the drug. The drug is released by exchanging with ions in the body. Pennkinetic , developed by Penwalt, uses an ion exchange resin coated with a non-dissolving polymer to control the flow of GI ions and drug release. ○ Tussionex suspension, containing hydrocodone, is formulated with an ion exchange resin surrounded by a rate-controlling semipermeable membrane. ○ Delsym , an over-the-counter long-acting dextromethorphan product, also utilizes this technology. ○ Delayed Release Systems Delayed release systems, often referred to as enteric coating, release the drug at a delayed time, typically in the small intestines. Traditional enteric coatings use phthalates with carboxyl groups that ionize and dissolve at a pH of 5.2 or higher. Other polymers, such as Eudragit (methacrylic acid copolymer), dissolve at a pH of 7 or greater, releasing the drug lower in the GI tract. ○ Asacol , a mesalamine product for ulcerative colitis, utilizes Eudragit for local action in the lower GI tract. ○ The SUBA system by Mann Pharma uses microencapsulated nanoparticles of itraconazole dispersed in a polymeric matrix. ○ Itraconazole, a poorly soluble drug, is in its amorphous form for enhanced solubility. ○ The system utilizes hypromellose phthalate as a delayed-release polymer. ○ Sporanox is an example of a product that uses the SUBA system. Important Considerations The various technologies used in oral modified release products result in different plasma profiles, making it generally unacceptable to switch between different modified release products of the same drug. Pharmacists must be aware of the specific properties and release characteristics of each product they dispense. 3.12 Transdermal Drug Delivery: Patches Patches are adhesive medicated patches that deliver medication through the epidermis for mainly systemic purposes. They consist of: ○ An occlusive backing to protect the patch and prevent drug escape. ○ A drug layer with a polymer control mechanism. ○ An adhesive layer to stick to the skin. ○ A release liner to prevent premature sticking. Ideal Drugs for Transdermal Delivery Small size: To facilitate passage through interlaminar lipids. Lipophilic nature: To dissolve in the lipid layers of the skin. Potency: Effective at low concentrations due to limited drug delivery compared to oral or injectable routes. Penetration Enhancers in Transdermal Drug Delivery Purpose: To increase drug permeation through the skin. Common enhancers: Ethanol and propylene glycol. Mechanism: Disruption of interlaminar lipids in the stratum corneum, creating spaces for drug travel. Other mechanisms: Altering keratin to prevent drug binding or enhance release. Application Site Considerations for Transdermal Patches Manufacturer recommendations: Adhering to the approved application sites is crucial. Variations in skin properties: Different body areas have varying stratum corneum thickness, appendage density (hair follicles, sweat glands), affecting drug permeation. Importance of following instructions: Applying patches to unapproved sites may result in altered drug absorption and therapeutic effects. Patch Size and Drug Release Surface area and drug release: The size of the patch directly affects the amount of drug released over time, as described by the flux equation. Nitroglycerin patch example: Different doses of nitroglycerin patches are achieved by varying the patch size while maintaining the same design. Limitations of Transdermal Patches Limited application time: Typically 7 to 10 days due to occlusiveness. Occlusion effects: Blocking transepidermal water loss leads to: ○ Increased drug permeation: By enhancing skin hydration and expanding lipid lamellae. ○ Skin hydration and maceration: Prolonged occlusion can cause skin breakdown. ○ Microbial growth: Occluded, moist environments favor the growth of potentially pathogenic microbes. Skin changes and permeability: Occlusion-induced skin changes can alter drug permeability, which may be a desired effect for some patches. Heat and Transdermal Patch Usage Increased drug release: Applying heat to a patch, even from sources like heating pads or fever, can significantly increase drug release and absorption. Potential dangers: Applying heat to patches can lead to excessive drug absorption and potential toxicity, especially for drugs like fentanyl. Types of Transdermal Patch Designs Adhesive Matrix Patches: ○ Dominant type, characterized by the adhesive serving as the drug matrix. ○ Advantages: Super thin, translucent or transparent, less noticeable. ○ Examples: Nitroglycerin, Climara (estradiol), Fentanyl, Daytrana (methylphenidate), contraceptive patches, Lidoderm (lidocaine), Flector (diclofenac). Reservoir Patches: ○ Older design featuring a drug reservoir, typically a saturated solution, separated from the skin by a rate-controlling membrane. ○ Characteristics: Elevated drug reservoir, potential for leakage, zero-order release pattern. ○ Example: Androderm (testosterone). Membrane-Matrix Hybrid Patches: ○ Combines a drug matrix with a rate-controlling membrane. ○ Advantages: Provides both immediate and sustained drug release. ○ Purpose of drug in adhesive matrix: Initial burst effect, saturation of skin binding sites. ○ Examples: Catapres (clonidine), Transderm Scop (scopolamine), Fentanyl. Other Advanced Drug Delivery Systems Intravitreal Implants: ○ Membrane-controlled reservoir implants sutured into the vitreous humor. ○ Example: Retisert (fluocinolone acetonide) for chronic non-infectious uveitis. ○ Characteristics: Non-biodegradable, provides 30 months of drug delivery. Intrauterine Devices (IUDs): ○ Membrane-controlled reservoir systems releasing medication over extended periods. ○ Example: Mirena (levonorgestrel) for contraception, providing steady release for 8 years. Intravaginal Products: ○ Swellable matrix system: Example: Cervidil (dinoprostone) for cervical ripening. ○ Vaginal rings: Flexible membrane-controlled reservoir rings. Examples: NuvaRing (etonogestrel and ethinyl estradiol), Estring (estradiol). Dental Products: ○ Biodegradable microsphere systems: Example: Arestin (minocycline) for periodontal disease. ○ Mechanism: Microspheres containing minocycline break down over time, releasing the drug locally for 21 days. Pulmonary Drug Delivery: ○ Liposomal inhalation suspensions: Example: Arikayce (amikacin) for Mycobacterium avium complex lung disease. ○ Mechanism: Liposomes encapsulate the drug and are naturally taken up by macrophages, targeting the site of infection. Arikayce (Amikacin Liposome Inhalation Suspension) FDA Designations: Fast track, breakthrough therapy, priority review, qualified infectious disease product. 3.13 History of Biopharmaceuticals Biotechnology is the merging of biology and technology to create useful products from raw materials. The term was coined in 1919 by Karl Ereky, a Hungarian agricultural engineer. The first biopharmaceutical, somatostatin, was produced in 1977 by the company Genentech using recombinant DNA technology. This marked the beginning of the biotechnology age. In 1982, the FDA approved Eli Lilly's human insulin, the first human insulin created using recombinant DNA technology. Biologics vs. Small Molecule Drugs Biologics are medications isolated from natural sources like humans, animals, and microorganisms, or produced through biotechnology. They include vaccines, blood components, gene therapy, tissues, recombinant proteins, and antibodies. Key differences between biologics and small molecule drugs : ○ Size and Complexity: Biologics are larger and more complex molecules than small molecule drugs. For example, antibodies can be 150 kilodaltons in size, while small molecules are typically around 21 atoms. ○ Production Process: Biologics are often made from living cells or tissues, while small molecule drugs are made through chemical synthesis. For example, insulin is a biologic produced by bacteria, while metformin is a small molecule drug produced through chemical synthesis. Regulation: Biologics and small molecule drugs have different review and approval processes under FDA rules. Insulin is an exception, as it was initially regulated as a drug but has since transitioned to being regulated as a biologic. Generic Biologics: The FDA's decision to regulate insulin as a biologic allows for the introduction of generic insulin , potentially increasing competition and lowering prices. The Rise of Biologics The number of biologic drug approvals has been increasing dramatically. In 2023, biologics accounted for 48% of new drug approvals, surpassing small molecule drugs. This trend is driven by the development of new biological modalities and the FDA's Center for Biologics Evaluation and Research (CBER). Therapeutic areas with a high number of biologics in development : ○ Cancer ○ Infectious Diseases ○ Autoimmune Diseases ○ Neurological Diseases Leading product categories for biologics in development : ○ Monoclonal Antibodies ○ Vaccines ○ Recombinant Proteins ○ Cell Therapy ○ Gene Therapy Protein Structure Four levels of protein structure : ○ Primary Structure: The linear sequence of amino acids. ○ Secondary Structure: Repeating patterns like alpha-helices and beta-sheets. ○ Tertiary Structure: The three-dimensional folding pattern of a single protein molecule. ○ Quaternary Structure: The arrangement of multiple protein subunits into a larger complex. Factors Affecting Protein Structure and Function Tertiary structure is critical for a protein's biological properties. It's determined by: ○ Amino acid sequence ○ Chain alignments ○ Glycosylation ○ Cellular environment Post-translational modifications can be used to modify and improve protein properties. Interactions that stabilize protein folding: ○ Disulfide bonds between cysteine residues ○ Hydrophobic interactions between nonpolar amino acids ○ Ionic interactions between oppositely charged residues ○ Hydrogen bonds Protein Conjugation: ○ Involves the attachment of a prosthetic group (inorganic or organic) to a protein. ○ Examples of prosthetic groups: metals (e.g., iron), lipids, carbohydrates (e.g., glycosylation sugars) Glycosylation: ○ The attachment of sugar molecules (oligosaccharides) to proteins. ○ Contributes to proteomic diversity. ○ Types: N-linked (occurs through asparagine residues) and O-linked (occurs through serine and threonine residues). Importance of Glycosylation: ○ Affects protein recognition, interaction, and solubility. ○ Influences protein stability by: Improving thermal stability Decreasing aggregation Decreasing proteolysis Other Post-Translational Modifications: ○ Chemical modifications (e.g., carboxylation, hydroxylation, sulfation, amidation) can also alter protein properties. Manufacturers can intentionally modify glycosylation to enhance stability and therapeutic benefits. Protein Production Ex vivo protein production pipelines use different expression systems: ○ E. coli (bacterial) ○ Yeast ○ Mammalian The DNA sequence for the desired protein is inserted into the chosen host cell, which then expresses and produces the protein. Comparison of Expression Systems Host cell selection is critical for recombinant protein production. Bacterial Cells (E. coli): ○ Advantages: Simple growth Easy to handle Rapid growth High yield ○ Disadvantage: No glycosylation possible Yeast Cells: ○ Advantages: Simple growth Resemble mammalian cells Rapid growth Good yields Good glycosylation fidelity Good secretion activity ○ Disadvantage: Glycosylation is not identical to human glycosylation Mammalian Cells: ○ Advantages: Best folding and glycosylation fidelity (human-like) ○ Disadvantages: Slow culture Expensive production Plant Cells: ○ Advantages: Simpler growth requirements than mammalian cells ○ Disadvantage: Limited to plant-based products Antibody Production and Function Antibodies are therapeutic drugs that our bodies naturally produce to fight off foreign invaders. Antibody production process in the body : ○ Antigens (foreign invaders) bind to B cells that have matching antibodies on their surface. ○ This binding triggers the B cell to multiply and secrete more of the same antibody. Antibodies protect against foreign molecules like viruses and bacteria. How antibodies work : ○ They bind to antigens on the surface of invaders and mark them for destruction. ○ Macrophages then ingest and destroy the marked invaders. ○ Special lymphocytes can attack and kill infected cells if macrophages are too late. Monoclonal Antibody Production Hybridoma technology is used to produce monoclonal antibodies. Steps involved : ○ An animal (e.g., a mouse) is challenged with the antigen. ○ The animal's B cells produce antibodies against the antigen. ○ Spleen cells containing B cells are extracted and fused with myeloma cells (tumor cells with unlimited proliferation). ○ The fused cells, called hybridomas, are cultured and produce large amounts of antibodies. ○ Antibodies are harvested from the hybridoma culture. Types of Antibodies and Their Production Types of Antibodies : ○ Monoclonal antibodies ○ Polyclonal antibodies ○ Recombinant antibodies Recombinant Antibody Production: ○ Uses DNA sequencing and bacterial expression systems to produce antibodies, making it cheaper and faster than hybridoma technology. ○ Offers greater flexibility in terms of antibody format and species. ○ Advantages of Recombinant Antibodies: Cheaper and faster production No animal use required Flexible antibody formats (e.g., Fab fragments, single-chain variable regions) Species switching possible Reliable and highly reproducible Can be optimized for improved affinity and other properties using bioinformatics and AI. Biologics Workflow and Manufacturing Biologics development is a lengthy and expensive process, taking around 12-15 years and costing millions of dollars. Workflow steps : ○ Target identification ○ Assay construction and target screening ○ Target validation ○ Safety package development ○ Clinical trials (Phase 1: safety testing; Phase 2: efficacy and safety; Phase 3: efficacy and safety in a larger population) ○ FDA review and approval Factors contributing to high production cost : ○ Complexity of biologics ○ Low number of patients for some biologics ○ Toxicity and immunogenicity concerns, requiring extensive safety testing Monoclonal Antibody Manufacturing Process: ○ Source DNA containing the target gene is cloned into a vector. ○ The vector is inserted into a host cell (bacteria, yeast, or mammalian). ○ Cells are cultured and expanded in growth media. ○ Large-scale culture is performed in bioreactors. ○ Purification steps are carried out to isolate the antibody from other cellular components and impurities. ○ Quality control (QC) measures are implemented throughout the process. Impurities in Recombinant Protein Production and Purification: ○ Potential contaminants: cell debris, viruses, protein contaminants from growth media, endotoxins ○ Purification methods are crucial for removing impurities and ensuring product purity. YouTube video resource for a comprehensive overview of purification steps. Factors Influencing Biologic Drug Cost and Safety Manufacturing complexity and host cell type affect drug cost. ○ Bacteria: easiest and cheapest to manufacture ○ Yeast: intermediate difficulty and cost ○ Mammalian cells: most difficult and expensive Immunogenicity and Toxicity: ○ Biologics derived from sources closer to human origin tend to have lower immunogenicity and toxicity. ○ Mammalian cell expression systems produce the most compatible products for humans. ○ Humanized antibodies have reduced immunogenicity. ○ Fully human antibodies have the lowest immunogenicity and toxicity. mRNA-Based Antibody Therapy mRNA-based antibody therapy is a promising and increasingly popular approach. Advantages: ○ High safety and low immunogenicity ○ Transient expression, reducing the risk of long-term side effects ○ Potential for personalized therapy Mechanism: ○ mRNA encoding the desired antibody is designed and manufactured. ○ The mRNA is administered to the patient. ○ Host cells in the patient's body take up the mRNA and express the antibody. ○ The expressed antibody targets and neutralizes or kills the intended target (e.g., tumor cells, bacteria, viruses). Advantages over Traditional Recombinant Protein and DNA-Based Therapies: ○ Reduced immunogenicity and toxicity ○ Natural post-translational modifications ○ Potential for improved tolerance Challenges and Considerations: ○ Higher production cost ○ Difficulty in expression ○ Potential for immune sensor activation (needs further investigation) Example: mRNA-Based COVID-19 Vaccines: ○ mRNA vaccines carry the genetic blueprint for the spike protein of the SARS-CoV-2 virus. ○ The body's cells express the spike protein, triggering an immune response and providing immunity against COVID-19. Conclusion The use of biologics in drug development is rapidly increasing. Understanding the production, manufacturing, cost, and safety considerations of biologics is essential. The next session will focus on managing biologics in terms of stability and therapeutic property development. 3.14 Pharmaceutical Biotechnology Study Guide Session 2: Introduction to Pharmaceutical Biotechnology This session focuses on the stability of biotech drugs and practical aspects pharmacists need to know about dispensing biologics. The session also aims to explain the concept of biosimilars and how they differ from generic drugs. Fundamentals ○ Isoelectric Point (pI) pI is the pH at which a protein molecule has a net charge of zero, making it neutral. pI is crucial in determining protein stability and related properties. Proteins have positively charged amine groups and negatively charged carboxyl groups. At low pH, proteins are protonated, showing a more positive charge. At high pH, proteins are deprotonated, showing a more negative charge. At pI, the positive and negative charges balance, resulting in a neutral charge. pI affects protein stability, precipitation, and interactions. Adding a base increases negative charges, maximizing protein-water interaction. Adding an acid increases positive charges, potentially reducing interactions. Minimized protein-water interactions at pI (neutral charge) lead to precipitation. The example of "sweet ice," a peptide composed of amino acids, illustrates this concept. Depending on the pH of the surrounding solution, the amino acid side chains within the peptide can be protonated or deprotonated, leading to changes in the overall charge of the molecule. Manipulating the pH, such as by adding an acid or a base, can alter the charge and thereby influence the protein's interactions with water and its propensity to precipitate. ○ Factors Affecting Stability Chemical Instability Covalent modification of the protein Involves bond formation or cleavage, creating new chemical structures. Occurs through chemical reactions like deamidation, hydrolysis, and oxidation. Influenced by storage conditions and buffer pH. Deamidation: Removal of ammonia from an amide, commonly affecting asparagine and glutamine, leading to changes in charge. Hydrolysis: Breakdown of peptide bonds by water, reducing protein activity. Oxidation: Reaction with atmospheric oxygen, commonly targeting cysteine residues, affecting protein stability. Controlling Chemical Instability Hot Spot Amino Acids: Specific amino acids that influence susceptibility to hydrolysis. Changing the amino acid sequence can reduce hydrolysis and enhance stability. Examples include Asp-Pro sequences. Oxidation Control: Packaging lyophilized proteins under nitrogen and modifying cysteine sequences can reduce oxidation. Disulfide Bond Exchange: Oxidation can lead to disulfide bond exchange, altering protein conformation and properties. Maillard Reaction: Reaction between a sugar's carbonyl group and an amino acid's amino group, forming new active protein structures. Physical Instability (Denaturation) Loss of native protein conformation, potentially leading to loss of activity. Affects the tertiary structure of the protein, including domains, disulfide bonds, and folding, crucial for activity and properties. Causes of Denaturation: Heating pH changes Organic cosolvents Salt concentration Mechanical stress (e.g., shaking, freezing) Drying Surfactants Denaturation leads to aggregation , changing the protein's structure and potentially increasing antigenicity, leading to toxicity. Visible signs of denaturation include precipitation, bubbles, and cloudiness in the protein solution. Denaturation is irreversible. Aggregation: Can be due to non-covalent or covalent forces (e.g., disulfide bond breakage and exchange). Can render the product unusable as described in package inserts. Absorption to Surfaces: Denatured protein can absorb to surfaces, leading to inactivation and removal from the solution. ○ Formulation of Protein Drugs Formulation is crucial for stability and is more complex than traditional drug formulations. Factors to Consider: pH: Highly relevant to the protein's pI. Balancing chemical stability and avoiding aggregation. pH influences solubility. Inorganic Salts: Enhance thermal stability, especially di-cations like zinc. Ionic strength (salt concentration) is important. Stabilizers: Protect against physical degradation, aggregation, precipitation, absorption, and denaturation. Examples: Albumin, glycine, Tweens, polysorbates. Antioxidants and Chelators: Maintain protein stability. Preservatives: Commonly used in protein drug formulations. ○ Preservation of Protein Drugs: Refrigeration: Low temperatures prevent denaturation. Freezing: Requires cryoprotectants (e.g., sugars, amino acids) to avoid damage from freeze-thaw cycles. Lyophilization (Freeze-Drying): Uses cryoprotectants and water-replacing stabilizers (e.g., sugars) to maintain a glassy state. ○ Novel Preservation Approaches: Site-Directed Mutagenesis: Replacing hot spot amino acids (e.g., with serine) to increase stability. Chemical Modification: Attaching PEG polymers to stabilize the protein drug. Administration of Protein and Peptide Drugs: ○ Challenges: Physical Barriers to Transport: In vivo barriers like intestinal epithelium and capillary endothelia hinder transport. Peptides and proteins larger than four amino acids have difficulty crossing barriers. Capillary Endothelia: Varying permeability based on location. Sinusoidal: Discontinuous with large gaps (>100 nm) in liver, spleen, bone marrow, and lymph nodes. Fenestrated: Gaps (60-100 nm) covered by diaphragms in the GI tract and renal glomeruli. Continuous: Present in lung, brain, muscle, and subcutaneous tissue; permeability depends on protein size. Lymphatic System: Important for absorbing proteins larger than 20 kDa. Route of Administration: Determined by protein size and target location. Example: Monoclonal antibodies (>20 kDa) are administered through the lymphatic system to reach circulation. Chemical and Enzymatic Degradation: Proteases: Enzymes that break down proteins, present in various locations in the body. Types: Serine, cysteine, aspartate, metalloproteases. Locations: Body fluids, mucous membranes, cell membranes, liver, kidney, lung. Immunogenicity: The potential of a substance to trigger an immune response. Formulations (e.g., PEG) can introduce immunogenicity. Routes of Administration: ○ Nasal: Advantages: Rich vasculature, rapid absorption, avoids hepatic first-pass metabolism. Disadvantages: Catabolism, mucociliary elimination, limited surface area, decreased absorption with increased size. Examples: Lypressin, desmopressin acetate, vasopressin analogs, oxytocin, nafarelin (synthetic peptide products). Limited bioavailability (10-40%) due to size restrictions and mucus barriers. Optimal size for absorption: Smaller than 2-5 microns (aerosol) or 2 microns (particles). ○ Pulmonary: Advantages: Larger surface area than nasal, buccal, or rectal routes; short distance to capillaries. Disadvantages: Inconsistent delivery to absorption sites due to individual variations in lung structure, generally low bioavailability. Suitable for local and systemic delivery, dosage may be non-critical. Examples: Recombinant DNase (local delivery), inhaled insulin (systemic delivery). ○ Parenteral: Intravenous is the preferred route in many cases. Challenges: Vascular barriers (e.g., capillary endothelium), proteases. Advantages: Potential for controlled release (CR) products, preferred for self-administration. ○ Oral: Most desired route but faces challenges from the GI tract (transport and degradation). Formulations like vesicles, micelles, and solid polymers protect drugs from GI degradation. Popular due to ease of self-administration. Improving Protein Delivery: ○ Protein Modification: Chemical Conjugation (e.g., Pegylation): Adding PEG to modify protein structure and increase stability. Site-Directed Mutagenesis: Engineering recombinant analogs by replacing hot spot amino acids to enhance stability. Example: Insulin lispro, where reversing the amino acids at positions 28 (Pro) and 29 (Lys) increases stability. Generics vs. Biosimilars: ○ Generic Biopharmaceuticals: The concept of generic drugs does not apply to biopharmaceuticals in the same way as small molecule drugs. Small Molecule Drugs: Fixed molecular structure, allowing for generic equivalents. Protein Drugs: Modifications (e.g., changing one amino acid) create challenges for generic regulation. ○ Generic Drugs: Identical or bioequivalent to the innovator (reference listed) drug. Match in dosage form, safety, strength, route of administration, quality, performance, characteristics, and intended use. Chemically identical to the original drug and must be bioequivalent. Manufactured reproducibly under controlled conditions. ○ Biosimilars: Term used instead of "generics" for biologics, especially complex proteins and antibodies. Follow-on products are not chemically identical to the reference drug, potentially having modifications in molecular structure. Key Differences from Generic Drugs: Size and Complexity: Biosimilars are larger and more complex than generic drugs. Manufacturing: Biosimilars are produced in living systems (e.g., CHO cells) using complex processes. Deviations in cell lines and production conditions can lead to differences in the final product. Structure: Generic drugs are chemically identical, while biosimilars are similar but not identical to the reference drug. Administration: Generics are often oral, while biosimilars may require intravenous or subcutaneous administration. Half-Life: Biosimilars typically have longer half-lives (up to weeks) compared to generic drugs (hours). Anti-Drug Antibody Formation: Possible in biosimilars, affecting pharmacokinetic properties, not applicable to generics. ○ Biosimilar Evaluation: Requires extensive analysis, including tests for purity, structure, uniformity, potency, glycosylation patterns, and aggregation. Different degradation mechanisms contribute to product heterogeneity. ○ Immunogenicity in Biosimilars: Even biosimilars of the same drug can have different immunogenicity profiles, raising safety concerns. Each biosimilar requires unique safety evaluation. Clinical studies are often needed to assess therapeutic differences and safety concerns. ○ Cost of Biosimilars: Production costs remain high due to complex manufacturing and clinical study requirements. Not considered "cheap generics." FDA Approval of Biosimilars: ○ Biosimilarity: Demonstrating high similarity to the licensed reference product. No clinically meaningful differences in safety, purity, and potency. ○ Interchangeability: The biosimilar product must be expected to produce the same clinical results as the reference product in any given patient. Alternating or switching between the biosimilar and reference product should not pose greater risks than using the reference product alone. Interchangeable products can be substituted at the pharmacy level without healthcare provider intervention. Conclusion: ○ The lecture covered key aspects of biologics, protein drug stability, formulation, delivery, biosimilars, and FDA regulations. ○ Understanding these concepts is essential for pharmacists dispensing biologics and biosimilars.