Cytoskeleton 80-100 PDF
Document Details
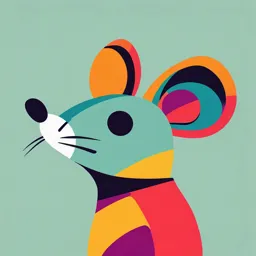
Uploaded by HonestChlorine9195
Koç University
Tags
Summary
This document provides a detailed explanation of microtubules, including their structure, function, and interactions within cells. It features diagrams and illustrations to support the concept. The document does not appear to be exam papers.
Full Transcript
A centriole consists of a cylindrical array of short, modified microtubules arranged into a barrel shape with striking ninefold symmetry. Together with a large number of accessory proteins, the centrioles recruit the pericentriolar material, where microtubule nucleation takes place. The pericentriol...
A centriole consists of a cylindrical array of short, modified microtubules arranged into a barrel shape with striking ninefold symmetry. Together with a large number of accessory proteins, the centrioles recruit the pericentriolar material, where microtubule nucleation takes place. The pericentriolar material consists of a dense spherical matrix that is thought to form through a process of biomolecular condensation. The centrosome duplicates before mitosis, forming a pair of centrosomes that each contain a centriole pair. When mitosis begins, the two centrosomes move apart to form the poles of the mitotic spindle. Charge distribution on the surface of microtubule (red indicates negative charges, blue positive charges) Localization of MAPs in the axon and dendrites of a neuron. This immunofluorescence micrograph shows the distribution of the proteins tau (green) and MAP2 (orange) in a hippocampal nMAP2 staining is confined to the cell body and its dendrites. Organization of microtubule bundles by MAPs. (A) One end of MAP2 binds along the microtubule lattice and extends a long projecting arm. (B) Tau possesses a shorter projection arm. (C) Electron micrograph showing a cross section through a microtubule bundle in a cell overexpressing MAP2. The regular spacing of the microtubules (MTs) in this bundle results from the constant length of the projecting arms of MAP2. (D) Similar cross section through a microtubule bundle in a cell overexpressing tau. Here the microtubules are spaced more closely together than they Microtubule branching by augmin. (A) Augmin - tubulin ring complex that nucleates a new microtubule with a low branching angle. (B) Fluorescence micrographs showing augmin (orange) nucleating a microtubule branch in the cortex of an epidermal cell in the plant Arabidopsis thaliana. (C) Depletion of augmin severely stunts plant growth The effects of proteins that bind to microtubule ends. The transition between microtubule growth and shrinkage is controlled in cells by a variety of proteins. Catastrophe factors such as kinesin-13, a member of the kinesin motor protein superfamily, bind to microtubule ends and pry them apart, thereby promoting depolymerization. On the other hand, a MAP such as XMAP215 promotes rapid microtubule polymerization. XMAP215 binds tubulin dimers and delivers them to the microtubule plus end, thereby increasing the microtubule growth rate. Frames from a fluorescence time-lapse movie of the edge of a cell expressing fluorescently labeled tubulin that incorporates into microtubules (green) as well as the +TIP protein EB1 tagged with a different color (red). The same microtubule is marked (asterisk) in successive movie frames. When the microtubule is growing (frames 1, 2), EB1 is associated with the tip. When the microtubule undergoes a catastrophe and begins shrinking, EB1 is lost (frames 3, 4). The labeled EB1 is regained when growth of the microtubule is rescued (frame 5) Sequestration of tubulin by stathmin. Structural studies with electron microscopy and crystallography suggest that the elongated stathmin protein binds along the side of two tubulin heterodimers Microtubule severing by katanin can destabilize or amplify microtubules. (A) Taxol-stabilized, fluorescently labeled microtubules were adsorbed on the surface of a glass slide, to which purified katanin was added along with ATP. There are a few breaks in the microtubules 30 seconds after the addition of katanin. (B) Three minutes after the addition of katanin, the filaments have been severed in many places, leaving a series of small fragments at the previous locations of the long microtubules. (C) Incorporation of GTP-tubulin subunits from the soluble pool into sites of katanin-induced damage in the microtubule lattice stabilizes the severed end or generates an island of GTP-tubulin that promotes rescue. MICROTUBULES + Y-TuRC augmin nucleates assembly and centrosome nucleates microtubule remains associated with branching t h e minus end +¥ stathmin +TIPs binds subunits, aß-tubulin dimers associate with growing plus ends prevents assembly a n d can link t h e m to other structure s, such as m e m b r a n e s MICROTUBULES - microtubule - + ХМА Р215 stabilizes plus ends, kinesin-13 promotes rapid microtubule + growth induces catastrophe and disassembly + katanin MAPS severs microtubules stabilize microtubules by binding along sides filament bundling and cross-linking 170 tau MAP2 plectin links to i n t e r m e d i a t e fi l a m e n t s There are two major classes of microtubule-based motors, kinesins and dyneins, which perform three major functions: 1) they move cargo such as organelles and macromolecules within the cell. Unlike actin-based transport, however, microtubule-based motors are used to transport cargo over long distances. 2) motors can slide microtubules relative to one another, thereby generating specific arrangements of microtubules, as in neurons and epithelial cells and in the mitotic spindle. 3) a subset of microtubule-based motors regulates microtubule dynamics, as illustrated by kinesin-13 Kinesins As in the myosin superfamily, only the motor domains are conserved. Kinesin-1 has the motor domain at the N-terminus of the heavy chain and moves toward the microtubule plus end. The middle domain forms a long coiled-coil, mediating dimerization. The C-terminal domain forms a tail that attaches to cargo, such as a membrane-enclosed organelle. Kinesin-5 forms tetramers in which two dimers associate by their tails. The bipolar kinesin-5 tetramer is able to slide two microtubules past each other, analogous to the activity of the bipolar thick filaments formed by myosin II. Kinesin-13 has its motor domain located in the middle of the heavy chain. It is a member of a family of kinesins that have lost typical motor activity and instead bind to microtubule ends to promote depolymerization. Kinesin-14 is a C-terminal kinesin. Unlike most kinesins, Structures of four kinesin superfamily members members of the kinesin-14 family travel toward the microtubule minus end. Dyneins Cytoplasmic dynein. (A) Cryo-electron microscopy (cryoEM) reconstruction of a molecule of cytoplasmic dynein. Like myosin II and kinesin-1, cytoplasmic dynein is a two-headed molecule. The dynein head is very large compared with the head of either myosin or kinesin. (B) Schematic depiction of cytoplasmic dynein showing the two heavy chains that contain a motor head with domains for microtubule binding and ATP hydrolysis, connected by a long stalk. The tail domain consists of a linker that connects the motor heads to a dimerization domain. Bound to the linker domain are multiple intermediate chains and light chains (blue) C) The organization of domains in a dynein heavy chain. This is a huge polypeptide, containing more than 4000 amino acids. The conserved dynein motor head domain contains six AAA domains, four of which retain ATP-binding sequences, but only one of which has the major ATPase activity (brown). The tail domain is not as highly conserved as the head domain and varies among different dynein subtypes. The power stroke of dynein. Illustration of the movement of a monomeric axonemal dynein found in the flagellum of the unicellular green alga Chlamydomonas reinhardtii. As in cytoplasmic dynein, the motor-containing head domain of axonemal dynein connects to a long, coiled-coil stalk with the microtubule-binding site at the tip. The tail -swing, dynein- ATP binding and hydrolysis cause the linker to throw the head domain toward the microtubule minus end like a fishing hook. The microtubule-binding domain reattaches 8 nm along the microtubule. Release of ATP and phosphate then leads to a large conformational power stroke in the linker domain, pulling the tail and its attached microtubule toward the minus end. Each cycle generates a step of about 8 nm, thereby contributing to flagellar beating (see Figure 16 60). In the case of cytoplasmic dynein, the tail is attached to a cargo such as a vesicle, and a single power stroke transports the cargo about 8 nm along the microtubule toward its minus end (see Figure 16 56).