Cytoskeleton 23-65 PDF
Document Details
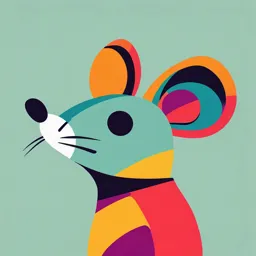
Uploaded by HonestChlorine9195
Koç University
Tags
Summary
This document discusses nucleotide hydrolysis and its effects on cytoskeletal polymers, focusing on actin filaments and treadmilling. It also covers chemical inhibitors of actin and microtubules.
Full Transcript
NUCLEOTIDE HYDROLYSIS Each actin molecule carries a tightly bound ATP molecule that is hydrolyzed to a tightly bound ADP molecule soon after its assembly into the polymer. Similarly, each tubulin molecule carries a tightly bound GTP molecule that si converted to a tightly bound GDP molecule soon aft...
NUCLEOTIDE HYDROLYSIS Each actin molecule carries a tightly bound ATP molecule that is hydrolyzed to a tightly bound ADP molecule soon after its assembly into the polymer. Similarly, each tubulin molecule carries a tightly bound GTP molecule that si converted to a tightly bound GDP molecule soon after the molecule assembles into the polymer. T( = monomer carrying ATP or G T P T T D (D = m o n o m e r carrying ADP free m o n o m e r subunit in polymer or G D P Hydrolysis of the bound nucleotide reduces the binding affinity of the subunit for neighboring subunits and makes it more likely to dissociate from each end of the filament. It is usually the T form that adds to the filament and the D form that leaves. Considering events at the plus end only: K T on D D D D D T D D D D D D off As before, the polymer will grow until C= C. For illustrative purposes, we can ignore T on and k off since they are usually very small, so that polymer growth ceases when K D off KoonnC= kf、 =ko or =C kkonon This is a steady state and not a true equilibrium, because the ATP or GTP that is hydrolyzed must be replenished by a nucleotide exchange reaction of the free subunit ( D → T ). DYNAMIC INSTABILITY a n d TREADMILLING a r e t w o b e h a v i o r s observed in cytoskeletal polymers. Both are associated with nucleoside triphosphate hydrolysis. Dynamic instability is believed to predominate in microtubules, whereas treadmilling may predominate in actin filaments. TREADMILLING One consequence of the nucleotide hydrolysis that accompanies polymer formation is to change the critical concentration at the two ends of the polymer. Because KDoff and KTon refer to different reactions, their ratio on need not be the same at both ends of the polymer, so that: C (minus end) >cC (plus end) Thus, fi both ends of a polymer are exposed, polymerization proceeds until the concentration of free monomer reaches a value that si above C, for the plus end but below C, for the minus end. At this steady state, subunits undergo a net assembly at the plus end and a net disassembly at the minus end at an identical rate. The polymer maintains a constant length, even though there is a net flux of subunits through the polymer, known as treadmilling. Subunits with bound ATP (T-form subunits) polymerize at both ends of a growing filament and then undergo ATP hydrolysis within the filament. As the filament grows, elongation is faster than hydrolysis at the plus end in this example, and the terminal subunits at this end are therefore always in the T form. However, hydrolysis is faster than elongation at the minus end, and so terminal subunits at this end are in the D form. Treadmilling occurs at intermediate concentrations of free subunits. The critical concentration for polymerization on a filament end in the T form, Cc(T), is lower than that for a filament end in the D form, Cc(D). If the actual subunit concentration is somewhere between these two values, the plus end grows while the minus end shrinks, resulting in treadmilling. The Functions of Actin Filaments Are Inhibited by Both Polymer-stabilizing and Polymer-destabilizing Chemicals TA B L E 1 6 - Chemical Inhibitors of Actin and Microtubules Effe ct o n Chemical fi l a m e n t s Mechanism Original source Actin Latruncul in Depolymerizes Binds actin subunits Spo nges Cytochalasin B Depolymerizes Caps filament plus ends Fungi Phalloidin Stabilizes Binds along filaments Amanita mushroom Microtubules Taxol (paclitaxel) Stabilizes Binds along filaments Yew tree Nocodazole Depolymerizes Binds tubulin subunits Synthetic Colchicine Depolymerizes Caps both filament ends Autumn crocus ACTIN FILAMENTS + formin Arp2/3 complex nucleates assembly and remains nucleates assembly to form associated with the growing a branched network and remains plus end associated with the minus end thymosin binds subunits, profilin prevents assembly bind s mon ome rs, + concentrates them at sites of filament assembly tropomodulin actin s u b u n i t s prevents assembly and disassembly at minus end ACTIN FILAMENTS + a c t i n fi l a m e n t + tropomyosin stabilizes filament, modulates cofilin binding of other accessory proteins binds ADP-actin filaments, accelerates disassembly gelsolin capping protein severs filaments and binds to plus end prevents assembly and disassembly at plus end filament bundling, cross-linking, and attachment to membranes plasma fi m b r i n mem brane a-actinin filamin spectrin ERM Some of the major accessory proteins of the actin cytoskeleton. Except for the myosin motor proteins, an example of each major type is shown. Each of these is discussed in the text. However, most cells contain more than a hundred different actin-binding proteins, and ti is likely that there are important types of actin-associated proteins that are not yet recognized. plus end plus end p l us e n d D, from.I Rouiller et al., J. Cell Biol. 180:887-895, 2008. © 2008A. Rouiller et al. Originally published ni J. Cel Biol. 180:887-895. https://doi.org/10.1083/jcb.200709092. With permission from Rockefeller (A) actin [*] Arp2 nucleation-promoting factor (NPF) other minus plus proteins end end + Arp3 Arp2 inactive actin n u c l e a t e d actin fi l a m e n t Arp2/3 complex active Arp2/3 complex monomers (B) (D) 25 n m daughter actin fi l a m e n t 70° accessory 1-7- proteins University Press. preexisting actin fi l a m e n t (E) (C) Once nucleated, rapid polymerization of actin depends on the further addition of actin monomers at the plus end of each filament. A key factor is the monomer-binding protein profilin. Profilin binds to the face of the actin monomer opposite the ATP-binding cleft, blocking the side of the monomer that would normally associate with the filament minus end, while leaving exposed the site on the monomer that binds to the plus end. When the profilin actin complex binds a free plus end, a conformational change in actin reduces its affinity for profilin and the profilin falls off, leaving the actin filament one subunit longer. The ActA protein on the bacterial surface activates the Arp2/3 complex to nucleate new filament assembly along the sides of existing filaments. Filaments grow at their plus end until capped by capping protein. Actin is recycled through the action of cofilin, which enhances depolymerization at the minus ends of the filaments. By this mechanism, polymerization is focused at the rear surface of the bacterium, propelling it forward bacteria in blue and actin filaments in red (A) Electron micrograph of a myosin II thick filament isolated from frog muscle. Note the central bare zone, which is free of head domains. (B) Schematic diagram, not drawn to scale. The myosin II molecules aggregate by means of their tail regions, with their heads projecting to the outside of the filament. The bare zone in the center of the filament consists entirely of myosin II tails. (C) A small section of a myosin II filament as reconstructed from electron micrographs. In the relaxed (noncontracting) state, the two heads of a myosin molecule are bent backward and sterically interfere with each other to switch off their activity. An individual myosin molecule in the inactive state is highlighted in green. The cytoplasmic myosin II filaments in non-muscle cells are much smaller, although similarly organized Figure 16 23 Direct evidence for the motor activity of the myosin head. In this experiment, purified myosin heads were attached to a glass slide, and then actin filaments labeled with fluorescent phalloidin were added and allowed to bind to the myosin heads. (A) When ATP was added, the actin filaments began to glide along the surface, owing to the many individual steps taken by each of the dozens of myosin heads bound to each filament. The video frames shown in this sequence were recorded about 0.6 second apart; the two actin filaments shown (red) were moving in opposite directions at a rate of about 4 /sec. (B) Diagram of the experiment. The large red arrows indicate the direction of actin filament movement (Movie 16.4). (A, courtesy of James Spudich.) Figure 16 25 The force of a single myosin molecule moving along an actin filament measured using an optical trap. (A) Schematic of the experiment, showing an actin filament with beads attached at both ends and held in place by focused beams of light called optical tweezers (Movie 16.6). The tweezers trap and move the bead and can also be used to measure the force exerted on the bead through the filament. In this experiment, the filament was positioned over another bead to which myosin II motors were attached, and the optical tweezers were used to determine the effects of myosin binding on movement of the actin filament. (B) These traces show filament movement in two separate experiments. Initially, when the actin filament is unattached to myosin, thermal motion of the filament produces noisy fluctuations in filament position. When a single myosin binds to the actin filament, thermal motion decreases abruptly, and a roughly 10-nm displacement results from movement of the filament by the motor. The motor then releases the filament. Because the ATP concentration is very low in this experiment, the myosin remains attached to the actin filament for much longer than it would in a muscle cell. (Adapted from C. et al., News Physiol. Sci. 17:213 218, 2002. With permission from the American Physiological Society.) Figure 16 26 Skeletal muscle cells (also called muscle fibers). (A) These huge multinucleated cells form by the fusion of many muscle cell precursors, called myoblasts. Here, a single muscle cell is depicted. In an adult human, a muscle cell is typically 50 in diameter and can be up to several centimeters long. (B) Fluorescence micrograph of rat muscle, showing the peripherally located nuclei (blue) in these giant cells. Myofibrils are stained red. (B, courtesy of Nancy L. Kedersha.) Figure 16 27 Skeletal muscle myofibrils. (A) Low-magnification electron micrograph of a longitudinal section through a skeletal muscle cell of a rabbit, showing the regular pattern of cross- striations. The cell contains many myofibrils aligned in parallel (see Figure 16 26). (B) Detail of the skeletal muscle shown in A, showing portions of two adjacent myofibrils and the definition of a sarcomere (black arrow). (C) Schematic diagram of a single sarcomere, showing the origin of the dark and light bands seen in the electron micrographs. The Z discs, at each end of the sarcomere, are attachment sites for the plus ends of actin filaments (thin filaments); the M line, or midline, is the location of proteins that link adjacent myosin II filaments (thick filaments) to one another. (D) When the sarcomere contracts, the actin and myosin filaments slide past one another without shortening. (A and B, courtesy of Roger Craig.) Figure 16 28 Electron micrographs of an insect flight muscle viewed in cross section. The myosin and actin filaments are packed together with almost crystalline regularity. Unlike their vertebrate counterparts, these myosin filaments have a hollow center, as seen in the enlargement on the right. The geometry of the hexagonal lattice is slightly different in vertebrate muscle. (From J. Auber and R. Couteaux, J. Microsc. 2:309 324, 1963. With Microscopie.) Figure 16 30 T tubules and the sarcoplasmic reticulum. (A) Drawing of the two membrane systems that relay the signal to contract from the muscle cell plasma membrane to all of the myofibrils in the cell. (B) Electron micrograph showing a cross section of a T tubule. Note the position of the large Ca2+-release channels in the sarcoplasmic reticulum membrane that connect to the adjacent T-tubule membrane. (C) Schematic diagram showing how a Ca2+-release channel in the sarcoplasmic reticulum membrane is thought to be opened by the activation of a voltage-gated Ca2+ channel in the membrane of the T tubule (Movie 16.7). (B, courtesy of Clara Franzini-Armstrong.) Figure 16 31A The control of skeletal muscle contraction by troponin. (A) A thin filament of a skeletal muscle cell, showing the positions of tropomyosin and troponin along the actin filament. Each tropomyosin molecule has seven evenly spaced regions with similar amino acid sequences, each of which is thought to bind to an actin subunit in the filament. (A, adapted from G.N. Phillips et al., J. Mol. Biol. 192:111 131, 1986.) Figure 16 32 Smooth muscle contraction. (A) Upon muscle stimulation by activation of cell-surface receptors, Ca2+ released into the cytoplasm from the sarcoplasmic reticulum (SR) binds to calmodulin (see Figure 15 34). Ca2+- bound calmodulin then binds myosin light-chain kinase (MLCK), which phosphorylates myosin light chain, stimulating myosin activity. Non-muscle myosin is regulated by the same mechanism (see Figure 16 34). (B) Smooth muscle cells in a cross section of cat intestinal wall. The outer layer of smooth muscle is oriented with the long axis of its cells extending parallel along the length of the intestine, and upon contraction will shorten the intestine. The inner layer is oriented circularly around the intestine and when contracted will cause the intestine to become narrower. Contraction of both layers squeezes material through the intestine, much like squeezing toothpaste out of a tube. (C) A model for the contractile apparatus in a smooth muscle cell, with bundles of contractile filaments containing actin and myosin (red) oriented obliquely to the long axis of the cell. Their contraction greatly shortens the cell. In this diagram, the bundle angles are exaggerated to schematically illustrate the effect of contraction. In addition, only a few of the many bundles are shown. (B, courtesy of Gwen V. Childs.)