Cytoskeleton 1-23 PDF
Document Details
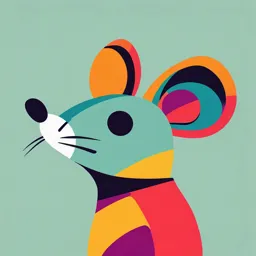
Uploaded by HonestChlorine9195
Koç University
Tags
Summary
This document provides an overview of the cytoskeleton, a complex network of protein filaments in cells. In cells, the cytoskeleton is responsible for structural support, cell shape, and intracellular transport. The different types of filaments (actin, microtubules, and intermediate) and their functions are detailed.
Full Transcript
A cell in culture has been fixed and labeled to show its cytoplasmic arrays of microtubules (green) and actin filaments (red). This dividing cell has been labeled to show its spindle microtubules (green) and surrounding cage of intermediate filaments (red). cell cortex MICROTUBULES...
A cell in culture has been fixed and labeled to show its cytoplasmic arrays of microtubules (green) and actin filaments (red). This dividing cell has been labeled to show its spindle microtubules (green) and surrounding cage of intermediate filaments (red). cell cortex MICROTUBULES 100 n m ili 25 n m Microtubules are long, hollow cylinders made of the protein tubulin. With an outer diameter of 25 nm, they are much more rigid than actin filaments. Microtubules are long and straight and frequently have one end attached to a microtubule-organizing center (MTOC) called a centrosome. (i) Single microtubule; (ii) cross section at the base of three cilia showing triplet microtubules; (iti) interphase microtubule array (green) and organelles (red); (iv) Micrographs courtesy of R. Wade (i); DT.. Woodrow and RW.. Linck (ii);.J Seemann (iii); ciliated protozoan.. Burnette (iv) D INTERMEDIATE FILAMENTS 100 mm 25 nm Intermediate filaments are ropelike fibers with a diameter of about 10 nm; they are made of intermediate filament proteins, which constitute a large and heterogeneous family. One type of intermediate filament forms a meshwork called the nuclear lamina just beneath the inner nuclear membrane. Other types extend across the cytoplasm, giving cells mechanical strength. In an epithelial tissue, they span the cytoplasm from one cell-cell junction to another, thereby strengthening the entire epithelium. (i) Individual intermediate filaments; (ii) Intermediate filaments (blue) in neurons and (ili) epithelial cell; (iv) nuclear lamina. Large-scale cytoskeletal structures can change or persist, according to need, lasting for lengths of time ranging from macromolecular components that make up these structures are in a constant state of flux. As a result, a structural rearrangement in a cell requires little extra energy when conditions change. For example, actin filaments form many types of cell- surface projections. Some of these are dynamic structures, such as the filopodia, lamellipodia, and pseudopodia that cells use to explore territory and move around. Motility is initiated by an actin-dependent protrusion of the leading edge, which is composed of lamellipodia and filopodia. These protrusive structures contain actin filaments, with elongating barbed ends orientated towards the plasma membrane time 0 min 1 min 2 min 3 min From a video recorded by David Rogers 15 um A neutrophil in pursuit of bacteria. In this preparation of human blood, a small clump of bacteria (white arrow) is about to be captured by a neutrophil. As the bacteria move, the neutrophil quickly reassembles the dense actin network within a pseudopod ("false foot") at its leading edge (highlighted in red) to push toward the location of the bacteria (Movie 16.1). Rapid disassembly and reassembly of the actin cytoskeleton ni this cell enable ti to change its orientation and direction of movement within a few minutes. (From a video recorded by David Rogers.) The regulation of actin filament formation is an important mechanism by which cells control their shape and movement. Actin subunits can spontaneously bind one another, but the association is unstable until subunits assemble into an initial oligomer, or nucleus, that is stabilized by multiple subunit subunit contacts and can then elongate rapidly by addition of more subunits. This process is called filament nucleation. N U C L E AT I O N A helical polymer is stabilized by multiple contacts between adjacent subunits. In the case of actin, two actin molecules bind relatively weakly to each other, but addition of a third actin monomer to form a trimer makes the entire group m o r e stable. Further monomer addition can take place onto this trimer, which therefore acts as a nucleus for polymerization. For tubulin, the nucleus is larger and has a more complicated structure (possibly a ring of 13 or more tubulin molecules) —but the principle is t h e same. The assembly of a nucleus is relatively slow, which explains the lag phase seen during polymerization. The lag phase can be reduced or abolished entirely by adding premade nuclei, such as fragments of already polymerized microtu bules or actin filament s. TIME COURSE OF POLYMERIZATION The ni vitro assembly of a protein into a long polymer such as a cytoskeletal filament typically shows the following time course: EQUILIBRIUM PHASE amou nt of polymer GROWTH LAG PHASE PHASE time — The lag phase corresponds ot time taken for nucleation. The growth phase occurs as monomers add to the exposed ends of the growing filament, causing filament elongation. The equilibrium phase, or steady state, si reached when the growth of the polymer due to monomer addition precisely balances the shrinkage of the polymer due to disassembly back to monomers. PLUS A N D M I N U S E N D S The two ends of an actin filament or microtubule polymerize at different rates. The fast-growing end is called the plus end, minus plus whereas the slow-growing end si called the minus end. The end end difference in the rates of growth at the two ends is made possible by changes in the conformation of each subunit as SLOW FAST it enters the polymer. free subun it in subunit polymer This conformational change affects the rates at which subunits add to the two ends. loss, which determines the equilibrium constant for its association Even though kon and kof wil have different values for the plus and with the end, is identical at both ends: if the plus end grows four minus ends of the polymer, their ratio Koffkon-and hence C-must be times faster than the minus end, it must also shrink four times the same at both ends for a simple polymerization reaction (no ATP or faster. Thus, for C> Ca both ends grow; for C< C, both ends GTP hydrolysis). This si because exactly the same subunit interactions shrink are broken when a subunit is lost at either end, and the final state of The nucleoside triphosphate hydrolysis that accompanies the subunit after dissociation si identical. Therefore, the AG for subunit actin and tubulin polymerization removes this constraint. NUCLEOTIDE HYDROLYSIS Each actin molecule carries a tightly bound ATP molecule that is hydrolyzed to a tightly bound ADP molecule soon after its assembly into the polymer. Similarly, each tubulin molecule carries a tightly bound GTP molecule that si converted to a tightly bound GDP molecule soon after the molecule assembles into the polymer. T( = monomer carrying ATP or G T P T T D (D = m o n o m e r carrying ADP free m o n o m e r subunit in polymer or G D P Hydrolysis of the bound nucleotide reduces the binding affinity of the subunit for neighboring subunits and makes it more likely to dissociate from each end of the filament. It is usually the T form that adds to the filament and the D form that leaves. Considering events at the plus end only: K T on D D D D D T D D D D D D off As before, the polymer will grow until C= C. For illustrative purposes, we can ignore T on and k off since they are usually very small, so that polymer growth ceases when K D off KoonnC= kf、 =ko or =C kkonon This is a steady state and not a true equilibrium, because the ATP or GTP that is hydrolyzed must be replenished by a nucleotide exchange reaction of the free subunit ( D → T ).