Cytopathology PDF
Document Details
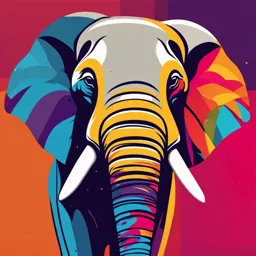
Uploaded by HearteningRelativity
University of Padua
Tags
Summary
This document provides a comprehensive overview of cytopathology, focusing on cell injury, adaptation, and diverse forms of cell death. It explores the mechanisms and consequences of cellular responses to various noxious stimuli, including hypoxia, physical agents, chemical agents, infection, and immunologic reactions.
Full Transcript
Table of Contents I. Introduction to Pathology and Cell Injury............................................................... 3 Steps in evolution of disease................................................................................................... 3 Causes of Cell Injury...................
Table of Contents I. Introduction to Pathology and Cell Injury............................................................... 3 Steps in evolution of disease................................................................................................... 3 Causes of Cell Injury............................................................................................................... 4 II. Cellular Responses to Stress and Noxious Stimuli................................................ 4 III. Cellular Adaptations........................................................................................... 5 1. Hypertrophy........................................................................................................................ 5 2. Hyperplasia........................................................................................................................ 6 3. Atrophy............................................................................................................................... 6 4. Metaplasia.......................................................................................................................... 6 IV. Mechanisms of Cell Injury and Death.................................................................. 7 1. Reversible Cell Injury........................................................................................................... 8 2. Irreversible Cell Injury and Cell Death................................................................................... 8 V. Intracellular and Extracellular Accumulations...................................................... 8 I. Overview of Necrosis........................................................................................... 10 What happens during ischemia?............................................................................................ 10 Ischemia survival of cells depends on their type..................................................................... 11 What happened during mitochondrial damage?..................................................................... 11 Necrosis and inflammation................................................................................................... 11 Infarction types..................................................................................................................... 12 II. Types of Necrosis............................................................................................... 12 1. Coagulative Necrosis........................................................................................................ 12 2. Liquefactive Necrosis........................................................................................................ 23 3. Fat Necrosis..................................................................................................................... 24 4. Caseous Necrosis............................................................................................................. 26 5. Fibrinoid Necrosis............................................................................................................. 27 6. Gangrenous Necrosis........................................................................................................ 29 7. Dystrophic calcification..................................................................................................... 30 IV. Comparative Summary of Necrosis Types.......................................................... 31 I. Introduction to cell death..................................................................................... 32 Evolution of Disease............................................................................................................. 32 Causes of Cell and Tissue Injury............................................................................................ 32 Cellular Response to Injury................................................................................................... 32 Progression of Cellular Injury................................................................................................. 33 Short Reversible Period After Injury........................................................................................ 33 Point of No Return in Cell Death............................................................................................ 33 II. Necrosis............................................................................................................ 33 Causes of Necrosis............................................................................................................... 34 Key Steps in Necrosis............................................................................................................ 34 Microscopic Changes........................................................................................................... 34 Comparison to Other Cell Death Types.................................................................................. 34 III. Apoptosis – programmed cell death................................................................... 34 When Apoptosis is Prominent................................................................................................ 35 Apoptosis............................................................................................................................. 35 The Two Major Apoptotic Pathways........................................................................................ 36 Caspases in Apoptosis.......................................................................................................... 38 Mitochondrial Outer membrane Permeabilization (MOMP) drives the intrinsic pathway........... 39 IV. Classic terms.................................................................................................... 43 V. Autophagy-dependent PCD................................................................................. 43 Cellular damage and adaptation I. Introduction to Pathology and Cell Injury Pathology: The study of structural, biochemical, and functional changes in cells, tissues, and organs underlying diseases. Pathology is divided in: General pathology: common reactions of cells and tissues to injurious stimuli, such reactions are not tissue specific (e.g. acute inflammation in response to bacterial infections produces a very similar reaction in most tissues) Systemic pathology: alterations and mechanism in diseases of particular organ systems Steps in evolution of disease 1. Etiology (causation): the study of the underlying causes and modifying factors responsible for disease initiation and progression. Can be grouped into two classes: o Genetic (e.g. inherited mutations) o Environmental (e.g. infectious) The idea that one etiologic agent is the cause of one disease arose from the study of infections and inherited disorders however the majority of diseases arise form effects of various environmental insults on a genetically susceptible individual (multifactorial). 2. Pathogenesis (molecular mechanisms): refers to the mechanisms of development and progression of disease. Basically, it explains how the underlying etiologies produce the morphologic manifestations of the disease. New technological advances (omics technologies) aim to explain pathogenic mechanism in a better way and to identify biomarkers that predict disease progression and therapeutic responses. 3. Morphologic changes (structural and functional alterations): refer to the structural alterations in cells or tissues that are characteristics of a disease and hance diagnostic of an etiological process. Traditionally, diagnostic pathology used morphology to determine the nature of disease and follow its progression however nowadays molecular analysis has been implemented since it can reveal genetic differences and predict responses to therapies. 4. Functional alterations (resulting clinical consequences): the end result of genetic, biochemical and structural changes in cells and tissues are functional abnormalities that lead to the clinical manifestations (symptoms and signs) of disease, as well as its progression (clinical course and outcome). Rudolph Virchow Father of modern pathology and social medicine Omnia cellula e cellula (every cell is derived from a [preexisting] cell “Disease arose, not in organs or tissues in general, but primarily in their individual cells” Causes of Cell Injury Hypoxia: A deficiency of oxygen that affects aerobic respiration. Causes include ischemia (reduced blood flow), inadequate oxygenation of the blood due to cardiorespiratory failure, reduced oxygen-carrying capacity of blood (e.g., anemia). Physical Agents: Trauma, temperature extremes, sudden changes in atmospheric pressure, electric shock and radiation. Chemical Agents and Drugs: Toxic substances like heavy metals and therapeutic drugs but also higher concentrations of simple chemicals such as glucose. Infectious Agents: From viruses to parasites each impact in their own way. Immunologic Reactions: Autoimmune reactions or hypersensitivity reactions. Genetic Abnormalities: Mutations that impair cell function because of deficient protein function or defects in inborn errors of metabolism or accumulation of damaged DNA. Polymorphisms can also influence the susceptibility of cells to injury by chemicals and other environment insults. Nutritional Imbalances: Deficiencies (e.g., protein-calorie malnutrition) or excesses (e.g., obesity) but also the composition of diets (e.g. atherosclerosis is very common in lipid rich diets). II. Cellular Responses to Stress and Noxious Stimuli 1. Homeostasis: Cells maintain a steady state of function and structure though adaptations. These are reversible functional and structural responses to physiological changes and can be divided into: o Hypertrophy is the increase of size o Hyperplasia is the increase in cell number o Atrophy is the decrease in the size and metabolic activity of cells o Metaplasia is the change in the phenotype pf cells 2. However, beyond a certain threshold, stress can lead to cell injury. o Reversible Injury: Cells can repair themselves if the stressor is mild or transient. o Irreversible Injury: Severe, persistent stress leads to cell death through necrosis or apoptosis. Adaptation, reversible injury, and cell death may be stages of progressive impairment For instance, in response to increased hemodynamic loads: 1. the heart muscle becomes enlarged, a form of adaptation, which leads to increased metabolic demands 2. if the blood supply to the myocardium is compromised or inadequate, the muscle first suffers reversible injury 3. Unless the blood supply is quickly restored, the cells suffer irreversible injury and die. Cell death occurs just for pathological reasons? No, the removal of damaged and aged cells through cell death is a normal and essential process in embryogenesis, the development of organs, and the maintenance of homeostasis into adulthood. However, excessive cell death as a result of progressive injury is one of the most crucial events in the evolution of disease in any tissue or organ. It results from diverse causes, including ischemia (reduced blood flow), infection, and toxins. Stresses of different types may induce changes in cells and tissues other than typical adaptations, cell injury, and death. Three other processes that affect cells and tissues are for example: intracellular accumulations, pathologic calcification and the normal process of aging. III. Cellular Adaptations Adaptations are reversible changes in cell function or structure in response to changes in their environment. These can be: o physiological (normal), responses of cells to normal stimulation by hormones or endogenous chemical mediators. o pathological (in response to harmful stress), allow cells to escape injury, but at the expense of normal function. 1. Hypertrophy Definition: An increase in cell size leading to an increase in organ size, typically as a response to increased workload. Mechanism: it is induced by activation of growth factors and transcription factors by mechanical sensors and occurs in tissues incapable of cell division. o Physiological Examples: § Muscular hypertrophy due to increased physical activity. § Uterine hypertrophy during pregnancy, influenced by estrogen. § Kidney size increase due to loss of the other kidney. o Pathological Examples: § Cardiac hypertrophy due to hypertension or aortic valve disease. This condition results from increased hemodynamic stress, causing the heart to work harder. § Goiter and thyroidea due to iodine deficiency 2. Hyperplasia Definition: An increase in the number of cells due to hormonal or compensatory stimuli. Occurs in tissues whose cells are able to divide. It may occur concurrently with hypertrophy. Mechanism: It is the result of growth factor–driven proliferation of mature cells and, in some cases, by increased output of new cells from tissue stem cells. o Physiological Hyperplasia: occurs when there is a need to increase functional capacity of hormone sensitive organs, or when there is need for compensatory increase after damage or resection. § Hormonal: Occurs in the glandular epithelium of the female breast during puberty and pregnancy. § Compensatory: Hyperplasia of liver hepatocytes following partial hepatectomy. o Pathological Hyperplasia: caused by excessive or inappropriate actions of hormones or growth factors acting on target cells. § Hormonal: hyperplasia of the endometrium due to excessive estrogen stimulation (higher risk of cancer or viral infections like papilloma). 3. Atrophy Definition: A decrease in cell size and organ size, resulting from decreased nutrient supply or disuse. It is associated with decreased synthesis of cellular building blocks and increased breakdown of cellular organelles and autophagy. Mechanism: Atrophy results from decreased protein synthesis and increased protein degradation in cells through ubiquitin-proteasome pathway and autophagy (residual bodies remain as lipofuscin granules). o Physiological Atrophy: Occurs during fetal development. o Pathological Atrophy: Results from factors such as reduced activity, denervation, inadequate nutrition, reduced blood flow, tissue compression or loss of endocrine stimulation. However, the most important cause of atrophy is aging. 4. Metaplasia Definition: A reversible change where one adult cell type is replaced by another type to better withstand adverse conditions. Mechanism: does not result from a change in the phenotype of an already differentiated cell type; rather, it results from either reprogramming of local tissue stem cells or, alternatively, colonization by differentiated cell populations from adjacent sites. o Example: Squamous metaplasia in the respiratory tract due to chronic smoking, where columnar epithelium is replaced by squamous epithelium (more durable but less protective against infection, also predispose to cancer development). o Pathological Implications: Metaplasia may reduce cellular function and predispose tissues to malignant transformation, as seen in Barrett's esophagus where esophagus epithelium changes to cells that look like intestinal cells due to stomach contents reflux. This is associated with cancer development (adenocarcinoma). o Connective tissue metaplasia: formation of cartilage, bone or adipose cell in tissues that normally do not contain these elements (e.g. myositis ossificans). No association with increased risk of cancer. Cell adaptation predispose to pancreatic cancer KRAS mutations Injury leads to some structural Injury combined with KRAS alone (identified though changes: mutations leads to sequencing) maintain acinar dedifferentiation pronounced tissue homeostasis but do not cause meaning they lost their disorganization and the significant tissue disruption. specialized potential onset of pancreatic characteristics cancer, illustrating how cell All characteristics of fibroblast activation due adaptations, along with pancreatic tissue maintained: to a fibrotic response genetic mutations, Acinar structure infiltration of the immune predispose tissues to Ducts system malignancy. The acinar cells Islets of Langerhans However, this does not cause no longer resemble their severe damage unless original form, and the tissue combined with mutations. appears highly disorganized. IV. Mechanisms of Cell Injury and Death Cell injury usually results from functional and biochemical abnormalities in one or more of a limited number of essential cellular components. Hypoxia and ischemia: ATP depletion and failure of many energy-dependent functions resulting initially in reversible cell injury (cell and organelle swelling) and later in cell death by necrosis. In ischemia-reperfusion injury: restoration of blood flow to an ischemic tissue can lead to: o Oxidative stress: accumulation of ROS, which can damage cellular lipids, proteins (e.g. misfolding), and DNA (e.g. apoptosis pathway activation). oInflammation is associated with cell injury because of the damaging actions of the products of inflammatory leukocytes. Chemical Injury o Direct toxicity: some chemicals injure cells directly by combining with critical molecular components. o Conversion to toxic metabolites: most toxic chemicals are not biologically active in their native form but must be converted to reactive toxic metabolites which then act on target molecules. 1. Reversible Cell Injury Reversible Cell injury is characterized by functional and structural alterations in early stages or mild forms of injury, which are correctable if the damaging stimulus is removed. Two features: Generalized swelling of the cell and its organelles: o Blebbing plasma membrane o Detachment of ribosomes from the ER o Clumping of nuclear chromatin Fatty change: occurs in organs that are actively involved in lipid metabolism (e.g. liver). 2. Irreversible Cell Injury and Cell Death When the extent of damage to critical cellular components becomes so severe that the cell can no longer repair itself or maintain homeostasis cell death occurs, and it can either be through: Necrosis: A pathologic process resulting from severe cell damage which leads to an inflammatory response. Apoptosis: A regulated, energy-dependent process by which cells die without eliciting an inflammatory response. V. Intracellular and Extracellular Accumulations Intracellular Accumulations: o Reversible: § Hydropic changes (acute intracellular edema), identifiable because of the round shape of the cells with a central nucleus. § Fatty changes (steatosis), identifiable because hepatocytes look bigger compared to normal ones due to the increased amount of lipids inside them. § Main causes of steatosis are alcohol 1 , malnutrition, obesity, diabetes mellitus and liver toxins o Irreversible: Accumulation of pigments, proteins, and cholesterol. § Accumulation of protein is also called Mallory body and has a glossy appearance. A) Steatosis: fatty acid accumulation B) Hydropic changes: central nucleus C) Mallory body: protein accumulation Steatosis and hepatitis are reversible conditions so the liver can go back to normal through abstinence however continued exposure or repeated attacks lead to cirrhosis which is an irreversible condition. Extracellular Accumulations o Pathological Calcification: caused by increased secretion of parathyroid hormone by parathyroid tumors; destruction of bone; vitamin D–related disorders; and renal failure, in which phosphate retention leads to secondary hyperparathyroidism. § Dystrophic Calcification: Occurs in areas of necrosis due to increased binding of phosphates with necrotic and degenerative tissue and later forming calcium phosphate precipitates (generally irreversible). § Metastatic Calcification: Occurs in normal tissues with hypercalcemia due to increased precipitates of calcium phosphate (reversible upon correction of metabolic disorder). 1 Due to alcohol metabolism a great amount of reducing equivalents is produced and later stored as lipids Necrosis I. Overview of Necrosis Definition: Necrosis is the sequence of morphological changes following irreversible cell injury, resulting in the death of cells within living tissues. It is often accompanied by inflammation and tissue breakdown. Pathogenesis: o Triggered by physical damage, hypoxia, ischemia, chemical agents, or infections. o Leads to disruption of cellular structures and eventual cell lysis. Two important definitions Infarction: an area of ischemic necrosis caused by occlusion of either the arterial supply or venous drainage in a particular tissue (85-90% of all infarcts are due to arterial thrombotic or embolic events) Embolism: a mass carried by the blood or lymphatic stream usually formed in vivo by components in the blood. An embolus usually consists of thrombus material (= a blood clot), but in rare cases it can be a mass of fat, cholesterol, bone marrow, cotton tissue… What happens during ischemia? Ischemia is a reduction in blood flow to tissues (e.g. due to an embolus), leading to decreased oxygen supply. Without oxygen, mitochondria cannot perform oxidative phosphorylation effectively leading to a reduction of ATP production. This energy deficiency disrupts cellular processes that depend on ATP: 1. Na⁺ Pump Failure: which normally maintains ion gradients. This failure leads to: o Influx of Ca²⁺, H₂O, and Na⁺: These ions enter the cell, causing swelling. o Efflux of K⁺: Potassium leaves the cell. o ER Swelling and Cellular Swelling: The influx of water and ions causes swelling of the endoplasmic reticulum and the entire cell, which can lead to membrane blebbing and loss of microvilli. 2. Anaerobic Glycolysis: once oxidative phosphorylation is compromised, cells switch to anaerobic glycolysis for energy: o Glycogen Depletion: Cellular glycogen stores are used up quickly. o Lactic Acid Accumulation: Anaerobic glycolysis produces lactic acid, which lowers intracellular pH. o pH Drop: The acidic environment leads to chromatin clumping in the nucleus. 3. Protein Synthesis Impairment: ATP depletion also causes ribosome detachment from the rough endoplasmic reticulum, reducing protein synthesis. Ischemia survival of cells depends on their type Cardiac myocytes survive 30 minutes without blood supply Neurons survive 3-4 minutes without blood supply Hepatocytes survive 2.5 hours without blood supply What happened during mitochondrial damage? Mitochondrial Damage or Dysfunction: Other factors like toxins, radiation, or oxygen deprivation damage mitochondria. Decreased ATP Generation and Increased ROS: Damaged mitochondria produce less ATP and generate more reactive oxygen species (ROS), which can damage cellular components. Cell Necrosis: These disruptions lead to multiple cellular abnormalities, ultimately resulting in necrosis, a type of cell death associated with inflammation and tissue damage. Necrosis and inflammation In the context of cellular injury and necrosis, dying cells release specific molecules known as DAMPs (damage-associated molecular patterns). These molecules are recognized by the immune system as signals of cellular damage, triggering an inflammatory response. Key Points on DAMPs and Their Effects 1. Release of DAMPs from Dying Cells: o ATP from Mitochondria: As cells die, ATP, usually confined within mitochondria, leaks out. o Uric Acid and Uridine Triphosphate (UTP): When DNA breaks down, uric acid and UTP are released. 2. Activation of the Immune System: o Pro-inflammatory Cytokines: The release of DAMPs activates macrophages and other immune cells, prompting them to produce pro-inflammatory cytokines. These cytokines mediate the inflammation process, attracting additional immune cells to the site of injury. o Troponin as a Biomarker: In cases of heart injury, such as a heart attack, dying cardiac muscle cells release troponin, a protein that can be measured in blood. High troponin levels are indicative of cardiac muscle damage. 3. Reactive Oxygen Species (ROS): o Hydrogen Peroxide (H₂O₂): This ROS is highly reactive and contributes to cellular damage and oxidative stress. ROS can be released by damaged cells, exacerbating inflammation and cell death. 4. Chemokines and Immune Cell Recruitment: o CXC Chemokines: These chemokines primarily attract neutrophils to the site of injury. Neutrophils are a type of white blood cell involved in the early phases of inflammation. o CC Chemokines: These chemokines mainly recruit monocytes and lymphocytes (white blood cells involved in both the immediate and adaptive immune responses) to the damaged area. Together, these responses form an immune reaction aimed at clearing damaged cells and debris, repairing tissues, and preventing infection. However, excessive or chronic release of DAMPs and the resulting inflammation can lead to tissue damage and contribute to diseases associated with chronic inflammation. Infarction types Anemic (pale) infarcts Hemorrhagic (red) infarcts Caused by arterial occlusion Caused by arterial or venous occlusion Coagulative necrosis Coagulative necrosis Sharply outlined and paler than surrounding Sharply outlined, dark red to purple or tissue brownish Often a hemorrhagic border zone, but no Bleeding in the necrotic area from surrounding bleeding into the necrotic area arteries and veins Seen in lungs (dual blood supply), intestines Typical in heart, kidney, brain (when there is no (numerous collaterals), brain (spontaneous bleeding into the infarct) and spleen thrombolysis and reperfusion of the ischemic area) II. Types of Necrosis 1. Coagulative Necrosis Features: o Preserved tissue architecture for a few days. o Cells show eosinophilia, loss of nuclei (karyolitic), and a glassy appearance. Mechanism: o Protein denaturation predominates over enzymatic digestion. o Dead cells persist until inflammatory cells remove them. Common Causes: o Ischemic injury in solid organs except the brain. Examples: o Myocardial infarction (red or pale). o Renal infarcts. Myocardial infarction Occurs when blood flow through one of the coronary arteries is blocked, usually by a blood clot or a ruptured atherosclerotic plaque. This blockage causes ischemia (lack of oxygen) in the heart tissue. The heart muscle (myocardium), especially the muscle cells (myocytes), becomes deprived of oxygen, which leads to cell injury and death. Progression of Cellular Damage 1. Normal Myocyte: The top image shows a healthy cardiac muscle cell with an intact nucleus and no damage. 2. Reversible Injury: Under ischemic conditions, the myocyte initially undergoes reversible injury. Cells may swell, and metabolic disturbances (such as accumulation of lactic acid) begin, but they can potentially recover if blood flow is restored. 3. Irreversible Injury and Necrosis: If ischemia persists, myocytes die through a process known as coagulative necrosis. In this stage: o Nucleus Breakdown: The nucleus becomes fragmented, and cells lose their structure. o Release of Intracellular Contents: Dead myocytes release substances (e.g., troponin) into the bloodstream, which can be measured to confirm MI. o Scar Formation: Over time, dead tissue is replaced by collagen type I, forming scar tissue, which lacks the contractile ability of normal muscle. Macroscopic Anatomy and Progression of Infarction 1. Initial Blockage: The blockage typically occurs in one of the coronary arteries (left anterior descending, right coronary, or left circumflex coronary artery). When an artery is blocked, the area supplied by it becomes the “zone of perfusion” or "area at risk." 2. Zone of Necrosis: o Early Hours (0-2 hours): Initially, ischemic changes are confined to the innermost layer (subendocardium) of the heart wall. o 24 Hours Post-occlusion: Without intervention, the necrosis extends through more layers of the myocardium. Eventually, the entire thickness (transmural infarction) becomes necrotic. 3. After Days to Weeks: o Collagen and Scar Formation: Over time, necrotic tissue is replaced with fibrous scar tissue, composed of collagen type I. This scar tissue doesn’t function like normal heart muscle and can impair the heart’s ability to pump effectively. o Marbling and Mural Thrombus: Sometimes, “marbling” (a pattern due to hemorrhage and inflammation) can be seen in the affected tissue, indicating infarction a few days old. Blood clots (mural thrombi) may also form along the damaged endocardial surface, increasing the risk of embolism. 4. Likelihood of Rupture: In some cases, weakened myocardial tissue may rupture, particularly when scar tissue hasn't fully formed. This rupture can lead to sudden death if it occurs in a critical structure like the ventricular wall. Impact of Infarct Location and Size Proximal vs. Distal Blockage: If the occlusion is in a proximal part of a major artery, the infarct area is large, and the damage is extensive. A distal blockage affects a smaller portion of the myocardium, increasing the chances of survival. End-Artery Occlusion: Coronary arteries are end-arteries, meaning they don’t have significant alternative pathways (collaterals) for blood flow. Thus, a blockage typically leads to infarction in the downstream myocardium unless new blood vessels have formed (collaterals) over time. Cross-Section of Heart, Left Ventricle with Epicardial Fat This image highlights a section of the heart with visible pathological changes. Key points include: Pale (anemic) infarct: this area corresponds to coagulative necrosis, where the tissue appears frozen in time due to cell death, preserving the structural outline. Neighboring zone of hyperemia: Surrounding the infarct is a hyperemic zone, where expanded vessels are evident due to vascular dilation. This zone is indicative of an inflammatory response, with neutrophils infiltrating the area to phagocytize and clear the debris of dead cells. Myocardial Infarction: Macroscopic Anatomy Progression The process of myocardial infarction evolves over time, starting with initial ischemic injury and progressing to fibrosis and scar formation. 0–12 hours: No visible changes observed at this stage. Cellular injury occurs at a molecular level. 24 hours: Infarcted area begins to appear pale, marking the onset of coagulative necrosis. 3–4 days: The infarcted region becomes marbled and sharply outlined, with a central yellowish, necrotic zone surrounded by a hyperemic border (vascular dilation). Neutrophils infiltrate to clear cellular debris. 2–3 weeks: The tissue becomes soft and gelatinous, with hemorrhagic changes due to macrophage activity and granulation tissue formation. Months: Healing progresses with the deposition of collagen type I, leading to fibrosis and the formation of scar tissue. Clinical Insight: Many patients survive initial heart attacks; however, repeated infarctions (fourth or fifth) significantly increase the likelihood of mortality due to cumulative damage and reduced cardiac function. Histological Changes in Myocardial Infarction Progression Normal Myocardium (Baseline): Healthy tissue with visible striations and intact nuclei. Cells are organized, and the architecture is well-preserved. 12–18 Hours Post-Ischemia: The tissue begins to show damage. Hematoxylin and eosin (H&E) staining reveals redder tissue. Nuclei undergo fragmentation (karyorrhexis, karyolysis), but the overall architecture is still recognizable. 1 Day Post-Ischemia: Neutrophil infiltration becomes evident, marking the inflammatory response. Architecture starts to break down, making it harder to distinguish intact structures. 3 Weeks Post-Ischemia: Granulation tissue forms, making the architecture almost unrecognizable. Features include: o New blood vessels: Indicating angiogenesis. o Macrophages and fibroblasts: These elongated cells secrete collagen, contributing to scar formation. o Endothelial cells and T-cells: Supporting the inflammatory and repair processes. 3 Months Post-Ischemia (Long-Term Changes): Scar tissue replaces damaged myocardium. Scar tissue is rigid, significantly impacting the heart’s function. Hypertrophy of surrounding myocardium may occur to compensate for reduced pumping ability, sometimes leading to the heart doubling in size. Clinical Implications: Scar tissue lacks the contractile properties of normal myocardium, permanently reducing heart function. Over time, compensatory hypertrophy increases the risk of further cardiac dysfunction or heart failure. Microscopic Progression of Myocardial Infarction 1 Day (Wavy Fibers and Early Necrosis): Fibers appear wavy due to the loss of contractile function following ischemia. Infarct Formation: The myocardium shows early coagulative necrosis. Some nuclei are still visible, but others are fragmented or undergoing karyolysis, appearing as “ghost nuclei.” 2–3 Days (Neutrophilic Infiltration): The tissue architecture is still somewhat recognizable. A significant infiltration of neutrophils marks the inflammatory response. The nuclei are more faded and fragmented as necrosis progresses. 7–10 Days (Granulation Tissue and Edema): Granulation Tissue Formation: Macrophages replace neutrophils, clearing cellular debris. Fibroblasts start to proliferate, laying down the extracellular matrix. Edema may be observed in the affected area. 2–3 Weeks (Neoangiogenesis): Formation of new blood vessels occurs as part of the repair process. Proliferation of endothelial cells is visible under the microscope. Bluish staining indicates the presence of collagen, produced by fibroblasts, which contributes to scar formation. 3 Months (Scar Tissue Formation): The myocardium is replaced with dense, fibrous scar tissue rich in collagen. This scar is rigid and non-contractile, permanently impairing heart function. Hypertrophy of the remaining myocardium may occur to compensate for the loss of functional tissue. Clinical Implications: These histological changes are sequential and can be used to estimate the timeline of a myocardial infarction. The transition from granulation tissue to scar tissue marks the point of irreversible damage, leading to long-term functional deficits. ⚠ Probable exam picture 1: Acute Histological Changes in Myocardial Infarction This image demonstrates very acute changes in myocardial tissue, consistent with early stages of a myocardial infarction (1–2 days post-event). Key observations include: Coagulative Necrosis: The overall architecture of the myocardium is still recognizable, indicative of coagulative necrosis. The structural integrity of the tissue remains largely intact, although the cells are no longer viable. Karyolysis: The nuclei are absent or appear faint due to progressive breakdown (karyolysis). This is a hallmark of necrotic cell death. Inflammatory Response (Limited): There is no massive influx of inflammatory cells like neutrophils yet, suggesting the infarct is still in the early stages (within 1–2 days). ⚠ Probable exam picture 2: Granulation Tissue (~2 Weeks Post-Myocardial Infarction) If this image appears in your exam, here’s what to note: No Coagulative Necrosis: The tissue architecture is no longer recognizable, indicating the loss of structural integrity that typically follows the inflammatory response and clearance of necrotic debris. Granulation Tissue Formation: The hallmark feature here is granulation tissue, a critical component of wound healing. Key elements include: Newly Formed Blood Vessels: Evidence of neoangiogenesis, essential for delivering nutrients and oxygen to the healing area. Numerous Erythrocytes: Red blood cells within the new vessels indicate active blood supply. Inflammatory and Repair Cells: High cellularity is evident, with fibroblasts, macrophages, and T-cells actively participating in the repair process. Fibroblasts: Secrete collagen to lay the groundwork for scar formation. Macrophages: Clear remaining debris and stimulate tissue repair. Clinical Relevance: This tissue is very fragile, and excessive strain or exercise during this stage can disrupt the healing process. Patients are advised to rest and avoid physical exertion for a prolonged period post-infarction to allow stable scar formation. ⚠ Probable exam picture 3: Scar Tissue in Myocardial Infarction If this image is shown in your exam, here’s a description: Fibrotic Tissue Formation: The image represents scar tissue, which is the end stage of myocardial healing after an infarction. The original myocardium has been replaced by dense, collagen-rich fibrotic tissue, as indicated by the loss of myocardial architecture and the uniform, fibrotic staining. Loss of Function: While the patient can survive with this tissue, scar tissue lacks the contractile properties of normal myocardium. This inevitably leads to a reduction in pumping efficiency, which may progress over time, increasing the risk of heart failure. Long-Term Impact: The surrounding myocardial tissue may undergo compensatory hypertrophy to maintain cardiac output, which could further strain the heart over time. Patients with significant scarring often require long-term medical management and lifestyle modifications Transmural Myocardial Infarction: Complication - Ventricular Rupture 2–3 Weeks Post-Infarction: The heart wall is fragile due to granulation tissue, which lacks the structural integrity of mature scar tissue. This stage is particularly high risk for ventricular rupture, especially in transmural infarctions. Rupture and Hemopericardium: Ventricular Wall Rupture: The image on the left shows a rupture through the ventricular wall, leading to massive bleeding into the pericardial space. Hemopericardium (Right Image): Accumulation of blood within the pericardium due to the rupture. Although remembering the term "hemopericardium" isn’t necessary, understanding its consequence is: it can cause cardiac tamponade, a fatal compression of the heart. Clinical Significance: Very few patients survive this complication due to the rapid onset of massive bleeding and cardiac dysfunction. It highlights the importance of early intervention and stabilization during the high-risk period post-infarction. Renal infarction Coagulative necrosis is the hallmark of renal infarction. The affected area appears pale due to the lack of blood supply and tissue death. Etiology could be: Occlusion of a renal artery or one of its branches Embolism from cardiac thrombus Embolism from heart valves in bacterial endocarditis Embolism from an atherosclerotic plaque in aorta Renal Infarction: Macroscopic Anatomy Type of Infarct: white (anemic) Infarct: This occurs due to the blockage of an end artery, which is the sole blood supply to this portion of the kidney. The infarcted area lacks blood supply, leading to pale tissue. Wedge-Shaped Appearance: The infarct area is typically wedge-shaped, with the base pointing toward the renal capsule and the apex toward the occluded artery. Coagulative Necrosis: The tissue structure within the infarct is preserved initially but is non-viable. On microscopic examination, the architecture of the tissue remains visible, indicative of coagulative necrosis. Hemorrhagic Border: Surrounding the infarct is a zone of hemorrhagic congestion, where blood vessels in adjacent viable tissue are dilated due to reactive hyperemia. Healing Process: Over time, the infarct heals by fibrosis, resulting in a depressed scar on the renal surface. This scar may undergo dystrophic calcification in some cases. Microscopic Appearance of White Kidney Infarct Sharp Demarcation Line: There is a clear boundary between healthy tissue and the infarcted area. The transition reflects the abrupt cessation of blood supply in the affected region. Coagulative Necrosis: The infarcted tissue shows preserved architecture, a hallmark of coagulative necrosis. Despite the loss of cellular function, the structural outline of cells remains intact. Neutrophil Infiltration: Neutrophils are arriving at the infarcted site, initiating the inflammatory response. Their presence is key to breaking down dead cells and preparing the tissue for eventual healing or scar formation. Clinical Implication: Such infarcts occur due to end-artery occlusion, cutting off blood supply completely. This is commonly associated with embolism or thrombus from conditions like cardiac valve disease or atherosclerosis. Acute Kidney Infarct: Histological Features Preserved Architecture: The glomerulus and tubules are still visible, maintaining their structural integrity, which is characteristic of coagulative necrosis. Absence of Nuclei: Cellular nuclei are absent due to karyolysis (nuclear dissolution), a hallmark of necrosis. This absence indicates that the cells are non-viable. No Neutrophil Infiltration Yet: The absence of neutrophils suggests that this is an early stage of infarction, preceding the inflammatory response. White Infarct Characteristics: Despite appearing red under the microscope, this is a white (anemic) infarct due to a lack of erythrocytes in the affected region. The red staining is caused by eosin, which binds strongly to proteins in necrotic cells. The acidic environment from ischemia (due to lactic acid accumulation) enhances eosin binding, intensifying the staining. White vs. Red Infarct: White Infarct: Lack of erythrocytes in the affected area (as seen here). Red Infarct: Presence of erythrocytes, typically due to venous congestion or collateral circulation. Mechanism of Coagulative Necrosis: Ischemia leads to reduced ATP production due to impaired oxidative phosphorylation. Anaerobic glycolysis increases, producing lactic acid and lowering the pH. Proteins in dying cells bind eosin (negatively charged), leading to the intense red staining. Intestinal Infarction: Red Infarcts with Coagulative Necrosis Occurs due to venous obstruction or congestion, which allows blood to pool in the affected region. Commonly leads to coagulative necrosis, with preserved architecture in the early stages. Etiology: 50%: embolism or thrombus in the superior mesenteric artery, which supplies the majority of the small intestine. 25%: embolism or thrombus in the inferior mesenteric artery, or thrombosis in the superior mesenteric vein (commonly associated with stasis or inflammation). 25%: No vascular occlusion is identified, often caused by twisting of the bowel (e.g., volvulus), leading to ischemia. Collateral Circulation and Red Infarcts Dual Blood Supply: Organs like the intestines receive blood from multiple arteries. If one artery is blocked, collateral circulation compensates partially. Red Infarct Formation: Inadequate collateral blood flow leads to ischemia, necrosis, and hemorrhage from leaking vessels, resulting in a red appearance. Histologically, coagulative necrosis is seen with neutrophil infiltration. Clinical Relevance: Often linked to mesenteric artery occlusion, presenting as pain, inflammation, and potential bowel necrosis. Acute Hemorrhagic Infarct of Intestine Appearance: Dark, hemorrhagic discoloration of the intestinal wall. Severity: Rare but life-threatening, with a 50-75% mortality rate. Often leads to sepsis, perforation, and systemic complications. Clinical Presentation: major cause of acute abdomen, requiring urgent surgical intervention. Symptoms may include severe abdominal pain, fever, and signs of shock. Hemorrhagic Infarct of the Small Intestine Extensive Hemorrhage: The reddish staining represents hemorrhage within the infarcted tissue, typical of a red infarct. Clusters of erythrocytes are visible throughout the tissue, especially with closer magnification. Neutrophil Infiltration: Dark spots indicate neutrophils, which are releasing enzymes to break down necrotic tissue. Necrosis Across Layers: Multiple layers of the intestinal wall show signs of coagulative necrosis. Lung Infarction: Red Infarcts with Coagulative Necrosis Red infarct caused by hemorrhage due to dual blood supply from the pulmonary and bronchial arteries. Commonly leads to coagulative necrosis, with preserved architecture in the early stages. Etiology could be represented by: 30% of all autopsies have thromboembolisms in the lungs. Smaller lung infarcts are clinically silent (no symptoms) The majority is embolisms from thrombi in the deep veins in the calf, thigh and pelvis (=deep veins in the leg) The rest is thromboembolisms from the right side of the heart Causes of pulmonary embolism Thromboembolism from the deep leg veins (more than 90 % of cases!) Tumor embolism Fatty embolism Bone marrow embolism Air embolism Amniotic fluid embolism Foreign body embolism (cotton wool, talcum powder) Schistosomiasis eggs (Bilharzia, snail fever, Trematoda) Deep Vein Thrombosis (DVT) Deep vein thrombosis (DVT) occurs when a blood clot forms in the deep veins of the leg, often near venous valves where blood flow is slower. The thrombus initially adheres to the vessel wall, creating a site of obstruction. Over time, part of the clot can break off, forming an embolus that travels through the venous system to the lungs, resulting in a pulmonary embolism (PE). PE often leads to lung infarction (red infarcts) and presents with symptoms such as sudden shortness of breath, chest pain, and potentially cardiovascular collapse. In some cases, the occlusion caused by the thrombus restricts blood flow to one leg, leading to significant swelling (edema), increased pressure, and pain. Severe cases of restricted blood flow can cause tissue death, requiring amputation of the affected limb. DVT and PE together form venous thromboembolism (VTE), with risk factors including immobility, surgery, malignancy, and hypercoagulable states. Early detection and intervention are crucial to prevent these serious complications. Deep vein thrombosis (DVT) can sometimes result in the formation of a large, atypical thrombus, such as the one shown in the image. This “lump” of coagulated blood adheres to the deep veins, often causing obstruction, swelling, and inflammation below the blockage site. If a thrombus of this size becomes dislodged, it can travel through the venous system to the right side of the heart and subsequently lodge in the pulmonary arteries as a massive embolus. This condition, known as a massive pulmonary embolism, is catastrophic, leading to sudden death within minutes due to acute obstruction of blood flow to the lungs. Early detection and management of DVT are critical to prevent such life-threatening complications. Pulmonary embolism This image illustrates a pulmonary embolism (PE), where a dislodged thrombus (often originating as part of a deep vein thrombosis (DVT)) has traveled to the lungs and caused a profound occlusion of the pulmonary arteries. Such emboli obstruct blood flow, leading to reduced oxygenation and increased pressure in the right side of the heart. Hemorrhagic lung infarct Hemorrhagic (red) pulmonary infarctions are a result of pulmonary embolism, where a thrombus obstructs blood flow in the pulmonary arteries, leading to ischemia and tissue necrosis. Due to the lung’s dual blood supply from the pulmonary and bronchial arteries, blood leaks into the affected area, giving the infarct its characteristic dark red appearance. These infarcts are typically infiltrated by neutrophils as part of the inflammatory response, and coagulative necrosis may develop, though it is often difficult to observe grossly in these cases. Upon microscopic examination, the infarcted area is filled with erythrocytes, characteristic of a red infarct, due to the lung’s dual blood supply from the pulmonary and bronchial arteries. The rupture of nearby healthy arteries contributes to significant bleeding into the infarcted tissue. This process results in the accumulation of blood and necrotic debris. In this histological view of a hemorrhagic lung infarction, erythrocytes are prominent, filling the alveolar spaces as a result of blood extravasation caused by vascular damage in a red infarct. Despite the extensive hemorrhage, the alveolar walls remain recognizable, a hallmark of coagulative necrosis, where the tissue architecture is preserved despite cell death. This preservation of structure highlights the early stage of necrosis typical of red infarcts, emphasizing the dual blood supply (pulmonary and bronchial arteries) that contributes to both ischemia and hemorrhage. 2. Liquefactive Necrosis Features: o Complete digestion of dead cells, forming a viscous liquid mass (pus). o Tissue is replaced by cellular debris and neutrophils. Mechanism: o Enzymatic digestion by hydrolytic enzymes, typically from neutrophils. Common Causes: o Bacterial and fungal infections. o Ischemic or hemorrhagic stroke in the brain: § Ischemic Stroke: Caused by a blockage of blood flow to the brain due to a thrombus or embolus, leading to tissue ischemia, liquefactive necrosis, and eventual formation of a fluid-filled cavity. § Hemorrhagic Stroke: Caused by a ruptured blood vessel, leading to bleeding, compression of brain tissue, and increased intracranial pressure. Examples: o Brain infarcts. o Liver abscesses. Brain blood clot This image shows a blood clot in the brain, a significant cause of mortality (e.g., 4th most frequent in Denmark). The clot leads to ischemic damage in the brain, resulting in liquefactive necrosis. This type of necrosis occurs due to the brain’s high lipid content and lack of structural connective tissue, which causes rapid tissue breakdown into a fluid-like, dissolved state. While some speculate this is a faster form of coagulative necrosis, the liquified appearance and cavity formation are distinct features of liquefactive necrosis, commonly observed in ischemic brain injuries. Liver abscess This image shows a liver abscess, an example of localized liquefactive necrosis. Abscesses are newly formed cavities within tissue, filled with pus, which includes necrotic debris and large numbers of neutrophils. These immune cells release enzymes that break down tissue, leading to the liquefaction characteristic of this necrosis type. While liquefactive necrosis is most associated with the brain (e.g., following ischemic injury), abscesses in other organs, like the liver, are also significant examples of this process. 3. Fat Necrosis Features: o Focal areas of fat destruction, often with chalky white deposits. o Saponification occurs when fat necrosis is accompanied by high calcium influx, resulting in the formation of insoluble calcium-fatty acid salts. Mechanism: o Lipases release during tissue injury or enzymatic dysfunction, breaking triglycerides into fatty acids. o Fatty acids interact with calcium, forming insoluble salts (only in saponification). Common Causes: o Trauma to fat tissues, such as in breast injuries. o Pancreatitis, particularly in alcohol-induced cases, where digestive enzymes attack nearby fat. Examples: o Pancreatic necrosis o Fat necrosis in breast tissue Pathogenesis In pancreatitis, stress on the pancreatic acinar cells—due to alcohol, reactive oxygen species (ROS), or duct obstruction—causes the release of digestive enzymes. These enzymes begin digesting pancreatic cells and surrounding tissues, including nearby fat. Lipases split triglycerides into fatty acids, which can further combine with calcium ions in a process called saponification, forming chalky white, soap-like deposits. While fat necrosis refers broadly to the enzymatic destruction of fat tissue, saponification highlights the secondary reaction involving calcium influx and fatty acid binding. Etiology 1. Duct Obstruction Causes: Conditions like cholelithiasis (gallstones), ampullary obstruction, chronic alcoholism, or ductal concretions. Pathophysiology: Obstruction leads to interstitial edema, which compresses blood vessels and impairs blood flow, resulting in ischemia. Ischemia promotes activation of digestive enzymes within the pancreas, contributing to tissue injury. 2. Acinar Cell Injury Causes: Triggered by alcohol, drugs, trauma, ischemia, hypercalcemia, or viral infections. Pathophysiology: Injured acinar cells release digestive enzymes (e.g., lipases and proteases) prematurely, either intracellularly or extracellularly. Enzyme activation leads to autodigestion of the pancreas, including fat necrosis, proteolysis, and damage to vessel walls (hemorrhage). 3. Defective Intracellular Transport Causes: Metabolic injuries (e.g., alcohol) or duct obstruction. Pathophysiology: Pancreatic proenzymes (inactive precursors) are abnormally delivered to the lysosomal compartment. Enzymes become activated intracellularly, resulting in acinar cell death and subsequent inflammation. Outcome Activated Enzymes drive key pathological changes in the pancreas: Inflammation and edema from interstitial damage. Proteolysis and digestion of pancreatic proteins. Fat necrosis, with saponification of fatty acids. Hemorrhage due to vessel wall damage. This histological image illustrates fat necrosis with hallmark features such as ghost-like fat cells— eosinophilic remnants of dying adipocytes. The cells have reacted with calcium, forming basophilic granular deposits of calcium salts, characteristic of saponification. These deposits stain darkly on H&E staining and are clearly distinguishable from unaffected fat cells. In addition, there is evidence of lobular panniculitis, with a mixed inflammatory infiltrate composed of foamy histiocytes, lymphocytes, and neutrophils, further contributing to the breakdown of adipose tissue. This macroscopic image depicts saponification in the omentum 2 , visible as chalky white deposits. These areas represent calcium salts formed by the combination of free fatty acids (released from adipocytes by lipases) and calcium ions, a hallmark of fat necrosis. Such deposits serve as an indicator to surgeons of ongoing fat necrosis and enzymatic digestion, commonly associated with conditions like pancreatitis. 4. Caseous Necrosis Features: o "Cheese-like" appearance of tissue. o Amorphous granular debris surrounded by granulomatous inflammation. Mechanism: o Lipid-rich mycobacterial cell walls resist complete digestion. Common Cause: o Tuberculosis. Examples: o Pulmonary lesions in tuberculosis. Pathogenesis and granuloma When Mycobacterium tuberculosis infects tissue, the immune system attempts to contain the bacteria by forming granulomas, which are organized structures of immune cells. 2 layer of fatty tissue that drapes over the abdominal organs, providing protection, insulation, and immune support Granuloma Structure: o Central area of caseous necrosis, characterized by cheese-like, necrotic debris resulting from immune-mediated tissue destruction. o Macrophages surround the necrotic core, often transforming into epithelioid cells or fusing to form multinucleated giant cells. o Surrounding the macrophages are lymphocytes, predominantly T cells (shown as black dots), which help regulate the immune response. Pathogenesis: o The granuloma serves to “wall off” the bacteria, preventing further spread. However, the bacteria may remain viable within the granuloma, leading to latent TB infection. o Latent TB can reactivate in immunocompromised states (e.g., with diseases like COVID-19, RSV, or HIV), causing widespread tissue damage and active TB disease. The macroscopic image shows the characteristic cheese-like appearance of caseous necrosis, often seen in granulomatous diseases like tuberculosis. This appearance is due to the necrotic tissue’s soft, white, and friable consistency, resembling cottage cheese. The microscopic image highlights the absence of any recognizable tissue architecture, distinguishing caseous necrosis from coagulative necrosis. The pink staining represents protein-rich debris from dying cells, and the necrotic core is surrounded by inflammatory cells, including macrophages and lymphocytes, forming a granuloma. This type of necrosis is caused by bacterial infections, primarily Mycobacterium tuberculosis, rather than ischemia or infarction. If stained for the causative bacteria (e.g., acid-fast stain for TB), the bacteria could be identified within the granuloma. The hallmark of this process is the immune system’s attempt to contain the infection, leading to granuloma formation around the necrotic core. 5. Fibrinoid Necrosis Features: o Bright pink, amorphous material visible in vessel walls on histology, resembling fibrin. o Initially thought to consist primarily of fibrin, but now understood to also contain proteins and collagen, contributing to its “fibrin-like” appearance. Mechanism: o Triggered by immune complex deposition in blood vessels. o This activates the immune response, leading to fibrin leakage and accumulation of plasma proteins within the vessel wall. Common Causes: o Immune-mediated vascular damage, such as: o Vasculitis (e.g., polyarteritis nodosa). o Autoimmune diseases, such as systemic lupus erythematosus (SLE). o Non-immune causes, including severe hypertension or mechanical stress on vessel walls. Examples: o Vasculitis with immune complex deposition in vessel walls. o Systemic lupus erythematosus (SLE) involving vascular injury. o Hypertensive damage in arterioles. o Peptic ulceration, with fibrinoid material on the luminal surface. Hypertensive damage in arterioles This sequence of images illustrates the progression of vascular damage due to very high blood pressure (hypertension) leading to fibrinoid necrosis: 1. Hyaline Arteriolosclerosis: Persistent hypertension forces plasma proteins into the intima and media layers of small arteries, causing their thickening. The vessel wall becomes glassy and homogeneous, appearing pink on H&E staining. 2. Hyperplastic Arteriolosclerosis: Continued pressure causes proliferation of smooth muscle cells and fibroblasts in the media. This results in concentric, “onion-skin” thickening of the arterial wall, progressively narrowing the lumen and reducing blood flow. 3. Fibrinoid Necrosis: If the damage progresses further, the vessel ruptures or becomes severely inflamed due to immune cell activation and fibrin leakage. The wall appears as a bright pink, amorphous deposit of fibrin, plasma proteins, and necrotic debris, representing fibrinoid necrosis. This pathological sequence can lead to complete vessel occlusion, tissue ischemia, or localized hemorrhage. The hallmark of fibrinoid necrosis is the replacement of normal vessel structure with necrotic fibrin-like material, resulting from severe immune-mediated or mechanical vascular injury. 🚩 In hyaline arteriolosclerosis, deposited proteins in the intima and media include C3b, a complement protein. C3b binds to extracellular matrix components like hyaluronic acid, indicating classical complement pathway activation. This immune activation exacerbates inflammation, attracts leukocytes, and promotes vascular remodeling, contributing to the progression of vascular damage. Fibrinoid necrosis – wall of artery shows circumferential bright pink area of necrosis This image illustrates fibrinoid necrosis in an arteriole, where the vessel wall disintegrates and is replaced by bright pink amorphous material on staining. This material includes a mix of fibrin, collagen, and other proteins, indicating severe vascular injury often seen in conditions like vasculitis or hypertension. The intense staining highlights the loss of cellular architecture and immune-mediated damage. This image shows fibrinoid necrosis in a kidney, highlighting massive cell destruction and widespread tissue death. The surface appears irregular and dotted with pale areas, indicative of extensive necrosis and vascular injury. Such damage is commonly associated with severe hypertension, autoimmune disorders, or immune complex deposition, leading to significant functional impairment of the organ. 6. Gangrenous Necrosis Features: o Combination of coagulative and liquefactive necrosis. o Dry gangrene: Tissue appears mummified, dry, and darkened due to ischemia. o Wet gangrene: Infected tissue with swelling, pus, and a foul odor due to bacterial infection. Common Causes: o Ischemia: Leading to dry gangrene (coagulative necrosis). o Superimposed infection: Causes wet gangrene (liquefactive necrosis due to tissue lysis by bacterial and neutrophilic enzymes). Examples: o Diabetic foot ulcers. o Bowel infarction. Foot – gangrene, early clinical presentation This image depicts an early clinical presentation of gangrenous necrosis in a foot, characterized by tissue damage due to a loss of blood supply, resulting in coagulative necrosis. In this stage, the tissue shows no signs of bacterial infection or leukocyte infiltration, making it a case of dry gangrene. The affected area appears darkened and shriveled, reflecting ischemic injury. If left untreated, bacterial superinfection can occur, leading to wet gangrene, which is marked by tissue swelling, pus formation, and systemic complications such as sepsis, potentially resulting in death. 7. Dystrophic calcification Features: o Local deposition of calcium at sites of cell injury and necrosis. o Characterized by dark, violet-stained formations in histological sections, indicating calcium accumulation in dying tissue. o Calcium binding in fat cells is called saponification, while in other tissues it is referred to as dystrophic calcification. Mechanism: o Tissue necrosis or degeneration releases enzymes that: § Breakdown organic phosphates, which bind to calcium. § Alter pH, leading to increased calcium deposition. o The calcium deposits are associated with proteins and other cellular debris in the necrotic tissue. Common Causes: o Cell injury or necrosis from trauma, inflammation, or chronic degenerative conditions. o Commonly seen in fat necrosis (as saponification) and other tissue injuries. Examples: o Fat necrosis (calcium binding to fatty acids in damaged fat cells). o Calcification in necrotic areas of atherosclerotic plaques or damaged cardiac valves. Lymph Node Dark violet staining in the tissue represents calcium deposits associated with necrosis. These deposits highlight the accumulation of calcium in dying cells, often linked to conditions like tuberculosis or chronic infections. Atherosclerosis in Arteries Calcium deposition is visible as black or violet areas on histological slides, often occurring in necrotic cores of atherosclerotic plaques. These are hallmarks of chronic injury and inflammation in blood vessels. Aorta (Macroscopic View) Chalky white deposits are visible on the aorta, indicative of dystrophic calcification. This occurs due to necrotic damage, often seen in atherosclerosis or aging-related vascular changes. Lung On imaging, dystrophic calcifications in the lung appear as dense, localized areas, which can sometimes mimic malignancies. These arise from prior infections or chronic inflammatory processes. Aortic Valve Calcification is evident as white, hard deposits on the valve, leading to potential stenosis or impaired valve function. This is commonly seen in aging or post-inflammatory conditions. Differences Between Dystrophic and Metastatic Calcification Feature Dystrophic Calcification Metastatic Calcification Definition Deposition of calcium salts in dead Deposition of calcium salts in normal, or damaged tissues. healthy tissues. Calcium Normal. Deranged. Metabolism Serum Normal. Elevated (hypercalcemia). Calcium Levels Reversibility Generally irreversible. Reversible upon correction of underlying metabolic disorder. Causes Necrosis (caseous, liquefactive, fat Hyperparathyroidism, chronic kidney necrosis), infarcts, thrombi, disease, vitamin D intoxication, atherosclerosis, tumors, chronic metastatic carcinoma, milk-alkali inflammation. syndrome. Pathogenesis Localized calcium deposition due Systemic hypercalcemia leads to to phosphates binding calcium at calcium-phosphate precipitation in sites of tissue injury or necrosis. normal tissues. Examples Calcifications in atherosclerotic Calcification in lungs, kidneys plaques, heart valves, granulomas, (nephrocalcinosis), stomach, and necrotic tumors, fat necrosis. systemic soft tissues. IV. Comparative Summary of Necrosis Types Type Pathological Appearance Common Causes Examples Coagulative Firm, preserved tissue Ischemia, hypoxia Myocardial, renal infarcts Liquefactive Pus-like, liquid tissue Bacterial/fungal infections, hypoxia Brain infarcts, abscesses Caseous Cheeselike debris Tuberculosis Tuberculous lung lesions Fat Chalky white spots Trauma, pancreatitis Breast tissue injury, pancreas Fibrinoid Pink vessel walls Immune reactions Vasculitis Programmed Cell Death and Apoptosis Objectives: you are expected to be able to explain general molecular disease processes and how they manifest in tissues and organs. you should furthermore be able to identify these manifestations histologically. Learning outcomes: explain the different types of programmed cell death and autophagy, as well as the underlying causes know the connection between cellular adaptation, cell stress and cell death I. Introduction to cell death Cell death is a normal physiological process where approximately 50 to 70 billion cells die daily, equating to the body’s weight annually. It plays a critical role in maintaining homeostasis and is intricately involved in disease. Excessive cell death, such as in ischemia, or its absence, as seen in cancer, highlights its importance in pathological conditions. Evolution of Disease The progression of disease can be understood in four key stages. Etiology refers to the underlying causes of diseases, such as infections, trauma, or genetic abnormalities. Pathogenesis focuses on the mechanisms that lead to disease development, revealing how these causes disrupt normal cellular function. This disruption results in morphologic changes, which include structural and functional abnormalities in cells and tissues. Finally, these changes manifest as clinical symptoms and signs, forming the clinical manifestations of the disease. Causes of Cell and Tissue Injury Cell and tissue injuries arise from various causes, including infections, toxins, genetic mutations, nutritional imbalances, and physical trauma. These factors disrupt cellular homeostasis, leading to biochemical and structural changes that can progress into pathological conditions. For instance, infections caused by bacterial or viral agents, toxins from environmental or pharmaceutical sources, and genetic mutations can initiate a cascade of damaging events at the cellular level. Cellular Response to Injury Cells respond to injury in two ways: reversible or irreversible damage. A normal cell, under homeostatic conditions, functions optimally. In reversible injury, cells may adapt to mild, transient stressors through swelling of organelles like the endoplasmic reticulum and mitochondria, formation of membrane blebs, and accumulation of lipid vacuoles. This stage is recoverable if the stimulus is removed. However, if the injury persists or intensifies, it progresses to irreversible damage. In such cases, cells undergo necrosis, which is uncontrolled cell death, or apoptosis, a programmed and orderly process of cellular self-destruction. Progression of Cellular Injury Progression of cellular injury begins with reversible damage, where the cell attempts to adapt to mild or transient stress. During this phase, the initial cellular response includes swelling of organelles like the endoplasmic reticulum and mitochondria, formation of membrane blebs, and the appearance of lipid vacuoles (fatty changes). These changes impair cellular function temporarily but are not permanent, and recovery is possible if the stressor is removed. However, if the injury persists or worsens, it progresses to progressive injury, where the damage becomes more severe and ultimately irreversible. This stage is marked by the breakdown of the plasma membrane, leading to leakage of cellular contents into the surrounding tissue and triggering an inflammatory response. Mitochondrial damage becomes significant, disrupting energy production and signaling pathways. Once these changes occur, the cell’s structural integrity is irreversibly compromised, resulting in necrosis, a form of uncontrolled cell death. Short Reversible Period After Injury The timeline of injury reveals a brief period during which cell damage is reversible. Initially, cell function declines, followed by ultrastructural and microscopic changes. If the injury persists, gross morphological alterations occur, signaling irreversible damage. This emphasizes the importance of timely intervention to prevent the transition from reversible to irreversible injury. Point of No Return in Cell Death The concept of the “point of no return” in cell death remains undefined. Attempts to identify this threshold include markers such as massive activation of intracellular enzymes (e.g., caspases), irreversible mitochondrial permeability changes, and translocation of phosphatidylserine—a key apoptotic signal. However, a consensus from the National Cell Death Committee (NCCD) confirms that no definitive method or marker exists to determine the exact moment when cell death becomes inevitable. II. Necrosis Necrosis, derived from the Greek word for “death,” is a significant form of cell death associated with disease. Unlike programmed cell death (apoptosis), necrosis is uncontrolled and often results from external factors such as trauma, ischemia (lack of blood supply), or toxin exposure. This type of cell death is inflammatory and not regulated by enzymes like caspases, which are central to apoptosis. Causes of Necrosis Necrosis can result from a variety of conditions, including: Trauma: Physical damage to tissues. Ischemia: Reduced blood flow leading to oxygen deprivation. Toxins: Exposure to harmful substances. Infections: Microbial damage causing tissue breakdown. Key Steps in Necrosis 1. Cellular Swelling and Plasma Membrane Permeability: Cells and their organelles swell as the plasma membrane’s permeability increases. This marks the early stage of cellular failure. 2. Ion Pump Failure and Blebbing: Ion pumps in the plasma membrane cease functioning, leading to blister-like protrusions (blebs) on the cell surface. These blebs contain fluid (mostly water). Eventually, ruptures in the plasma membrane occur, causing the leakage of intracellular components. 3. Karyolysis: The nucleus dissolves, and DNA is broken down in a disordered manner. 4. Cell Rupture and Inflammation: Swollen cells burst, releasing their contents into the surrounding tissue. This triggers an inflammatory response, often resulting in further tissue damage. 5. Tissue Necrosis: Necrosis develops within 24 hours of the initial injury, leading to the breakdown and death of tissue. Microscopic Changes At the cellular level, necrosis is characterized by: Blebbing: The formation of fluid-filled surface protrusions due to plasma membrane instability. Rupture of Plasma Membrane: Disintegration of the membrane leads to the release of cellular components, exacerbating inflammation. Loss of Nuclear Integrity: The nucleus undergoes disintegration (karyolysis), marking the cell’s irreversible death. Comparison to Other Cell Death Types Necrosis is uncontrolled, inflammatory, and often caused by external factors. Apoptosis, on the other hand, is a regulated and non-inflammatory process of programmed cell death, typically necessary for normal development and tissue homeostasis. III. Apoptosis – programmed cell death Apoptosis, or programmed cell death, is a highly regulated process that allows cells to die in an orderly fashion without triggering inflammation. This process is essential for maintaining tissue homeostasis, development, and immune function. Unlike necrosis, apoptosis is non-inflammatory and involves activation of intracellular signaling pathways, including caspases, which coordinate the dismantling of cellular components. When Apoptosis is Prominent Apoptosis occurs during both normal physiological processes and pathological conditions: 1. Normal Body Functions: Embryogenesis: Essential for shaping organs and eliminating unnecessary cells during development. Hormone-Dependent Physiological Changes: Example: Regression of the endometrium during the menstrual cycle. Proliferating Cell Populations: Example: Removal of aged or damaged cells in intestinal crypts. Immune System Regulation: Elimination of self-reactive immune cells to prevent autoimmune reactions. 2. Pathological Conditions: DNA Damage: Cells with irreparable damage undergo apoptosis to prevent propagation of mutations. Example: Radiation exposure. Infections: Infected cells, particularly in viral hepatitis, may undergo apoptosis to limit infection. Protein Misfolding Disorders: Cells with excessive accumulation of misfolded proteins, such as in CNS degenerative diseases, may initiate apoptosis. Apoptosis Apoptosis is a highly regulated and structured form of cell death distinct from necrosis. It plays a crucial role in normal physiological processes and prevents inflammatory responses, which is why it is referred to as a “clean” form of cell death. Morphological Features of Apoptosis Chromatin Condensation: One of the earliest and most distinctive features of apoptosis. DNA within the nucleus condenses into dense patches, reflecting the orderly dismantling of nuclear material. Membrane Blebbing: The plasma membrane forms outward protrusions, called blebs, which eventually bud off as part of the apoptotic process. These blebs contain intact organelles and cellular material, avoiding leakage into the extracellular space. Cellular Fragmentation: The cell breaks into smaller, membrane-bound structures called apoptotic bodies. These bodies are compartmentalized to contain cellular contents, preventing the release of potentially harmful intracellular material. Phagocytosis: Specialized phagocytic cells, such as macrophages, recognize and engulf apoptotic bodies. This efficient clearance prevents the release of intracellular substances into the extracellular space, avoiding inflammatory responses. By orchestrating the condensation of chromatin, membrane blebbing, and the formation of apoptotic bodies, apoptosis ensures minimal disturbance to the surrounding tissue. This contrasts sharply with necrosis, which often triggers inflammation and exacerbates tissue damage. The specialized uptake of apoptotic bodies by phagocytes highlights the efficiency of this process, making apoptosis vital for development, immune regulation, and the maintenance of cellular homeostasis. Histological Staining Techniques Apoptosis can be observed and studied using various histological and molecular techniques that highlight its key features: Hematoxylin and Eosin (H&E) Staining: Hematoxylin: A basic dye that stains nuclei a purplish-blue color, highlighting chromatin condensation. Eosin: An acidic dye that stains the extracellular matrix and cytoplasm pink. Used to visualize the morphological changes in cells undergoing apoptosis, such as chromatin condensation and cytoplasmic changes. Feulgen Staining: Specific for DNA, this staining method identifies nuclear DNA by reacting with aldehyde groups of depurinated DNA. Useful in detecting apoptotic cells with fragmented DNA. TUNEL Assay (Terminal deoxynucleotidyl transferase dUTP Nick End Labeling): Detects DNA fragmentation, a hallmark of apoptosis. Involves labeling the 3’-OH ends of broken DNA strands using the enzyme terminal deoxynucleotidyl transferase (TdT). Apoptotic cells appear as brown or fluorescently labeled structures, depending on the visualization method. The Two Major Apoptotic Pathways Apoptosis is executed through two primary pathways: the intrinsic (mitochondrial) and extrinsic (death receptor) pathways. Both ultimately lead to caspase activation, cellular disassembly, and phagocytic clearance, but they are triggered and regulated differently. Intrinsic Pathway (Mitochondrial-Dependent) Trigger: Activated by internal signals such as: Growth factor withdrawal. DNA damage (radiation, toxins, free radicals). Protein misfolding (ER stress). Mechanism: Regulated by Bcl-2 family proteins (e.g., Bax, Bak), which alter mitochondrial outer membrane permeability. Pore formation in the outer mitochondrial membrane allows release of factors such as cytochrome c into the cytosol. Cytochrome c interacts with apoptotic protease-activating factor-1 (Apaf-1) to form the apoptosome, which activates caspase-9. Outcome: Caspase activation leads to: Nuclear fragmentation: Chromatin condensation and DNA breakdown. Cytoskeletal disintegration: Loss of cellular structure. Formation of apoptotic bodies that are phagocytosed without inflammation. Extrinsic Pathway (Receptor-Ligand Driven) Trigger: Activated by external signals, typically via death receptor-ligand interactions: Fas receptor (FasL ligand). TNF receptor (TNF ligand). Mechanism: Death receptor activation recruits adaptor proteins such as FADD (Fas-associated death domain). These adaptors directly activate caspase-8 (or caspase-10 in some cells). This pathway bypasses mitochondrial involvement. Outcome: Direct caspase activation leads to the same terminal events as the intrinsic pathway: Nuclear fragmentation. Formation of apoptotic bodies. Phagocytic uptake of cell fragments. Both pathways converge on the executioner caspases (e.g., caspase-3 and caspase-7), which dismantle cellular components and facilitate apoptotic body formation. Phagocytic cells then recognize and engulf these bodies, ensuring a non-inflammatory cleanup process. This dual- pathway system provides redundancy and flexibility, allowing cells to initiate apoptosis in response to both internal and external threats. Caspases in Apoptosis Caspases (Cysteine Aspartate-Specific Proteases) are central enzymes in apoptosis, functioning as molecular scissors to dismantle cellular components in a precise, regulated manner. They are categorized based on their roles in the apoptotic process: Types of Caspases Initiator Caspases: Includes Caspases 2, 8, 9, and 10. Activated early in the apoptotic pathways (intrinsic or extrinsic). Function to activate executioner caspases. Executioner (Effector) Caspases: Includes Caspases 33, 6, and 7. Trigger the breakdown of cellular components such as DNA, cytoskeletal proteins, and organelles. Execute the morphological and biochemical hallmarks of apoptosis. Caspase Targets ICAD (Inhibitor of CAD): Cleavage by executioner caspases activates CAD (Caspase- Activated DNase), which fragments nuclear DNA. PARP (Poly ADP Ribose Polymerase): Cleaved to prevent energy consumption during apoptosis, conserving cellular ATP. Cytoskeletal Proteins: Cleavage leads to the dismantling of the cytoskeleton, enabling cell shrinkage and apoptotic body formation. Pathways of Caspase Activation Intrinsic Pathway: Triggered by mitochondrial release of cytochrome c. Cytochrome c forms the apoptosome with Apaf-1, activating caspase-9, which then activates downstream executioner caspases. Extrinsic Pathway: Triggered by death receptor activation (e.g., Fas, TNF). Receptor-adaptor complexes activate caspase-8, directly activating executioner caspases. 3 can be detected in situ or in vivo using specific antibodies, making it an excellent marker for identifying apoptotic cells where caspases are activated. Mitochondrial Outer membrane Permeabilization (MOMP) drives the intrinsic pathway Pore Formation: The mitochondrial outer membrane becomes permeable, releasing key molecules like cytochrome c into the cytosol. Caspase Activation: Cytochrome c interacts with Apaf-1, forming the apoptosome, which activates caspase-9 and subsequently caspase-3. ICAD Cleavage and CAD Activation: o Caspase-3 cleaves ICAD (Inhibitor of CAD), releasing CAD (Caspase-Activated DNase). o Activated CAD fragments DNA, dismantling the nucleus and promoting cell death. While MOMP is critical for activating caspases, the process of apoptosis can still proceed without CAD activation. However, CAD is essential for the efficient removal of potentially harmful genomic material, enhancing the “clean-up” phase of apoptosis. Minority MOMP and Alternative CAD Activation A physiological variation of MOMP, called Minority MOMP, involves subtle changes in mitochondrial permeability that result in: Moderate Release of Cytochrome c: o Triggers localized and controlled CAD activation. o Causes specific DNA incisions without triggering full apoptosis. Role in Differentiation: o This controlled DNA cleavage drives processes like muscle cell differentiation. o Terminally differentiated cells, such as muscle cells, rely on minority MOMP to fine-tune differentiation processes. Implications and Challenges Dual Role of CAD: o While CAD’s activation is critical for normal differentiation, the DNA regions cleaved by CAD are also associated with translocation events implicated in cancer. o For example, CAD’s activity could contribute to genomic instability in cancer cells, raising concerns about its role as a “mutator” that aids tumor progression. Potential Roles in Cancer: o Investigations suggest pathways involving ICAD and CAD may have additional, non-apoptotic roles in cancer biology. o CAD’s activity may inadvertently support cancer cells by mutating DNA or promoting survival in specific contexts. The Intrinsic Pathway of Apoptosis: Regulation and Challenges The intrinsic pathway of apoptosis is triggered by internal stress signals, such as DNA damage or the absence of survival factors, leading to mitochondrial outer membrane permeabilization (MOMP). This process is tightly regulated to maintain cellular balance. Key players in this pathway include the pro-apoptotic proteins BAX and BAK, which form pores in the mitochondrial membrane, allowing the release of cytochrome c. This release activates caspases, ultimately driving apoptosis. The anti- apoptotic BCL-2 family proteins antagonize BAX and BAK to prevent premature cell death, requiring careful modulation during stress conditions. Unlike the receptor-driven (extrinsic) pathway, the intrinsic pathway is harder to regulate due to its reliance on finely tuned mitochondrial signals, including minority MOMP events that contribute to differentiation or repair rather than full apoptosis. Dysregulation of this pathway can result in excessive cell survival, as seen in cancer, or inappropriate cell death, highlighting the importance of its precise control. BCL-2 Family Proteins and Regulation of Apoptosis The BCL-2 family of proteins plays a crucial role in regulating apoptosis through interactions between pro-apoptotic and anti-apoptotic members. Pro-apoptotic proteins, such as BAX, BAK, and BOK, belong to the BH1-3 subgroup and contain characteristic BH domains that enable their pore-forming activity at the mitochondrial membrane. These proteins facilitate cytochrome c release, initiating caspase activation and apoptosis. In contrast, anti-apoptotic proteins like BCL-2, BCL-XL, and MCL-1 (BH1-4 subgroup) counteract the activity of BAX and BAK by binding to their BH3 domains, preventing pore formation and cytochrome c leakage. This dynamic regulation ensures apoptosis occurs only under appropriate conditions, maintaining cellular homeostasis. Dysregulation of these interactions, such as overexpression of anti-apoptotic BCL-2 proteins, can lead to impaired apoptosis and contribute to diseases like cancer. Understanding this balance is critical for developing targeted therapies that manipulate these pathways. so the antiapoptotic proteins would be BH 124 so this would for example be this BCL 2 that can then interact and and make sure that the channels are closed and so then when you activate the optic pathway you bring in these subset of factors here these proapoptotic proteins that are called BH-3 only so they would require that there are quite a few of these big bit vimpat Puma noxa and they can be activated in different ways and reacting to different stimuli so they are antagonizing directly CL2 but they can also help actually in activating bug spark and it's still not for all of them exactly clear what is the major contribution if it's activation of bugs bug or if it's antagonizing BCL 2 so so yeah so that's kind of basic science questions that that we're still working on I have to say that also downstream of backs and back I mean that's a that's a huge machinery that operates maybe you've heard about it already with utilization factors and for leasing signalling so so it is relatively complicated it's just in in the in the context and the setup of this course it's not really relevant hopefully you will hear about it in other courses but as mentioned understanding cell death pathways is relevant disease and also in treatment of disease and so one of the quite major breakthroughs in for example Cancer Research and cancer treatment has been the ability to reactivate so because you can imagine that having excessive levels of detail too is is is needed for cancer cells so that's a common way to escape tosis is to load up BCL 2 to close your your backpack channels efficiently but set of molecules one that is particularly well known and which is in use in the clinic is venetoclax which binds to the B3B3 binding groove of BCL 2 and and inhibits its function and you can imagine that once PCL 2 is inhibited this makes the box back channels are more susceptible to activation and this is indeed what happens so that's a quite efficient way of eradicating cancer cells but you can imagine that normal cells could there be an issue indeed there is right so PCL 2 of course and normal cells is also suppressive so this means that common side effects related to unwanted cell killing so it was thought of as a kind of wonder drug but then in reality it turned out As for all anti cancer drugs to be something that has to be used with the care yeah so just to summarize necrosis versus artosis at this stage right so apoptosis generally affects the single cell no inflammatory response typically characterized by cell shrinkage as you also saw this gigantic multinucleated cells that that this membrane bleeding leading to to bots with with integrity of membranes confined and of course this chromatin condensation and DNA fragmentation and phagocytic market facility responds the other hand the crisis affects groups of neighbouring cells and can such affect the whole tissue very significantly and not least because of the significant significant inflammatory response that also could affect the whole body in fever and and strong immune reactions in necrosis cells generally as well there's a loss of membrane integrity and and this random degradation of DNA and live cells can of course be ingested by macrophages but it's really an inefficient right because there is there isn't this confined control of of intracellular components as in the pot forming in apple sources OK so when when is which type of death evoked you could say so there's certain types here of injuries that would typically lead trend towards necrosis but not completely black and white and as I mentioned there is this necroptosis so it's not but they're just kind of a general rule of the thumb so market changes in metabolism such as during ischemia where region it's completely short of from from from oxygen would lead to massive cell injury and necrosis on the other hand radiation and some more kind of particular DNA damage or damage to mitochondria accumulation of these misfolded proteins in the cytoplasm but also certain types of inflammation would be triggering although only cruises of course also could be playing a role in general with inflammation as I will show later there are many different cell types that are related to apoptosis that are that are relevant you may have heard heard of them already actually OK so just looking a little bit at some classic terms which you may already have heard so necrosis talked about this tissue breakdown Tissue breakdown here's a terrible example of this gangrene there is a carrier lysis and carries career highs and and prognosis depicted here this is catalysis so this nuclear fading so that's what you would see for example in the chaotic cell death there was also a pig nosis this nuclear shrinkage you can sometimes in apoptosis see I think you've seen it actually already that the dying cells would have at least at at at some time point clearly markedly staining DNA areas I would say it's a relatively large pieces of the UK that I can look agnostic and then finally there is the carrier rexes which is nuclear fragmentation these are kind of classical terms to describe the nuclear and because and you know in these pathology sections these are so relatively easy to see as manifestation even without any specific markers right Yep like why would happen and like we've seen our license and I guess pretty similar but yeah and that that's pignoise that's more just the shrinkage that's something like dehydration yeah for example let's carry this is more fragmentation it's more disintegration that can happen after certain types of damage and and and rupturing so that's why yeah I think I recall some of the the the samples that you saw in the in in in in the intestine might have looked a little bit agnostic as if it was shrinking yeah so that's that's before cat has efficiently degraded the the commencing Yep so as I mentioned in earlier times it was relatively easy there was apoptosis and necrosis and then more and more different types of cells pathways have been has have been uncovered we will briefly talk about autophagy dependent program cell death but