CRP 201: Water Relations in Plants Lecture Notes PDF
Document Details
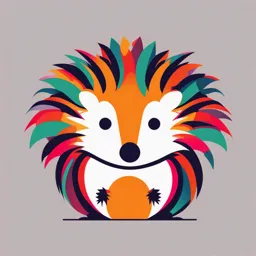
Uploaded by UndisputableBowenite303
Olabisi Onabanjo University
Tags
Summary
This document provides an introduction to water relations in plants. It covers fundamental concepts, including water absorption, transport, and properties of water. It discusses various forces that influence water potential and explains the importance of water in agricultural productivity.
Full Transcript
## CRP 201: INTRODUCTION TO AGRICULTURAL BOTANY II LECTURE NOTE 7 ### WATER RELATIONS IN PLANTS #### INTRODUCTION The study of water relations in plants encompasses the area of water absorption by the roots, the transport of water within the plant body, and subsequent release of water to the atmosph...
## CRP 201: INTRODUCTION TO AGRICULTURAL BOTANY II LECTURE NOTE 7 ### WATER RELATIONS IN PLANTS #### INTRODUCTION The study of water relations in plants encompasses the area of water absorption by the roots, the transport of water within the plant body, and subsequent release of water to the atmosphere. For every gram of organic matter made by a plant, approximately 500g of water is absorbed by the roots, transpired through the plant body, and lost to the atmosphere. Water typically constitutes 80-95% of the mass of a growing plant tissue, and may be lower about 75% in wood. Seeds with water content of 5-15% are among the driest of plant tissues, yet before germinating they must absorb a considerable amount of water. About 100 times the fresh weight of the plant may be lost through the leaf surfaces, and this can result into cooling of leaf surfaces. Such water loss is called transpiration. Of all the resources that plants need to grow and function, water is the most abundant and at the same time the most limiting for agricultural productivity. #### Properties of water Water is polar in nature and thus gives rise to hydrogen bonds, and also makes it an excellent solvent. The thermal properties of water result from hydrogen bonding. It has high specific heat and latent heat of vaporization. For water at 25°C, the heat of vaporization is 44kJ/mol, the highest value known for any liquid. #### Cohesive and adhesive properties of water Cohesion is the mutual attraction between water molecules. Adhesion is the attraction of water to a solid phase such as a cell wall or glass surface, while surface tension is the energy required to increase the surface area of an air-water interface. All these properties give rise to a phenomenon known as capillarity, the movement of water along a capillary tube. The upward movement of water in a capillary tube is due to: - the attraction of water to the polar surface of the glass tube (adhesion) - the surface tension of water. The study of water relations in plants is best described in terms of various forces acting at various parts of the plant, which determines the movement of water within plants and between plants and its environment. These forces are related to water potential, which is a quantitative expression of the free energy associated with water. The water content in the soil, plants, and atmosphere is usually described as water potential (Ψw). This is based on the relation between the water content in the part of a system and pure water at the same temperature and atmospheric pressure, measured in pressure units (megapascal-MPa or bars-Bar). By definition, the potential of free pure water at atmospheric pressure and at a temperature of 25°C corresponds to 0 (zero) MPa. The contrast in the water potential between two points invariably determines the direction of water transport in a system. More precisely, the water potential (Ψ) represents all the water pressure in a given system and it is the sum of osmotic or solute potential (Ψπ), matrix potential (Ψm), hydrostatic pressure or the turgor potential (Ψp) and the gravitational potential (Ψg) i.e Ψ = Ψπ+ Ψm + Ψp+ Ψg Osmotic potential (Ψπ) is the chemical potential of water in a solution due to the presence of dissolved substances (solutes). It is always negative because the water moves from one point with a lower concentration of solutes (for example, pure water) to a point with a higher concentration. So, the higher concentration of the solutes at a point which makes the system more negative will be the osmotic potential in this place. Matric potential (Ψm) is another force that can influence water potential especially when water moves through a charged surface – mainly by soil components and cell walls. In the soil, this influence of the matrix is so great that water potential is assumed negligible and therefore equivalent to the matrix potential. In plants however, the volume of matric water is very small compared to the volume of vacuolar water in parenchyma, therefore Ψm has a negligible effect on the total water potential. However, in developing seeds or thick walled cells where the vacuolar water constitutes a small fraction of the total water, matric potential can control the cell water potential. Hydrostatic pressure or the turgor potential (Ψp) is the physical pressure that water exerts on a given system. If we observe a turgid cell of a root cortex or a leaf mesophyll, the hydrostatic pressure is positive. However, in a xylem vessel subjected to a stressful condition – in a transpiring plant – this component of hydrostatic pressure is negative. Gravitational potential (Ψg). The effect of gravitational water is of negligible importance within root or leaf but becomes important in comparing potentials in leaves at different heights on trees and in soils. Upward movement of water in a tree trunk must overcome a gravitational force of 0.01MPa/m and gravity causes drainage of water downward in soil. Thus, for herbaceous plants and annual field crops of a short vertical height (<10m) the values of the matric potential and gravitational potential are small and are commonly omitted. Thus, Ψ - Ψπ + Ψp | Location | Water potential | Pressure (Ψp) | Osmotic (Ψπ) | Gravity (Ψg) | Water potential in gas phase (RT/Vw In {R}) | |-------------------------------------|-----------------|---------------|----------------|---------------|--------------------------------------| | Outside air (relative humidity-50%) | -95.2 | | | | -95.2 | | Leaf internal air space | -0.8 | | | | -0.8 | | Cell wall of mesophyll (at 10m) | -0.8 | -0.7 | -0.2 | 0.1 | | | Vacuole of mesophyll (at 10m) | -0.8 | 0.2 | -1.1 | 0.1 | | | Leaf xylem (at 10m) | -0.8 | -0.8 | -0.1 | 0.1 | | | Root xylem (near surface) | -0.6 | -0.5 | -0.1 | 0.0 | | | Root cell vacuole (near surface) | -0.6 | 0.5 | -1.1 | 0.0 | | | Soil adjacent to root | -0.5 | -0.4 | -0.1 | 0.0 | | | Soil 10mm from root | -0.3 | -0.2 | -0.1 | 0.0 | | The movement of water from the soil through the plant to the atmosphere involves different mechanisms of transport. - in the soil and the xylem, water moves by bulk flow in response to a pressure gradient - in the vapour phase, water moves primarily by diffusion at least until it reaches the outside air where convection (a form of bulk flow) becomes dominant. - when water is transported across membranes, the driving force is the water potential difference across the membrane. Such osmotic flow occurs when cells absorb water and when roots transport water from the soil to the xylem. #### Water content in soil With regard to the physiological aspect, it is important to point out that the water content in soil is associated with three terms - field capacity, the permanent wilting point, and the available water content. The term "field capacity" corresponds to the maximum water content that a given soil can retain by capillarity, after saturation and gravity drainage, and it is conventionally estimated as the water content when the matrix potential is -0.03 MPa (-0.3 Bar). In general, clay soils or those with higher content of organic matter (upper to 5% of organic matter) present a higher soil water holding capacity (average field capacity ranging from 35 to 40%vol). In contrast, sandy soils have a lower water holding capacity and field capacity typically ranges from 10-15%vol. The wilting point also known as permanent wilting point, can be defined as the amount of water per unit weight (or volume) of soil that is so tightly retained by the soil matrix that roots are unable to absorb causing the wilting of plants. In other words, it corresponds to the water potential of soil under which plants cannot maintain turgor pressure, even if a series of defence mechanisms have been triggered. At the permanent wilting point, the water potential of soil tends to be less than or equal to the osmotic potential of the plant, which is extremely low in plants adapted to dry environments. The available water is the difference between field capacity (FC) and permanent wilting point (PWC). AW-FC-PWC Thus, available water should be considered as a relative value, for the same soil water potential, it may have different proportions of accessibility, depending on the ability of each species to exploit or capture available water. #### Soil water movement Water moves through soils predominantly by bulk flow driven by a pressure gradient. In addition, diffusion of water vapor accounts for some water movement. As plants absorb water from the soil, they deplete the soil of water near the surface of the roots. This depletion reduces Ψw in the water near the root surface and establishes a pressure gradient with respect to neighboring regions of soil that have higher Ψw values. Because the water-filled pore spaces in the soil are interconnected, water moves to the root surface by bulk flow through these channels down the pressure gradient. * The rate of water flow in soils depends on two factors - the size of the pressure gradient through the soil, and the hydraulic conductivity of the soil. Soil hydraulic conductivity is a measure of the ease with which water moves through the soil, and it varies with the type of soil and water content. Sandy soils, with their large spaces between particles, have a large hydraulic conductivity, whereas clay soils, with the minute spaces between their particles, have an appreciably smaller hydraulic conductivity. #### Uptake of water by plant roots With the water reaching the roots of a plant, the absorption process is directly dependent on the water potential gradient between the rhizosphere (the plane of soil surrounding the root where maximum interaction between the roots of plant and the soil is observed) and the root xylem. There are two ways to establish this gradient, characterized by two absorption processes. 1) osmotically driven absorption, common in plants with low transpiration activity; and 2) passive absorption, which dominates in plants with high transpiration activity. The osmotically driven absorption occurs in plants under conditions of heat and non-limiting water availability in the soil, but with a restricted capacity of transpiration (for example, without leaves or with a limited vapour pressure deficit). In these cases, there is an accumulation of solutes in xylem vessels (for example, sucrose by degradation of starch reserves in the roots), reducing the xylem water potential in relation to the soil water potential (Kramer & Boyer, 1995). In passive absorption and with an increasing rate of transpiration, the tension in the xylem vessels increases, indicating a predominance of the pressure potential influence upon the osmotic potential through the establishment of the water potential gradient between the root xylem and the rhizosphere. Under these conditions, the roots become a passive absorption organ, where the water is sucked into a mass flow promoted by the transpiration activity of aerial parts of the plant. The movement of water inside the plant is driven by a reduction in free energy, and water may move by diffusion, by bulk flow or by a combination of these fundamental transport mechanisms. Water diffuses because molecules are in a constant thermal agitation which tends to even out concentration differences. Water moves by bulkflow in response to a pressure gradient, whenever there is a suitable pathway for bulk movement of water. Thus, water potential difference (i.e solute potential and pressure potential) across the cells starting from root hairs to xylem plays an important role in uptake and transport of water. #### Pathways for water movements in plant The water intake in the roots can follow three ways into the root tissue in relation to the route of the epidermis to the endoderm of the root, called radial water transport. - apoplastic pathway, where the water moves through the intercellular spaces and does not pass through any membranes, exclusively occupying the continuous network of the cell walls; - symplastic pathway, where the water moves exclusively from one cell to another through plasmodesmata connections; and - transmembrane pathway, which corresponds to a mixed path between the first two, where the water goes in one direction through the root tissue, entering (symplastic) and exiting (apoplastic) cells. The relative importance of these pathways is still a cause of much discussion, but there is some evidence for the suggestion that plants displaying low transpiration activity predominantly witness symplastic transport, while those displaying high transpiration activity witness a greater proportion of apoplastic transport (Boyer, 1985; Steudle, 2001). Experiments with the pressure probe technique also indicated that the apoplast pathway is particularly important for water uptake by young corn roots (Frensch et al., 1996, Steudle and Frensch, 1996). #### THE XYLEM TISSUE The xylem is the tissue most involved in water translocation, and consists of several different types of cells, living and non-living. Of all these cell types, the tracheary elements are the most characteristics, and it is through these cells that practically all water translocation takes place. Also found in the xylem tissue are xylem fibers and living parenchyma cells. The tracheary elements consist of the vessel elements and tracheids and are the cells most concerned with water translocation in the plant. Both are more or less elongated, have lignified secondary walls and are dead when mature and functional. Since both are dead at maturity there is no interfering protoplast in the lumina of the cells - a situation that allows for the efficient translocation of relatively large amounts of water. Perforated end walls are characteristics of vessel elements, but do not occur in tracheids. However, tracheids are well supplied with bordered pits, structures which allow water to pass from one tracheid to another. The vessel elements are the principal pathway by which water is translocated in the angiosperms. However, vessels are not present in the conifers and in this group, the tracheids form the principal pathway for water translocation. Obviously, the movement of water in a group of tracheids as compared to vessel systems is much less direct and meets with more resistance. It is interesting thing to note however is that most of our taller trees are conifers which are completely devoid of vessels. #### Mechanisms involved in water movement in plants Three mechanisms have been proposed to explain the movement of water in plants. These include root pressure, vital theories, and cohesion-tension theory. #### Root pressure Stocking (1956) defines root pressure as a pressure developing in the tracheary elements of the xylem as a result of the metabolic activities of the roots. Root pressure is therefore referred to as an active process. It should be noted and clearly understood that the movement of water up the stem as a result of root pressure is due to osmotic mechanisms (passive) which are created as a result of the active absorption of salt by the roots. We can refer to root pressure as being an active process in the sense that living roots are essential for it to occur. However, several reasons have been provided to prove that root pressure is not responsible for movement of water in plants. - the magnitude of pressure developed by root is too small (< 2atm) to push water up to the heights reached by most trees. Indeed root pressures of any magnitude are conspicuously absent from most conifers which are among the tallest of trees. In addition, most estimates of the ability of root pressures to raise water to appreciable heights do not take into account the friction encountered in the passage of water through the xylem ducts. - exudation rates are generally much slower than normal transpiration rates. - xylem sap under normal condition is generally under tension instead of pressure, an observation which supports the argument that the root pressure is not an important factor in water translocation. However, when conditions for transportation are poor, root pressure may be a significant factor in the movement of water e.g guttation. Root pressure is most likely to occur when soil water potentials are high and transportation rates are low. When transpiration rates are high, water is taken up so rapidly into the leaves and lost to the atmosphere that a positive pressure never develops in the xylem. Plants that develop root pressure frequently produce liquid droplets on the edges of their leaves, a phenomenon known as guttation. Guttation is most noticeable when transpiration is suppressed and the relative humidity is high such as during the night. #### Vital theories Many early investigations believed that the ascent of water in plants was under the control of vital activities in the stem. This belief was most likely stimulated by the fact that living cells are present in the xylem tissue. This has also been doubted. Strasburger (1891, 1893) demonstrated that stems in which living cells have been killed by the uptake of poisonous are still capable of water translocation. It appears quite probable that living cells of the stem have little to do with the movement of water. However, this has not been proven in the water relations of plants. #### Cohesion-Tension theory The general mechanism to explain this upward movement of water is the cohesion-tension theory, which was proposed in the late 19th century. Basically, this theory holds that the water evaporated in leaves establishes a tensile strength in the xylem, where the hydrogen bonds provide a continuous intermolecular attraction (cohesion) between the water molecules from the leaf to the root. Recently, the cohesion theory has been questioned as a result of assessments of tension in xylem vessels, which do not present a direct relation with tension values measured on leaves through pressure chambers probably due to cavitation (a phenomenon of bubble formation in the water column under tension) introduced when the xylem walls are punctured with the glass capillary of the pressure probe (Tyree, 1997). Furthermore, it is assumed that the hydrophobic interaction between the internal walls of the xylem and the sap composition (lipids, proteins, polysaccharides etc.) prevents the development of a tensile strength larger than 1 MPa (Zimmerman et al., 2004), which is smaller than the estimated tension of rising water in a 30 m high tree (3 MPa). However, despite these questions, many studies argue that the fundamentals of the cohesion-tension theory are still valid for explaining the water flow in the continuous soil-plant-atmosphere (Richter, 2001; Steudle, 2001; Cochard, 2002; Tyree, 2003). #### TRANSPIRATION The loss of water from aerial parts of plants in the form of vapor is known as transpiration. Water loss from plants is through openings on the surface of leaves known as stomata (singular -stoma). In addition to stomatal transpiration, water is lost as vapor directly from leaf surfaces and through lenticels (small openings in the corky tissue covering stems and twigs). The former is called cuticular transpiration and the later as lenticular transpiration. Stomatal transpiration accounts for 80-98% of the total transpiration loss from plants (free to herbaceous plants) under very dry conditions, stomata are closed and water loss occurs through the cuticle and lenticels. Cuticular transpiration accounts to 2to 20% of the total transpiration loss from plants which is insignificant when compared to stomatal transpiration. In the continuum soil-plant-atmosphere of water flow, there are two major factors determining the water potential of a plant. (a) the water potential of the soil, which characterises the water supply; and (b) transpiration, which defines the loss of water. The plant, which is an intermediate in this process, may regulate the water potential gradient between the soil and the atmosphere primarily through the regulation of stomatal conductance. The stomata have a quick and fine control of the water relations of a plant, coordinating the control of the water potential gradient between the leaf and the air. Transpiration from the leaf depends on two major factors. (1) the difference in water vapor concentration between the leaf air spaces and the external air and (2) the diffusional resistance () of this pathway. The difference in water vapor concentration is expressed as Cwv (leaf) - Cuv(air). The water vapor concentration of bulk air (Cwv(air)) can be readily measured, but that of the leaf (Cwv(leaf)) is more difficult to assess. Whereas the volume of air space inside the leaf is small, the wet surface from which water evaporates is comparatively large. (Air space volume is about 5% of the total leaf volume for pine needles, 10% for corn leaves, 30% for barley, and 40% for tobacco leaves.) In contrast to the volume of the air space, the internal surface area from which water evaporates may be from 7 to 30 times the external leaf area. This high ratio of surface area to volume makes for rapid vapor equilibration inside the leaf. Thus, we can assume that the air space in the leaf is close to water potential equilibrium with the cell wall surfaces from which liquid water is evaporating. The concentration of water vapor, Cwv changes at various points along the transpiration pathway. We see from Table 7.2 that Cwv decreases at each step of the pathway from the cell wall surface to the bulk air outside the leaf. The important points to remember are (1) that the driving force for water loss from the leaf is the absolute concentration difference (difference in Cwv, in mol m³), and (2) that this difference depends on leaf temperature. | Relative humidity | Concentration (mol m³) | Water vapour Potential (MPa)* | |-----------------|-----------------------|-----------------------------| | 0.99 | 1.27 | -1.38 | | 0.95 | 1.21 | -7.04 | | 0.47 | 0.60 | -103.7 | | 0.50 | 0.50 | -93.6 | Source: Adapted from Nobel, 1999 Throughout this water route between the leaf and the air there are two components that can exert resistance to the diffusion process. 1) stomatal resistance (rs), the resistance associated with diffusion through the stomatal pore which is coordinated by the stomatal opening; and 2) air boundary layer resistance (rb), which is located closest to the leaf surface and it is directly influenced by wind speed. The higher the speed of the wind, the greater is the frequency of air renewal in this layer surrounding the leaf, restricting diffusion resistance for the maintenance of a major gradient of the water potential. #### Transpiration ratio The effectiveness of plants in moderating water loss while allowing sufficient CO2 uptake for photosynthesis can be assessed by a parameter called the transpiration ratio. Transpiration ratio (T,)is defined as the amount of water transpired by the plant, divided by the amount of carbon dioxide assimilated by photosynthesis. In C3 plants about 500 molecules of water are lost for every molecule of CO2 fixed by photosynthesis, giving a transpiration ratio of 500. The reciprocal of transpiration ratio gives the water use efficiency (WUE) of plants; in C4 and CAM plants T, are 250 and 50 respectively i.e WUE- 1/T, for the C3 plants described above WUE - 1/500 - 0.002 The large ratio of H2O efflux to CO2 influx results from three factors. 1. The concentration gradient driving water loss is about 50 times larger than that driving the influx of CO2. In large part, this difference is due to the low concentration of CO₂ in air (about 0.03%) and the relatively high concentration of water vapor within the leaf. 2. CO2 diffuses about 1.6 times more slowly through air than water does (the CO₂ molecule is larger than H₂O and has a smaller diffusion coefficient). 3. CO₂ uptake must cross the plasma membrane, the cytoplasm, and the chloroplast envelope before it is assimilated in the chloroplast. These membranes add to the resistance of the CO2 diffusion pathway. #### Significance of transpiration Transpiration is advantageous because - It creates suction force and help in the ascent of sap - It helps in the absorption of water and minerals by roots. - It helps in evaporating excess amount of water from moist soil. - It plays a role in translocation of food from one part of the part of the plant to the other..