Optical Communication Technologies PDF
Document Details
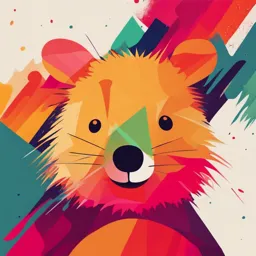
Uploaded by QualifiedBauhaus
Université Abdelmalek Essaâdi
Pr. Yassin LAAZIZ
Tags
Summary
This document is a collection of lecture notes/presentation slides on high-speed and all-optical telecommunications. It covers a broad range of topics, from basic concepts like broadband and optical fiber technology, to more advanced topics like optical amplifiers and switching technologies focusing on different generations. The document provides a wide overview of technical developments from the 1970s to the 2020s in this field.
Full Transcript
Génie des Systèmes de Télécommunications et Réseaux Haut Débit & Télécommunications Tout-Optique --o-- Broadband & All- Optical Telecommunications Pr. Yassin LAAZIZ Université Abdelmalek Essaadi ENSA de Tanger 1...
Génie des Systèmes de Télécommunications et Réseaux Haut Débit & Télécommunications Tout-Optique --o-- Broadband & All- Optical Telecommunications Pr. Yassin LAAZIZ Université Abdelmalek Essaadi ENSA de Tanger 1 7-déc.-24 BROADBAND AND THE NEED FOR SPEED 2 7-déc.-24 Nielsen's Law of Internet Bandwidth The high-end user's connection speed grows by 50% per year. 3 7-déc.-24 Bandwidth Growth 4 7-déc.-24 Bandwidth Growth Internet of Things (IoT) - 2013 A network of uniquely identifiable things that communicate without human interaction using IP connectivity 5 7-déc.-24 Bandwidth Growth Internet of Everything (IoE) - 2020 Bringing together the people, things, data and process to make networked connections more meaningful by turning information into actions. - Smart home security systems. - Autonomous farming equipment. 6 - Wearable health monitors. 7-déc.-24 - Smart industry (4.0)…. OPTICAL FIBER & INTERNET All the services we have today on the Internet require high-speed connections that use cable links based on optical fibers. Optical transmission technology has undergone numerous advances since its introduction in the 1970s to meet the ever-growing demand for increased bandwidth. 7 7-déc.-24 Internet Traffic Around 99% of intercontinental Internet traffic is carried by fiber optic submarine cables. 8 7-déc.-24 Optical Systems Evolution Several key elements have collectively contributed to the evolution and advancement of optical transmission technology, enabling the creation of communication networks with high capacity and high performance. 9 7-déc.-24 Optical Systems Evolution 1st generation (1975-1978) - Multi-mode optical fibers - LED et LD MLM at 0.9µm (1st Window) - Intermodal dispersion - Electronic Amplification - Figure of Merit : some Gb/s.km - One Optical Systems Evolution 2nd generation (1978-1989) - Single mode Fibres (SMF) - FP Laser Diodes (MLM) at 1.3µm - Chromatic dispersion issues - Electronic amplification - One - Dispersion Shifted Fibers (SMF-DS) - Monomode LD at 1.55*µm - Minimal linear attenuation (~0.2dB/km) - 2 0.85 µm 1.3 µm or 1.3 µm & 1.5 µm - Figure of merit : 100 Gb/s. km Optical Systems Evolution 3rd generation (1989-2000) - Erbium Doped Fiber Amplification : EDFA - Multiplexing systems : WDM, DWDM - Dispersion Compensating Fibres : DCF - Non-zero Dispersion Shifted Fibers : NZ-DSF puis NZ-DSF (LEAF) Forward Error Correction : FEC (Reed-Solomon codes) OOK with Modulation formats Figure of Merit : 100 000 Gb/s.km. Optical Systems Evolution 4th generation (2005 - 2010) - Routing & Switching optically : OXC, OADM - Compensation of dispersion Slope - Raman Amplification : No need of In-line amplifiers - 40Gbit/s/channel limitation, due to non linear effects - Objective : 10 Tbit/s/fiber - 5th optical window : Fibres LWP Fibers (G652C & G652D) - Coerse WDM (CWDM) - FTTH … Optical Systems Evolution 5th generation (2010 - 2020) Coherent transmission and Digital Signal Processing (DSP) - Coherent optical transmission is a technique that uses modulation of the amplitude and phase of the light, as well as transmission across two polarizations, to enable the transport of considerably more information through a fiber optic cable. Using digital signal processing at both the transmitter and receiver. - Data rates reached 100 Gbps per wavelength. On/Off Modulation Coherent transmission Tx Rx Coherent Transmission Complexity A symbol is the combination of amplitude/phase states and polarization states, which can be referred to as a “dual-pol symbol. Digital Signal Processing (DSP): Sophisticated DSP algorithms compensate for impairments such as chromatic dispersion, polarization mode dispersion, and nonlinear effects, ensuring high-fidelity signal recovery over long distances. 15 7-déc.-24 Optical Systems Evolution 6th generation (2020 - ….) Space Division Multiplexing (SDM) - Multicore and few-mode fibers, and massive WDM. - Combined WDM with SDM to increase fiber capacity dramatically - Advanced FEC methods like LDPC codes - Aggregate data rates exceeded 1 Tbps per fiber. - Towards Petabit transmission : Researchers have successfully transmitted high data rates over multicore fibers: A notable experiment demonstrated 305 Tbps using a 19-core multicore fiber and advanced WDM techniques. Multicore fibers with fewer cores (e.g., 7 or 12 cores) have also been tested in lab environments, achieving terabit-level capacities using a combination of SDM, WDM, and coherent transmission 16 7-déc.-24 WDM a Key Element for Optical Communications Increasing Bandwidth by multiplication of channels. 17 7-déc.-24 OPTICAL NETWORKS TOPOLOGY 18 7-déc.-24 SDH / SONET SONET (Synchronous Optical NETwork) USA SDH (Synchronous Digital Hirarchy) EUROPE SDH/SONET are two international standards for broadband transmission over optical networks. Based on TDM (Time Division Multiplexing), Used to transport different streams of data at different rates : IP / MPLS, ATM, DSL, Ethernet, PDH,.. 19 7-déc.-24 SDH / SONET example 20 7-déc.-24 WDM Advantages Easier network expansion Just add a new wavelength by connecting a new transmitter and receiver at the ends of the link Transparency to protocols (IP, ATM, SDH). 21 7-déc.-24 WDM Advantages WDM yield greater fibre capacity By increasing the number of channels By increasing throughput by channel : STM-64 (~10 Gbps), can move to STM-256 (~40 Gbps) WDM is a cost effective way of increasing capacity without replacing fibre. No need to replace in-line components such as optical amplifiers Incremental cost for a new channel is low 22 7-déc.-24 DWDM Standards ITU - G.692 Channels lie in the C- Band from 1528.77 nm to 1560.61 nm and in the L-Band from 1570 nm to 1620 nm. Supervisory channel also specified at 1510 nm to handle alarms and monitoring 23 7-déc.-24 DWDM Standards ITU - G.692 ITU Recommendation is G.692 "Optical interfaces for multichannel systems with optical amplifiers" G.692 includes a number of DWDM channel plans ITU channel spacings are 0.4 nm, 0.8 nm and 1.6 nm (50, 100 and 200 GHz) 0.8 nm 1550 1551 1552 1553 1553 1554 Systems with smaller channel spacing, 0.2 nm (25 GHz) and even 0.1 nm (12.5 GHz), exist. But, this requires laser sources with excellent long term wavelength stability, better than 10 pm (cooled lasers too expensive). 24 7-déc.-24 ITU DWDM Channel Plan 0.8 nm Spacing (100 GHz) All Wavelengths in nm 1528.77 1534.64 1540.56 1546.52 1552.52 1558.98 1529.55 1535.43 1541.35 1547.32 1553.33 1559.79 1530.33 1536.22 1542.14 1548.11 1554.13 1560.61 ITU C-Band 1531.12 1537.00 1542.94 1548.91 1554.94 43 channels defined 1531.90 1537.79 1543.73 1549.72 1555.75 1532.68 1538.58 1544.53 1550.52 1556.55 1533.47 1539.37 1545.32 1551.32 1557.36 1534.25 1540.16 1546.12 1552.12 1558.17 Speed of Light assumed to be 2.99792458 x 108 m/s 25 7-déc.-24 ITU DWDM Channel Plan 0.4 nm Spacing (50 GHz) All Wavelengths in nm 1528.77 1534.64 1540.56 1546.52 1552.52 1558.58 1529.16 1535.04 1540.95 1546.92 1552.93 1558.98 1529.55 1535.43 1541.35 1547.32 1553.33 1559.39 1529.94 1535.82 1541.75 1547.72 1553.73 1559.79 1530.33 1536.22 1542.14 1548.11 1554.13 1560.20 ITU C-Band 1530.72 1536.61 1542.54 1548.51 1554.54 1560.61 1531.12 1537.00 1542.94 1548.91 1554.94 1531.51 1537.40 1543.33 1549.32 1555.34 81 channels defined 1531.90 1537.79 1543.73 1549.72 1555.75 1532.29 1538.19 1544.13 1550.12 1556.15 1532.68 1538.58 1544.53 1550.52 1556.55 Another channel plan 1533.07 1538.98 1544.92 1550.92 1556.96 exists for the L-band 1533.47 1539.37 1545.32 1551.32 1557.36 above 1565 nm 1533.86 1539.77 1545.72 1551.72 1557.77 1534.25 1540.16 1546.12 1552.12 1558.17 Speed of Light assumed to be 2.99792458 x 108 m/s 26 7-déc.-24 CWDM (G694.2) - An economical WDM solution. - 20 nm channel spacing. - 8 to16 channels. - Erbium doped fiber amplifiers (EDFAs) not necessary. - For metropolitan networks (MAN). 27 7-déc.-24 DWDM/CWDM U-DWDM: Ultra Dense Wavelength Division Multiplexing More closer inter-channel spacings: up to 10 GHz (0,08 nm). 28 7-déc.-24 DWDM Basic System Receivers DWDM Multiplexer Optical fibre Power Line Line Receive Amp Amp Amp Preamp DWDM Transmitters DeMultiplexer 200 – 700 km Each wavelength behaves as if it has it own "virtual fibre“. Optical amplifiers help to overcome losses in mux/demux and long fibre spans. 29 7-déc.-24 DWDM System Spans Power/Booster Amp P Amplifiers Optical P R Receive Preamp R 160-200 km L Line Amp P L L R up to 600-700 km L 3R L P Regen R 700 + km 30 7-déc.-24 3R regeneration 31 7-déc.-24 3R regeneration in DWDM System In long-haul networks using EDFAs, transmitted signals must be regenerated every 700 km or so (depending on the characteristics of the EDFA) to overcome the signal loss due to attenuation, distortion caused by dispersion and nonlinear effects, as well as noise build-up generated within the EDFAs themselves. This regeneration is accomplished through optical-to-electrical-to- optical (O-E-O) conversion. The signal may then be reamplified, reshaped and retimed (3R). Such regeneration equipment is required on a per-channel basis, which is costly. Repeaters are costly and difficult to maintain in underground and submarine fiber optic links. That is why operators seek to use a lowest number of regenerators online. 32 7-déc.-24 Dual Fiber DWDM DWDM DWDM SFP - Multiplexer DeMultiplexer Transceiver Receivers Transceiver Power Line Receive Amp Amp Preamp DWDM DWDM DeMultiplexer Multiplexer Transmitters SFP = Small Form-Factor Pluggable Receive Line Power Preamp Amp Amp A link is ensured in general by two fibers; one fiber for each direction. At each end of the link there is transmitters and receivers. A combination of transmitter and receiver (for a given wavelength) is called "transceiver". Transceivers are easily plugged on the reception module. 33 7-déc.-24 Transceivers SFP (Small Form-Factor Pluggable) The small form-factor pluggable (SFP) is a compact, hot-pluggable network interface module for fiber-optic links. An SFP can easily be replaced for upgrading links without changing the entire hardware bloc of the module. Internal view of an SFP A range of SFPs is available for multimode or A 2,5Gb/s DWDM SFP single mode cabling and for different span 34 distances. 7-déc.-24 DWDM Transponders Optical Transponder Unit (OTU) : Converts incoming optical signals into the precise ITU-standard wavelengths that can then be multiplexed. Some transponders can perform electronic 3R functions. Ensures Dual fiber to single fiber and Multimode to single mode conversions. An 8 ports transponder = 4 data paths The wavelengths from all of the transponders in the system are then optically multiplexed. In the receive direction of the DWDM system, the reverse process takes place. + 3R 35 7-déc.-24 Bi-directional DWDM When a high number of channels is not required Bidirectional WDM can lead to a reduction in the number of fibers and line components (EDFA, DCF,..) Different wavelength bands are used for transmission in each direction. Typically the bands are called: The "Red Band", upper half of the C-band to 1560 nm The "Blue Band", lower half of the C-band from 1528 nm 1R 1B Transmitter Transmitter Transmitter 2R 2B Transmitter Red Band DWDM DWDM nR Mux/Demux Mux/Demux nB Transmitter Transmitter Receiver Blue Band Receiver 1B 1R Receiver 2B Fibre 2R Receiver Receiver Receiver nB nR 36 7-déc.-24 The need for a Guard Band To avoid interference red and blue bands must be separated This separation is called a "guard band" Guard band is typically about 5 nm waste of spectral space G u a Blue r d Red channel channel B band a band n d 1528 nm 1560 nm 37 7-déc.-24 Passive DWDM Passive DWDM systems use bi-directional transmission and do not have active components. The line only works through the optical budget of the transceivers used. No optical signal amplifier or dispersion compensator is used. Main application : metro networks and high speed communication lines with large channel capacity. Limited number of channels; limitations to upgrade systems 38 7-déc.-24 Bi-directional CWDM For CWDM systems adjacent wavelengths are sufficiently separated and the interference problems do not arise. The guard band is not necessary. 39 7-déc.-24 DWDM components Implementation of WDM networks requires a variety of passive and/or active devices to combine, distribute, isolate and amplify optical power at different wavelengths 40 7-déc.-24 DWDM Passive components - Fibers - Dispersion compensator (DCF) - Isolators - Circulators - Couplers / Spliters - Fiber Bragg Grattings (FBG) - Filters - Multiplexers-Demultiplexers - Array Waveguide Gratting (AWG) - Optical Add Drop Multiplexers (OADM) 41 7-déc.-24 Fibers Conventional Single-Mode Fiber (SMF) 30 20 S CL ITU-T : G652 Dispersion (ps/nm) 10 Commercialized since 1983. 0 The most deployed by operators. -10 First single-channel systems -20 operated at 1310nm. -30 Zero dispersion point at 1310nm. 1250 1350 1450 1550 1650 Wavelength (nm) No system amplification at 1300nm ; D(1530-1565nm) = 16 - 19 ps/nm*km WDM systems moved to 1550nm: wide low-loss window, but higher D = 0.065 ps/nm2km dispersion (So used with DCF). Aeff = 85 um2 42 7-déc.-24 Fibers Dispersion-Shifted Fiber (DSF) 30 20 S C L ITU-T : G653 Dispersion(ps/nm) 10 Available since 1985. 0 Move the zero dispersion point to -10 1550nm, so no dispersion compensation required for -20 1550nm signals, even at 10G. -30 However, lack of dispersion at 1250 1350 1450 1550 1650 Wavelength(nm) 1550 aggravates FWM and severely limits optical power levels for C-band DWDM systems. Thus, DSF was a “bad idea” for DWDM. 43 7-déc.-24 Fibers ITU-T G653 limitations With DWDM the aggregate optical power on a single fiber is high : Simultaneous transmission of multiple optical channels Optical amplification Causing Non-linear effects; whose effects are more critical with Higher optical power levels, Decreasing channel spacing. 44 7-déc.-24 Fibers Fiber non-linearities As long as optical power within an optical fiber is small, the fiber can be treated as a linear medium : Loss and refractive index are independent of the signal power. linear degradation of signal : Attenuation, dispersion When optical power levels get fairly high, the fiber becomes a nonlinear medium : Loss and refractive index depend on the optical power. non-linear degradation of signal : Four wave mixing (FWM) Self Phase modulation (SPM) Cross Phase Modulation (CPM) Stimulated Raman Scattering (SRS) Stimulated Brillouin Scattering (SBS) 45 7-déc.-24 Fibers Fiber non-linearities Refractive index variation (Kerr effects) The refractive index of glass is dependent on the optical power going through the material. The general equation for the refractive index of the core in an optical fiber is: P (t ) n n0 n2 Aeff Where: n0 = The refractive index of the fiber core at low optical power levels. n2 = The nonlinear refractive index coefficient (2.35 x 10-20 m2/W for silica). P = The optical power in Watts. Aeff = The effective area of the fiber core in square meters. 46 7-déc.-24 Fibers Fiber non-linearities Scattering effects scat - scat + La raie stokes est prédominante à la température ambiante 0,08nm 100nm Rayleigh scattering : an elastic interaction process; i.e. there is no frequency change of the output light. Brillouin Scattering & Raman Scattering : an inelastic interaction processes; resulting in frequency change. The occurrence of these effects is favored by the high power density in the fiber. 47 7-déc.-24 Fibers Limitations of Fiber non-linearities on DWDM systems Crosstalk between Restricts max power / DWDM channels when channel for systems > transmission near zero 10Gb/s dispersion wavelength Very low frequency Appears in WDM shift : to take into systems account in coherent with channel spacing transmission < 25 GHz (0,2 nm) increase gaps of received optical power between channels : Power Tilt & Produce 48 crostalk 7-déc.-24 Fibers Four Wave Mixing Four wave mixing (FWM) is one of the most troubling issues. Three signals combine to form a fourth spurious or mixing component, hence the name four wave mixing, shown below in terms of frequency : Non-Linear Optical Medium Spurious components cause two problems: Interference between wanted signals, causing "crosstalk" Power is lost from wanted signals into unwanted spurious signals 49 7-déc.-24 Fibers FWM: How many Spurious Components? The total number of mixing components, M increases dramatically with the number of channels; It is calculated from the formula: M = 1/2 ( N3 - N ) N is the number of DWDM channels Thus three channels creates 12 additional signals and so on. As N increases, M increases rapidly..... 50 7-déc.-24 Fibers FWM Components as Wavelengths 1 2 3 Original DWDM channels, evenly spaced 1 2 3 Original plus FWM components 123 312 321 Because of even 213 132 231 spacing some FWM components overlap DWDM channels 113 112 223 221 332 331 51 7-déc.-24 Fibers Four Wave Mixing example with 3 equally spaced channels 3 ITU channels 0.8 nm spacing Channel nm FWM mixing components 1542.14 Channel nm 1542.94 Equal spacing 1541.34 1543.74 1541.34 1544.54 For the three channels , and calculate all 1544.54 the possible combinations produced by adding two 1542.94 channel's together and subtracting one channel . 1542.94 1541.34 For example + - is written as and is 1540.54 calculated as 1542.14 + 1542.94 - 1543.74 = 1541.34 1543.74 nm 1542.14 Note the interference to wanted channels caused by 1545.34 the FWM components 312,132, 221 and223 1544.54 52 7-déc.-24 Fibers Four Wave Mixing example with 3 unequally spaced channels 3 DWDM channels Channel nm FWM mixing components 1542.14 1542.94 unequal Channel nm spacing 1541.24 1543.84 1541.24 As before for the three channels , and 1544.64 calculate all the possible combinations 1544.64 produced by adding two channel's together 1543.04 and subtracting one channel . 1543.04 1541.34 Note that because of the unequal spacing there 1540.44 is now no interference to wanted channels 1543.74 caused by the generated FWM components. 1542.04 1545.54 Hence, reducing FWM can be achieved by 1544.74 employing uneven channel spacing 53 7-déc.-24 Fibers FWM problem with 3 DWDM channels 54 7-déc.-24 Fibers Solution for the 3 channels FWM problem 55 7-déc.-24 Fibers FWM : unequally spaced channels results a- System input power spectrum and eye diagram b- System output, equally spaced channels c- System output, unequally spaced channels 56 7-déc.-24 Fibers Reducing Four Wave Mixing Effect of high dispersion Another approach is to use fibre with non-zero dispersion : FWM is most efficient at the zero- dispersion wavelength Problem : direct conflict with need to minimize dispersion ! 57 7-déc.-24 Fibers Dispersion-Shifted Fiber attractive for L-band S-Band C-Band L-Band 20 Dispersion (ps/nm) 16 12 8 4 0 -4 1510 1530 1550 1570 1590 1610 Wavelength (nm) L-band systems attractive for DSF because of reasonable dispersion values 58 7-déc.-24 Fibers Reducing FWM using NZ-DSF ITU-T G.655 Traditional non-multiplexed systems have used dispersion shifted fibre at 1550nm to reduce chromatic dispersion. Unfortunately operating at the dispersion minimum increases the level of FWM. Conventional fibre (ITU-G652) suffers less from FWM but chromatic dispersion rises. Solution is to use "Non-Zero Dispersion Shifted Fibre" (NZ-DSF), a compromise between DSF and conventional fibre. ITU-T standard is G.655 for non-zero dispersion shifted singlemode fibres Small amount of chromatic dispersion at C-band: minimization of nonlinear effects Optimized for DWDM transmission. 59 7-déc.-24 Fibers NZ-DSF Dispersion Characteristics Provides small amount of dispersion over EDFA band Non-Zero dispersion band is 1530-1565 (ITU C-Band) Minimum dispersion is 1.3 ps/nm-km, maximum is 5.8 ps/nm-km in the C band. 60 7-déc.-24 Fibers Lucent TrueWave “Classic” S-Band C-Band L-Band 20 Some dispersion is good Dispersion (ps/nm) 16 for DWDM systems 12 because the optical power 8 is reduced, thus reducing FWM. 4 0 -4 1510 1530 1550 1570 1590 1610 Wavelength (nm) SMF-28 DSF D(1550nm) = ~ 2 ps/nm*km TWC 61 7-déc.-24 Fibers Lucent TrueWave - RS S-Band C-Band L-Band 20 Increase dispersion from 2ps to 4ps/nm.km at Dispersion (ps/nm) 16 1550nm. 12 Significantly reduced 8 dispersion slope, so good for 4 dispersion management. 0 But small effective area ! -4 1510 1530 1550 1570 1590 1610 Wavelength (nm) D(1530-1565nm) = 2.6 – 6.0 ps/nm*km SMF-28 D(1565 – 1600nm) = 4.0 - 8.6 ps/nm*km DSF TWC S = 0.045 ps/nm2km TWRS Aeff = 55 um2 62 7-déc.-24 Fibers Dispersion management : True Wave-RS Accumulated dispersion over a 2000 km fiber span with a dispersion compensator, for two categories of NZ-DSF fibers. (TW-RS) 63 7-déc.-24 Fibers Corning LEAF S-Band C-Band L-Band 20 4 ps/nm*km average dispersion Dispersion (ps/nm) 16 Larger dispersion slope, but 12 Increased effective core area 8 4 0 -4 1510 1530 1550 1570 1590 1610 Wavelength (nm) D(1530-1565nm) = 2.5 - 6 ps/nm*km SMF-28 DSF S ~ 0.083 ps/nm2km TWC TWRS Aeff = 75 um2 64 LEAF 7-déc.-24 Fibers Reducing FWM using LEAF One way to improve on NZ-DSF is to increase the effective area of the fibre. In a singlemode fibre the optical power density peaks at the centre of the fibre core. FWM and other effect take place at locations of high-power density. Large Effective Area Fibres spread the power density more uniformly across the fibre core. Result is a reduction in peak power and thus FWM. 65 7-déc.-24 Fibers Corning LEAF Corning LEAF has an effective area 32% larger than conventional NZ-DSF Result is lower FWM FWM channels levels at least 30 dB below those of wanted channel. Impact on system design is that it allows higher fibre input powers so span increases Section of DWDM spectrum DWDM NZ-DSF shows channel higher FWM components FWM LEAF has lower component FWM and higher per channel power 66 7-déc.-24 Dispersion Compensation Module allocations and dispersion maps Post- + comp. Fiber#1 Fiber#2 [ps/nm] R.D. 0 Distance D [km] D - C C Pre-comp. Fiber#1 Fiber#2 + [ps/nm] R.D. 0 Distance D D [km] - C C Post- & Pre- Fiber#1 Fiber#2 + [ps/nm] comp. R.D. 0 Distance D D D [km] 67 - 7-déc.-24 C C C Dispersion Compensation Mono-Channel Compensation In WDM (Wavelength Division Multiplexing) systems, different channels experience different values of dispersion. If we use a dispersion compensator module with a dispersion parameter (-Dc), only the channel for which the dispersion parameter equals Dc will have its temporal dispersion fully compensated, while there will be uncompensated (residual) dispersion for the other channels. This implies limitations for DWDM systems due to residual dispersion after dispersion 68 compensation modules (DCMs). 7-déc.-24 Dispersion Compensation Multi-Channel Compensation Using Chirped Fiber Bragg Gratting (CFBGs) Multi-channel chromatic dispersion compensation using cascaded CFBGs Illustration of chromatique dispersion compensation using a Pulse CFBG 7-déc.-24 69 Dispersion Compensation Multi-Channel Compensation Using Cascaded Chirped Fiber Bragg Gratting (CFBGs) This chart depicts the reflectivity (top) and group delay (bottom) spectra of the first three channels of the 51-channel FBG-based dispersion compensator. 70 7-déc.-24 Optical Isolators Only allows transmission in one direction through it. Main application: To protect lasers and optical amplifiers from returning reflected light, which can cause instabilities. Insertion loss: – Low loss (0.2 to 2 dB) in forward direction – High loss in reverse direction: 20 to 40 dB single stage, 40 to 80 dB dual stage) 71 7-déc.-24 Optical Circulators Based on optical crystal technology similar to isolators – Insertion loss 0.3 to 1.5 dB, isolation 20 to 40 dB Typical configuration: 3 port device – Port 1 -> Port 2 – Port 2 -> Port 3 – Port 3 -> Port 1 72 7-déc.-24 Fiber Bragg Grating (FBG) as a basic element for network components FBG is a periodic refractive index variation (Period ) written along the fibre (single-mode) core using high power UV radiation. All the wavelengths satisfying the condition 0 = 2 neff are reflected. Current applications: - FBG for Multiplexing / Demultiplexing - FBG for OADM - FBG as EDFA Pump laser stabilizer - FBG as Optical amplifier gain flattening filter - FBG as Laser diode wavelength lock filter - FBG as Tunable filter - FBG as Sensor - …. 73 7-déc.-24 Characteristics of FBG It is a reflective type filter The demanded wavelength is reflected instead of transmitted It is very stable after annealing The gratings are permanent on the fiber after proper annealing process The reflective spectrum is very stable over the time It is transparent to through wavelength signals The gratings are in fiber and do not degrade the through traffic wavelengths. It is an in-fiber component and easily integrates to other optical devices 74 7-déc.-24 FBG as a Multiplexer / Demultiplexer Circulator Circulator Circulator 1, 2 … n FBG at 1 FBG at 2 FBG at 3... 1 2 3 Multiplexer Circulator Circulator Circulator 1, 2 … n FBG at 1 FBG at 2 FBG at 3... 1 2 3 75 De-multiplexer 7-déc.-24 Passive Optical Couplers The evanescent tail from one fiber core couples into another closely spaced fiber core Couplers are obtained by fusing together and stretching two parallel uncoated fibers, in these conditions, part of the mode power at input 1, leaves the fiber 1 and become guided also by the fiber 2. They are used to split light from one fiber to two fibers or to combine light from two fibers to one. These devices provide low insertion loss. 76 7-déc.-24 Fiber optic Coupler (Combiner /Splitters) Fiber optic Couplers take an optical signal and supply two outputs. Y-couplers have equal power distribution, meaning that the two output signal each receive half of the transmitted power. T-couplers have an uneven power Y-coupler distribution. The signal outputs still carrier the same signal, however the power of one output is greater than the second output. 30% 70% 20% 80% 10% 90% T- coupler 77 Fiber optic Coupler 7-déc.-24 T- coupler T-coupler (or Tap Coupler) can be cascaded to connect multiple terminals on a network. Low tap ratios such as 0.1%, 0.01% or 0.001% enable the monitoring without damage or saturation of photodetector. Their main application in WDM systems is to monitor optical power in lasers, EDFA and Raman Amplifiers. 78 7-déc.-24 The 2 2 Fiber Coupler P0 is the input power, P1 is the throughput power, and P2 is the power coupled into the second fiber. P3 and P4 are extremely low signal levels (-50 to -70 dB below the input level) resulting from backward reflections and scattering in the device 3-dB coupler: P1 = P2 = 0.5 P0 79 7-déc.-24 Example : Coupler Performance 80 7-déc.-24 N N Star Coupler Can construct star couplers by cascading 3-dB couplers The number of 3-dB couplers needed to construct an N N star is 16X16 Singlemode star coupler 81 7-déc.-24 Exercice : 82 7-déc.-24 Optical splitter key element for Passive Optical Networks (PON) Passive Optical Splitter is a key device in passive optical network (PON) systems, which splits the optical signal power evenly into all the output ports. A 1x16 optical splitter star-coupler 83 7-déc.-24 Thin-film Filters Obtained by alternating dielectric thin-film layers with different refractive index. Multiple reflections cause constructive & destructive interference. Variety of filter shapes and bandwidths varying from 0.1 to 10 nm. Insertion loss 0.2 - 2 dB, stopband rejection 30 - 50 dB. 0 dB Incoming Spectrum Transmitted Spectrum Reflected 30 dB Spectrum Layers Substrate 1535 nm 1555 nm 84 7-déc.-24 Multiplexer/ Demultiplexer using Thin- film Filters Demultiplexer 85 7-déc.-24 Array Waveguide Grating For Multiplexing / Demultiplexing Constant path difference = L between waveguides All of the wavelengths 1.... 5 1.... 5 Coupler travel along all of the Waveguides waveguides. But because of the Input fibre constant path difference between the waveguides a given wavelength emerges in phase only at one output fibre. At all other output fibres destructive interference cancels 5 out that wavelength. 1 Output fibres 86 7-déc.-24 Array Waveguide Operation An Array Waveguide Demux consists of three parts : 1st star coupler, Arrayed waveguide grating with the constant path length difference 2nd star coupler. The input light radiates in the 1st star coupler and then propagates through the arrayed waveguides which act as the discrete phase shifter. In the 2nd star coupler, light beams from different waveguides interfere, and according to the wavelength this interference is constructive only at one focal position. 87 7-déc.-24 Typical AWG Loss typically 4 - 6 dB Up to 80 channels Low crosstalk High uniformity 88 7-déc.-24 Optical Add-Drop Multiplexer (OADM) An Optical Add-Drop Multiplexer allow access to individual DWDM signals without conversion back to an electronic domain It has the capability of adding one or more new wavelength channels to an existing muli- wavelength WDM signal, or removing (dropping) one or more channels, routing those signals to another network path. In the example below visible colors are used to represent DWDM wavelengths Wavelengths 1,3 and 4 enter the OADM Wavelengths 1 and 4 pass through Wavelength 3 (blue) is dropped to a customer Wavelengths 2 (green) and a new signal on 3 (blue) are added Downstream signal has wavelengths 1,2,3 and 4 Wavelengths 1 2 3 4 Wavelengths 1 2 3 4 OADM 1234 1234 89 Dropped Wavelength(s) Added Wavelength(s) 7-déc.-24 OADM Requirements Remotely configurable: Modern OADMs can be remotely configured. This allows network operators to manage and adjust the optical channels without needing physical access to the equipment. Pass-through wavelengths are not demultiplexed: In an OADM, pass- through wavelengths continue on their path without being demultiplexed. This helps in maintaining the integrity and quality of the signal. Provide a low loss and low noise path for pass-through wavelengths: OADMs are designed to ensure that pass-through wavelengths experience minimal signal loss and noise, which is crucial for maintaining high-quality optical communication. 90 7-déc.-24 Simple Optical Network Example Wavelengths 1 2 3 4 N N o o d d e OADM OADM e A B 1234 1234 N o 1234 N d o e OADM d D OADM e C Note wavelength reuse of "blue" wavelength (no. 3), links Node A and B as well as Node C and A. 91 7-déc.-24 Active components - Tunable FBG - Programmable OADM - Optical modulation - Erbium Doped Fiber Amplifier - Raman Amplification - Optical Switching - Optical Cross-Connect (OXC) 92 7-déc.-24 Tunable FBG for dispersion compensation FBG technology is also well-suited for tunable dispersion compensation. Submitting the FBG to a temperature gradient allows the grating chirp to be changed and, accordingly, the dispersion level to be tuned. Single gratings can be used for producing negative dispersion over a typical range from –800 to –2000 ps/nm. https://www.photonics.com/Article.aspx?AID=19690 The group delay spectra of three channels of a dispersion- 93 7-déc.-24 compensation FBG resulted from adjusting the dispersion by a thermal gradient. Components for programmable OADM Optical Circulator Tunable Fibre Gratings 1....n 1 2 1....n 3 i & j less i & j Gratings etched in fibre Light in port Light out port Can pass or reflect selected wavelengths 1 2 Wavelength selection is tunable 2 3 (thermal or piezoelectric strain) 3 1 Diagram shows a series of gratings reflecting two wavelengths i & j 94 7-déc.-24 Tunable FBG for wavelength filtering Tunning of wavelength can be achieved either by pressure or electrically Example of compression tuning technology. Large wavelength tuning range of 45 nm. http://fltphotonics.com/products.htm 95 7-déc.-24 Programmable OADM Input Circulator (IC) Output Circulator (OC) 1 2 1 2 DWDM in DWDM out 3 Input Fibre Output Fibre 3 Gratings (IFG) Gratings (OFG) Demux Mux Drop Wavelengths Add Wavelengths All incoming wavelengths pass through the IC from port 1 to 2. At the IFG pass-through wavelengths continue toward the OC. The tuning of the IFG selects drop wavelengths that are reflected back to the IC to port 2 and are passed to port 3 to be demultiplexed. Add wavelengths are sent to port 3 of the OC and are passed to port 1 of the OC backward to the OFG. The OFG reflects the add wavelengths forward to port 1 of the OC along with the pass-through wavelengths. At port 1 of the OC the selected add wavelengths and pass- through wavelengths are passed to the output at port 2. 7-déc.-24 96 Laser Modulation Lasers are modulated in a very simple way by varying the injection current. 97 7-déc.-24 Direct modulation limits However, there is a major inconvenience for amplitude modulation of current, indeed if the bit rate exceeds some Gbits/s, the modulation bandwidth of the laser might not be sufficient to handle the rapid changes in current, leading to signal distortion at the laser output. This effect can generally be tolerated for bit rates up to around 10 Gbps. Beyond this threshold, external modulation becomes essential. 98 7-déc.-24 External modulation Here the injection current of the diode will remain constant to yield a continuous laser light; and to create a signal, we use an external device to modulate the light coming from the laser source. In practice, the throughput limits of external modulation systems can vary based on several factors, but typically, these systems can achieve data rates up to 100 Gbps or more. The actual limit depends on the specific technology used, the quality of the optical components, and the system design. Transmission performance by channel, can be improved by using Dual- Polarization modulation techniques and coherent detection. The Mach-Zehnder Modulator. A Mach-Zehnder interferometer is constructed in an integrated optical circuit, where a material with electro- optical properties (change in refractive index resulting from the application of a continuous electric field), e.g. LiNbO3, occupies a length on one of the two arms n1 n1 n2 n1 Δn = n2-n1 = λkE² Signal modulé en amplitude 100 – 400Gbit/s Coherent transmission (NGDWDM) DP-QPSK (Dual-polarization quadrature phase shift keying) modulation format, coherent detection and digital signal processing in the receiver, offer extremely robust technological platform for developing the new generation DWDM (NGDWDM) system with 100 Gbps channel speed. Coherent systems transponders increase spectral efficiency of transmission in four times (two polarizations and phases of signal are used). DP-QPSK more dispersion-tolerant than classical on-off keying (OOK). Structure of optical signal in DP-QPSK modulation format Transmission of 400G Dual-Carrier DP-16QAM is possible since 2013. 101 7-déc.-24 Optical amplification : the EDFA revolution 40 à 80 km 3R (Reamplify, Reshape, Retime) O-E-O 120 km 102 7-déc.-24 EDFA : 1R (Reamplify); amplifies all s Optically EDFA (Erbium Doped Fiber Amplifier) Introduced in the late 1980s, it was the first successful optical amplifier. It was a major factor in the rapid development of fiber-optic networks in the 1990s, because it extended the distance between costly regenerators. In addition, an EDFA amplifies all the channels in a WDM signal simultaneously, whereas regenerators require optical to electrical conversion for each channel. Principle : a high power laser « pump », gives up a part of its energy to amplify the energy of the signal. 7-déc.-24 103 Principle of functioning of an EDFA Functioning like a laser without 4I mirrors, the EDFA uses a 1µs 11/2 semiconductor pump laser to introduce a powerful beam at a shorter wavelength into a section of erbium-doped fiber several meters 4I 13/2 long. = 980nm 10ms pompe The pump light excites the erbium atoms to higher orbits, and the input signal stimulates them to release émission< 1570nm excess energy as photons in phase pompe= 1480nm and at the same wavelength. EDFAs 4I boost wavelengths in the C band, 15/2 and the pump light is typically 1480 Er3+ nm or/and 980 nm. 104 7-déc.-24 Typical gain of an EDFA in the C band - Bandwidth : 40 – 50 nm - Gain: 25 – 50 dB - Noise amplification also 105 7-déc.-24 Interior of an EDFA 106 7-déc.-24 Gain equalization Gain Flattening Filter (GFF) Gain flattening filters (GFFs) flatten the gain profile in optical amplifiers by selectively removing excess power. GFF for EDFAs are based on thin-film filter technology. They provide in line compensation of the spectral gain profile of EDFAs. 107 7-déc.-24 Dual-band (C & L) EDFA EDFA has been developed to extend the operation spectral window of DWDM to the L-band. For this purpose, very long (up to 200 m and more) highly doped erbium fibers are used. The overall covered band extend now from 1530 to 1610 nm. 108 7-déc.-24 RAMAN Amplification EDFAs give localised amplification. The associated high power levels produce non linear effects. With Raman amplification, the amplification is distributed along the transmission fiber avoiding power pics. 109 7-déc.-24 RAMAN Amplification Physics Une Partie de la Lumière est Diffusée dans Toutes les Directions de l’Espace: c’est la diffusion Raman Changement de Photon Vibration des molécules Photon Moins Couleur Pompe Perte d’énergie optique Energétique (=100nm) 110 7-déc.-24 Cette Nouvelle Couleur est à la Longueur d’Onde dite « Stokes » RAMAN Amplification Physics 4.Physique de l’Effet Raman - Effet Raman Stimulé (1962 - R.W. HELLWARTH) Si le Signal est à la Longueur d’Onde Stokes : 1 Photon Pompe 2 Photons Signal (1450nm) (1550 nm) + 1 Photon Signal + 1 Phonon (1550mn) Duplication de Photons 111 7-déc.-24 RAMAN multichannel Amplification 5.Bande Passante de l’Amplificateur - Bande Passante d’un Amplificateur Raman Monopompe - Bande Passante d’un Amplificateur Raman Multipompe 112 7-déc.-24 Raman amplification characteristics The Raman amplification consists in injecting into the line fiber (in contra or copropagative manner) an optical pump, offset by 100 nm with respect to the signal to be amplified. A power transfer by stimulated Raman scattering takes place over about 20 km. - No special fiber need : the amplification medium is the transmission fiber itself. - Less noise than EDFA. - Higher gain passband than other amplification techniques (see figure below) - Requires the use of high-power pumps than in an erbium-doped fiber. Erbium Yterbium Thulium Praseodymium 113 7-déc.-24 EDFA + RAMAN When used alone or as a complement to EDFA, the distributed Raman amplification prevents the signal from undergoing too much attenuation before being reamplified and thus contributes to improving the signal-to- noise ratio of the link. This allows EDFA amplifiers to be spaced even further. 114 7-déc.-24 FEC Forward error correction (FEC) or channel coding is a technique used for controlling errors in data transmission over unreliable or noisy communication channels. FEC consists in transmitting redundancy information along with the data sent by means of a predetermined algorithm. This allows the decoder to detect and correct transmission errors. FEC allows to perform transmission with low error rates. For example, a signal with a BER of 10-4, can be transformed into another with a BER of 10-15 using an FEC decoder. 115 7-déc.-24 RAMAN + FEC The combination of Raman amplification and FEC allows the deployment of very long-haule systems. Instead of the classical unregenerated links of 500 to 800 km distances, links of between 1,500 to 3,000 km can be achieved. Such distances are rarely imposed by geography. So, this combination of technologies has a great economic advantage due to removing / spacing of 3R regenerators. 116 7-déc.-24 Optical Switching Optical switching also called wavelength switching or lambda switching is the technology used in optical networking to switch individual wavelengths of light onto separate paths for specific routing of information. Lambda switching enables a light path to behave like a virtual circuit. Transparent switch: The incoming wavelengths are switched to the output fibers optically, without having to convert them to the electrical domain. Opaque switch: The input optical signals are converted to electrical signals, from where the packets are extracted. Packets are switched using a packet switch, and then they are transmitted out of the switch in the optical domain. 117 7-déc.-24 MEMS Switch Architectures 2D-switching architecture : use ON – OFF mirrors Low loss Low cross-talk Low switching time Low power consumption ON-OFF Switch (optical gate) 2x2 Switch 1xN Switch NxN Switch 118 7-déc.-24 Optical Switching: MEMS Micro-Electro-Mechanical Systems IEEE Communications Magazine, Vol. 40 No. 3 March 2002 119 7-déc.-24 Optical Switching: MEMS 3D-switching architecture : uses multidirectional movable mirrors Mirrors of a 1024 (32x32) port Lamdarouter from Lucent IEEE Communications Magazine, Vol. 40 No. 3 March 2002 AT&T 120 7-déc.-24 Optical Switching: MEMS 3D-switching architecture The direction in which the light beam is reflected can be changed by rotating the mirror to different angles, allowing the input light to be connected to any output port. This device can switch large numbers of optical signals simultaneously. 121 7-déc.-24 Optical Cross-Connect (OXC) Wavelength Routers An optical cross-connect (OXC) is a device used by telecommunications carriers to switch high-speed optical signals. OXC are remotely configurable. Inside the Cross Connect : All Optical Switch Technologies (MEMS). Non-blocking : any combination of dropped/added possible In addition, insertion loss, physical size and switching times are critical considerations. Large number of DWDM wavelengths possible means a large number of ports. OXC Input fibers Output fibers with WDM with WDM channels channels 122 7-déc.-24 OXC Constituents OXC are composed of multiplexers/demultiplexers and Add-drop multiplexers, of wavelength switches and wavelength converters. Multiplexors / Demultiplexors are used for grouping/separating signals of different wavelengths for transmission/commutation purposes. Wavelenght switch inter-connects each entry to the desired exit way. Wavelength convertor Converts an incoming wavelength in a different one when this wavelength is being used in the following fiber. 123 7-déc.-24 Wavelength converter 124 7-déc.-24 Passive Optical Cross-Connect Passive OXC : No conversion It switches optically all the incoming wavelengths of the input fibers to the outgoing wavelengths of the output fibers. For instance, it can switch the optical signal on incoming wavelength λi of input fiber k to the outgoing wavelength λi of output fiber m. 7-déc.-24 125 Active Optical Cross-Connect If it is equipped with converters, it can also switch the optical signal of the incoming wavelength λi of input fiber k to another outgoing wavelength λj of the output fiber m. This happens when the wavelength λi of the output fiber m is in use. Active OXC : convertor 126 7-déc.-24 Optical Systems Optical Cross-Connect Nortel OPTera photonic cross connect Optical Cross- Connect Terminating Equipment 110101 | SONET, ATM, IP... 127 7-déc.-24 Basic architecture and operation of a DWDM network This network is composed of End Nodes (noeuds d'extrémité ), of Switch Nodes (noeuds de commutation) and fiber optic links. 128 7-déc.-24 Basic architecture and operation of a DWDM network End Nodes consist of modulators/demodulators (or modems) for each channel, as well as multiplexers and demultiplexers, being respectively used for the grouping and separation of the light waves of different wavelenghts. Modulators / Demodulators convert the numerical data into lightwaves by modulation of intensity, while the demodulators reconvert the lightwaves signals in numerical data. 129 7-déc.-24