Controlling Microbial Growth in the Environment PDF
Document Details
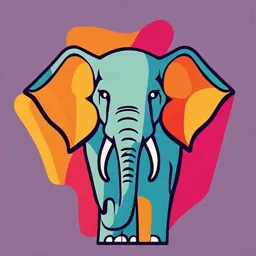
Uploaded by JawDroppingIrrational
Tags
Summary
This document provides an overview of controlling microbial growth in the environment, including key terminology, various control methods, and the mechanisms behind them. It covers different agents and their applications.
Full Transcript
**CONTROLLING MICROBIAL GROWTH IN THE ENVIRONMENT** **Terminology of Microbial Control** - **Importance of Terminology**: Correct terminology in microbial control is crucial for clarity, especially among microbiologists and healthcare workers, though often misunderstood by the public. -...
**CONTROLLING MICROBIAL GROWTH IN THE ENVIRONMENT** **Terminology of Microbial Control** - **Importance of Terminology**: Correct terminology in microbial control is crucial for clarity, especially among microbiologists and healthcare workers, though often misunderstood by the public. - **Sterilization**: - **Definition**: Complete removal or destruction of all microbes, including viruses and bacterial endospores, on an object (not prions). - **Practical Use**: Refers to the eradication of harmful microorganisms; innocuous microbes may still be present, e.g., hyperthermophiles in canned food. - **Aseptic**: - **Definition**: An environment or procedure free from contamination by pathogens. - **Application**: Common in food packaging (e.g., aseptic juice packaging) and medical procedures to prevent contamination. - **Disinfection**: - **Definition**: Use of physical or chemical agents (e.g., UV light, alcohol) to inhibit or destroy pathogens. - **Limitations**: Does not guarantee elimination of all pathogens, especially endospores; only applies to inanimate objects. - **Antisepsis**: Similar to disinfection but applied to skin or tissue, using antiseptics that are typically less concentrated than disinfectants. - **Degerming**: - **Definition**: Removal of microbes by scrubbing, often enhanced by soap or alcohol. - **Importance**: Physical scrubbing can be more effective than the chemical agents used. - **Sanitization**: - **Definition**: Disinfection to reduce pathogens in public areas to meet health standards, differing from private cleaning by location (e.g., restaurant utensils vs. home dishes). - **Pasteurization**: - **Definition**: Heat treatment to kill pathogens and reduce spoilage organisms in food and beverages (e.g., milk, juices). - **Microbial Control Types**: - **Sterilization**: Elimination of all microbes. - **Antisepsis/Disinfection**: Targets vegetative cells and many viruses; includes methods like degerming, sanitization, and pasteurization. - **Static vs. Cidal Agents**: - **Static (stasis)**: Inhibits microbial growth without killing (e.g., refrigeration is bacteriostatic). - **Cidal (cide)**: Destroys or permanently inactivates microbes (e.g., bactericides, fungicides, germicides). **Microbial Death Rates** - **Definition**: Microbial death is defined as the irreversible loss of a microorganism\'s ability to reproduce in ideal conditions. - **Microbial Death Rate**: - **Constant Rate**: Microbial death rate is typically constant over time for a specific microorganism under certain conditions. - **Example Calculation**: If an antimicrobial agent kills 90% of microbes in one minute, the death rate continues at this rate, reducing microbes by 10-fold each minute. - **Progression**: After 1 minute, 1 billion microbes reduce to 100 million; after 2 minutes, down to 10 million, and so on. - **Semilogarithmic Graph**: - **Graph Pattern**: When plotted on a semilogarithmic graph (logarithmic y-axis, arithmetic x-axis), the microbial death rate appears as a straight line, confirming its constant nature. **Action of Antimicrobial Agents** - **Categories of Antimicrobial Action**: - **Cell Structure Disruption**: Antimicrobials that damage cell walls or cytoplasmic membranes. - **Metabolism and Reproduction Interference**: Agents that affect proteins and nucleic acids, interrupting cellular functions. - **Alteration of Cell Walls and Membranes**: - **Cell Wall Function**: Protects cells in hypotonic environments by preventing bursting due to osmosis. - **Disruption Effects**: If the cell wall is damaged, water can enter the cell, leading to bursting and death. - **Cytoplasmic Membrane Role**: Contains the cytoplasm and controls chemical exchange. Damage to membrane proteins or phospholipids results in leakage of cell contents, leading to cell death. - **Effect on Enveloped Viruses**: The envelope, necessary for virus attachment, can be damaged, inhibiting viral replication. Nonenveloped viruses, lacking this membrane, are more resilient to antimicrobial agents. - **Damage to Proteins and Nucleic Acids**: - **Protein Function**: Proteins regulate metabolism, serve as enzymes, and provide structure. Their function relies on their three-dimensional shape, held by hydrogen and disulfide bonds. - **Denaturation**: Heat or chemicals can break these bonds, denaturing proteins and ceasing their function, leading to cell death. - **Nucleic Acid Alteration**: Agents like chemicals, heat, and radiation can damage nucleic acids, causing fatal mutations or inhibiting protein synthesis by interfering with ribozymes (RNA enzymes). - **Application in Microbial Control**: - Health professionals have various physical and chemical options to control microbial growth by targeting these cellular structures. **Selection of Microbial Control Methods** - **Ideal Characteristics of Antimicrobial Agents**: - Should be cost-effective, fast-acting, stable in storage, and safe for humans and animals. - No ideal agent exists; each has limitations. - **Factors Affecting Efficacy**: - **Site to Be Treated**: Choice depends on the nature of the site. - Harsh agents are unsuitable for human tissue or delicate objects. - Items penetrating the body (e.g., needles) require sterilization, while non-invasive items may only need disinfection. - **Microorganism Susceptibility**: Microbes vary in resistance to antimicrobial agents. - **Enveloped Viruses** (e.g., HIV) are more susceptible than **Nonenveloped Viruses** (e.g., poliovirus). - Hardier microbes are often targeted to ensure weaker organisms are also eradicated. - **Environmental Conditions**: Conditions at the site can impact agent effectiveness (temperature, pH, etc.). - **Resistant Microbes**: - **Bacterial Endospores**: Highly resilient, capable of surviving extreme conditions (e.g., Bacillus, Clostridium). - **Mycobacteria**: Waxy cell wall protects against drying and chemicals (e.g., *Mycobacterium tuberculosis*). - **Protozoan Cysts**: Resistant to most disinfectants and environmental stress. - **Prions**: Infectious proteins; require extreme heat (482°C for 4 hours) for denaturation. - **Classification of Germicides**: - **High-Level Germicides**: Kill all pathogens, including bacterial endospores; used for sterilizing invasive instruments (e.g., catheters). - **Intermediate-Level Germicides**: Kill fungal spores, protozoan cysts, viruses, pathogenic bacteria; used for non-invasive instruments contacting mucous membranes. - **Low-Level Germicides**: Kill vegetative bacteria, fungi, protozoa, some viruses; suitable for non-invasive items contacting only skin (e.g., furniture). **Environmental Conditions Affecting Microbial Control** - **Temperature**: Higher temperatures increase the efficacy of antimicrobial methods as they speed up chemical reactions, making disinfectants more effective when warm. - **pH**: - **Acidic Conditions**: Enhance the effect of heat as an antimicrobial method. - **Low pH**: Boosts the effectiveness of certain disinfectants, like household bleach. - **Organic Materials**: - **Interference**: Substances such as fat, feces, vomit, blood, and biofilm matrices obstruct the penetration of heat, chemicals, and some radiation. - **Inactivation of Disinfectants**: Some organic materials can inactivate disinfectants. - **Importance of Pre-cleaning**: Cleaning objects before sterilization or disinfection ensures antimicrobial agents can reach all surfaces effectively. **Biosafety Levels** - **Overview**: The CDC defines four Biosafety Levels (BSLs) to ensure safety in labs handling pathogens, with each level specifying stricter controls on techniques, equipment, and facility design. - **Biosafety Level 1 (BSL-1)**: - **Suitability**: For non-disease-causing microbes in healthy humans (e.g., *Escherichia coli*). - **Precautions**: Minimal; includes handwashing with antibacterial soap and surface disinfection. - **Biosafety Level 2 (BSL-2)**: - **Suitability**: For moderately hazardous agents (e.g., hepatitis and influenza viruses, MRSA). - **Precautions**: Limited access during experiments, extra care with contaminated sharps, and aerosol-generating procedures done in safety cabinets. - **Biosafety Level 3 (BSL-3)**: - **Suitability**: For agents causing serious or potentially lethal diseases through inhalation (e.g., tuberculosis, anthrax bacteria, yellow fever virus). - **Precautions**: All work done in HEPA-filtered safety cabinets, double-door entry, negative air pressure to contain pathogens, and HEPA-filtered exhaust. - **Biosafety Level 4 (BSL-4)**: - **Suitability**: For high-risk, life-threatening agents with no treatment or vaccine (e.g., Ebola, smallpox, Lassa fever viruses). - **Precautions**: Complete isolation, electronically sealed airlocks, multiple decontamination steps (e.g., showers, UV light), filtered air and water, personnel in pressurized, air-supplied \"space suits.\" **Physical Methods of Microbial Control** - **Overview**: Physical methods to control microbes include heat, cold, desiccation, filtration, osmotic pressure, and radiation. - **Heat-Related Methods**: - **Effects of Heat**: Denatures proteins, disrupts cell walls/membranes, and damages nucleic acids. Used for sterilization and food preservation. - **Thermal Death Point**: Lowest temperature that kills all cells in 10 minutes. - **Thermal Death Time**: Time required to sterilize a liquid at a set temperature. - **Decimal Reduction Time (D-value)**: Time needed to reduce microbes by 90%, useful for food safety (e.g., *Clostridium botulinum* in canning). - **Moist Heat**: - **Boiling**: Kills vegetative cells of bacteria, fungi, protozoa, and most viruses within 10 minutes; not effective for sterilization as some endospores and viruses can survive. - **Autoclaving**: Uses steam under pressure (121°C and 15 psi) for 15 minutes to achieve true sterilization. Effective for laboratory and medical materials. - **Pasteurization**: Heat treatment for food products like milk to destroy pathogens without affecting flavor. - **Flash Pasteurization**: 72°C for 15 seconds. - **Ultra-High-Temperature Pasteurization**: 135°C for 1 second. - **Ultra-High-Temperature Sterilization**: Superheats liquid to 140°C for 1-3 seconds, allowing indefinite storage without refrigeration. - **Dry Heat**: - **Application**: Used for materials that cannot be sterilized by moist heat (e.g., powders, oils). - **Requirements**: Higher temperatures and longer times than moist heat (e.g., 171°C for 1 hour). - **Incineration**: Complete sterilization by burning; used for microbiology tools and contaminated materials (e.g., incinerating infectious waste). **Refrigeration and Freezing as Methods of Microbial Control** - **Learning Outcome**: Understanding how refrigeration and freezing control microbial growth, especially in food storage. - **Effect on Microbes**: - **Refrigeration (0°C to 7°C)**: Slows down microbial metabolism, growth, and reproduction, as most chemical reactions are slower at low temperatures. - **Freezing (below 0°C)**: Halts growth and makes liquid water unavailable, further inhibiting microbial processes. However, **psychrophilic** (cold-loving) microbes can still grow in refrigerated food and spoil it. - **Pathogen Control**: - **Refrigeration**: Effective for halting most pathogens, which are typically **mesophiles** (moderate-temperature-loving). - **Exceptions**: *Listeria* can reproduce in refrigerated food, and *Yersinia* can multiply in refrigerated blood, posing risks in certain scenarios. - **Freezing Techniques**: - **Slow Freezing**: Forms ice crystals that puncture cell membranes, making it more effective in inhibiting microbes than quick freezing. - **Microbial Survivability**: Some bacteria, endospores, and viruses can survive freezing for years; scientists store microbes at -30°C to -80°C for long-term preservation. - **Food Safety Note**: Proper thawing and cooking of frozen foods are crucial, as they may still harbor viable pathogens despite freezing. **Desiccation, Lyophilization, and Filtration as Methods of Microbial Control** - **Desiccation (Drying)**: - **Purpose**: Long-used method to preserve foods (e.g., fruits, grains, nuts). - **Mechanism**: Inhibits microbial growth by removing water, which is essential for metabolism. - **Effectiveness**: Reduces pathogen spread (e.g., bacteria causing syphilis and pneumonia), but some molds can grow on foods with low moisture. - **Lyophilization (Freeze-Drying)**: - **Process**: Combines freezing and drying to preserve microbes long-term. - **Technique**: Culture is instantly frozen in liquid nitrogen or dry ice, followed by a vacuum to remove frozen water via sublimation (solid to gas). - **Outcome**: Prevents damaging ice crystal formation; allows reconstitution of viable cells even years later. - **Filtration**: - **Purpose**: Separates cells/viruses from fluids, useful for sterilizing heat-sensitive materials. - **Mechanism**: Fluids pass through filters with pores that trap larger particles, while smaller particles pass through. - **Historical Note**: Early filters could not trap small viruses, which were termed \"filterable viruses.\" - **Applications**: Sterilizes antibiotics, vaccines, ophthalmic solutions, and culture media. - **Materials**: Modern filters made from nitrocellulose/plastic, with pore sizes ranging from 25 mm to 0.01 mm. - **Health and Safety Use**: Prevents airborne contamination; includes HEPA filters in safety cabinets, operating rooms, and rooms for patients with airborne diseases. **Osmotic Pressure and Radiation as Methods of Microbial Control** - **Osmotic Pressure**: - **Mechanism**: High concentrations of salt or sugar create a hypertonic environment, causing cells to lose water through osmosis, inhibiting metabolism. - **Applications**: Preserves foods such as honey, jerky, jams, and pickles. - **Limitations**: Most bacteria are inhibited, but fungi can tolerate hypertonic environments, allowing them to grow on certain dried foods. - **Radiation**: - **Types**: Radiation methods include both **ionizing** (high energy) and **nonionizing** (lower energy) radiation. - **Ionizing Radiation**: - **Mechanism**: Includes electron beams, gamma rays, and X-rays with wavelengths shorter than 1 nm. They eject electrons from atoms, creating ions that damage DNA and cause fatal mutations. - **Applications**: Electron beams sterilize medical supplies and food in seconds, while gamma rays (emitted by radioactive elements) penetrate deeper, used for meats, spices, fruits, and vegetables. X-rays penetrate deeply but are impractical for sterilization due to long exposure times. - **Public Concerns**: Some consumers fear radiation in food, but studies confirm irradiated foods remain non-radioactive, safe, and nutritious. - **Nonionizing Radiation**: - **Mechanism**: UV light is the primary form used, with wavelengths over 1 nm, which excites electrons, causing changes in proteins and DNA. - **Applications**: UV light causes pyrimidine dimers in DNA, inhibiting accurate DNA replication and transcription. Effective for disinfecting air, fluids, and surfaces. Common in sewage treatment for reducing bacterial content without chlorine. **Chemical Methods of Microbial Control** - **Overview**: Chemical agents are commonly used for disinfection, antisepsis, and preservation, targeting cell walls, membranes, proteins, and DNA. Effectiveness varies based on factors such as temperature, pH, concentration, and exposure duration. Generally more effective on enveloped viruses and vegetative cells than on spores and cysts. - **Types of Chemical Agents**: - **Phenols and Phenolics**: - **Mechanism**: Denature proteins and disrupt cell membranes. - **Examples**: Phenol, chlorinated phenolics, and bisphenolics (e.g., triclosan in consumer products). - **Uses**: Effective even in the presence of organic material; widely used in health care and households. - **Limitations**: Strong odor and potential skin irritation. - **Alcohols**: - **Mechanism**: Denature proteins and disrupt membranes; effective against bacteria, fungi, and enveloped viruses. - **Examples**: Ethanol, isopropanol (rubbing alcohol). - **Concentration**: 70-90% solutions are optimal; pure alcohol is less effective. - **Uses**: Hand antiseptics, skin swabbing before injections. - **Limitations**: Ineffective against some spores and viruses (e.g., norovirus); evaporates quickly. - **Halogens**: - **Elements**: Iodine, chlorine, bromine, and fluorine. - **Mechanism**: Denature proteins and enzymes. - **Uses**: - **Iodine**: Used as a tincture or iodophor (e.g., Betadine for surgical preparation). - **Chlorine**: Disinfects water, pools, and waste; compounds like sodium hypochlorite are household disinfectants. - **Bromine**: Ideal for hot tubs and pool disinfection. - **Fluorine**: Fluoride in water/toothpaste reduces dental caries. - **Limitations**: Some cysts and spores are resistant to low concentrations; chloramines (chlorine-ammonia compounds) are slower acting but longer lasting. - **Oxidizing Agents** - **Mechanism of Action**: Oxidizing agents, including peroxides, ozone, and peracetic acid, kill microbes by oxidizing their enzymes, effectively disrupting microbial metabolism. - **High-Level Disinfectants and Antiseptics**: These agents are particularly effective against anaerobic microorganisms and are widely used in healthcare. - **Hydrogen Peroxide (H₂O₂)**: - Common household disinfectant and sterilizer for surfaces and inanimate objects. - Ineffective for open wounds because the catalase enzyme from damaged human cells neutralizes it quickly. - Used in food processing, such as hot hydrogen peroxide for sterilizing juice packages. - **Ozone (O₃)**: - Formed by electrical discharge on molecular oxygen, giving a fresh scent after thunderstorms. - Used in water treatment (e.g., in Canada and Europe) as an alternative to chlorine, though more costly and difficult to maintain. - **Peracetic Acid**: - Potent sporicide that sterilizes surfaces effectively without leaving toxic residues. - Commonly used by food processors and medical personnel due to resistance to organic contaminants. - **Surfactants** - **Definition and Action**: Surfactants are \"surface-active\" chemicals that reduce surface tension, making solvents (like water) more effective at dissolving solute molecules. - **Types of Surfactants in Microbial Control**: - **Soaps**: - Have a hydrophobic end (fatty acids) that breaks down oily deposits and a hydrophilic end that attracts water. - Primarily act as degerming agents rather than antimicrobial agents; they help wash away bacteria. - Antimicrobial soaps are effective due to added antimicrobial chemicals. - **Synthetic Detergents (Quaternary Ammonium Compounds or Quats)**: - Composed of positively charged ammonium ions. - More soluble in water than soaps and are commonly used in industrial and medical settings. - Examples: Benzalkonium chloride and cetylpyridinium (found in products like Zephiran and Cepacol mouthwash). - Effective as bactericidal (especially against Gram-positive bacteria), fungicidal, and virucidal against enveloped viruses. - Ineffective against nonenveloped viruses, mycobacteria, and endospores. - **Mode of Action**: - Quats disrupt cellular membranes, causing affected cells to lose essential ions (e.g., potassium). - **Limitations**: - Quats are inactivated by organic contaminants and soaps. - Some pathogens, such as *Pseudomonas aeruginosa*, can thrive in quats, so they are considered low-level disinfectants. - **Heavy Metals** - **Definition and Function**: - Heavy-metal ions include high-density metals like arsenic, zinc, mercury, silver, and copper. - These ions are antimicrobial because they bind with sulfur in cysteine amino acids, leading to protein denaturation and functional inhibition. - Heavy metals are low-level bacteriostatic (bacteria-inhibiting) and fungistatic (fungi-inhibiting) agents. - **Applications**: - **Silver Nitrate**: - Previously used in newborns' eyes to prevent blindness caused by *Neisseria gonorrhoeae*. - Replaced by less irritating antimicrobial ointments in hospitals. - Silver is still used in some surgical dressings, burn creams, and catheters for its antimicrobial properties. - **Thimerosal (Mercury Compound)**: - Used for over 70 years as a preservative in vaccines. - Alternatives are now preferred due to mercury's toxicity; however, small amounts are still considered safe in some vaccines (e.g., influenza in multi-dose vials). - **Copper**: - Used as an algicide in reservoirs, fish tanks, swimming pools, and water storage tanks at low concentrations (as low as 1 ppm). - Copper, along with zinc or mercury, is also used in mildew-preventative paints. - **Limitations**: - Heavy metals have been largely replaced by more effective antimicrobial agents. - Mercury-containing compounds like thimerosal are restricted due to toxicity concerns. - **Aldehydes** - **Definition**: - Aldehydes are organic compounds with terminal ---CHO groups. - Common aldehydes in microbial control include glutaraldehyde (liquid) and formaldehyde (gas). - **Mechanism**: - They work by cross-linking functional groups (amino, hydroxyl, sulfhydryl, carboxyl) in proteins and nucleic acids. - This action denatures proteins and inactivates nucleic acids, leading to microbial death. - **Uses**: - **Glutaraldehyde**: - A 2% solution can disinfect most objects within 10 minutes and sterilizes with prolonged exposure (10 hours). - Less irritating and more effective than formaldehyde but also more expensive. - **Formaldehyde**: - Used as a 37% solution (formalin) for embalming and disinfecting hospital rooms, instruments, and machines. - Requires careful handling due to its potential to irritate mucous membranes and its carcinogenic properties. - **Gaseous Agents** - **Definition**: - Gaseous agents include highly reactive microbicidal and sporicidal gases like ethylene oxide, propylene oxide, and beta-propiolactone. - **Mechanism**: - These gases penetrate materials, cross-linking and denaturing proteins and DNA, ensuring comprehensive sterilization. - **Uses**: - Ideal for sterilizing heat- and moisture-sensitive items, such as medical and lab equipment, heart-lung machine components, and electronic devices. - **Ethylene Oxide**: - Common in hospitals, dental offices, and even used by NASA to sterilize spacecraft. - Requires specialized chambers for application, similar to autoclaves. - **Drawbacks**: - Gaseous agents are highly explosive, toxic, and often carcinogenic (especially beta-propiolactone). - Post-sterilization, treated items must be thoroughly aired to remove toxic residues, which increases processing time. - **Enzymes** - **Definition**: - Antimicrobial enzymes are proteins that act against microorganisms. - **Examples and Functions**: - **Lysozyme**: - Found in human tears, it digests bacterial cell walls (specifically peptidoglycan), causing bacterial cells to rupture. - Used in food processing (e.g., cheese) to reduce bacterial count and in winemaking to prevent spoilage by bacteria, substituting for sulfur dioxide. - **Innovative Applications**: - **Prionzyme**: - Developed to target and remove prions (infectious proteins) that cause variant Creutzfeldt-Jakob disease (vCJD), a highly resistant pathogen. - Approved by the European Union in 2006 as a noncaustic, safe method to remove prions from medical instruments. - **Antimicrobial Drugs** - **Definition**: - Antimicrobial drugs include naturally produced antibiotics, semisynthetic derivatives, and synthetic drugs. - Unlike chemical agents used in environmental microbial control, these are primarily for disease treatment. - **Types and Uses**: - **Antibiotics**: - Naturally produced by microorganisms. - **Semisynthetics**: - Chemically modified antibiotics, enhancing their efficacy or spectrum. - **Synthetics**: - Fully lab-developed antimicrobials. - **Applications Beyond Treatment**: - Some antimicrobial drugs, such as **nisin** and **natamycin**, are also used outside of human treatment, particularly in food preservation to prevent bacterial and fungal growth, respectively, in cheese and other foods. **Methods for Evaluating Disinfectants and Antiseptics** - **General Considerations**: - Higher concentrations and fresher solutions are more effective, but high concentrations and prolonged exposure may damage surfaces or be harmful to living beings. - **Methods for Measuring Efficacy**: - **Phenol Coefficient**: - **Definition**: Compares a disinfectant's effectiveness to that of phenol. - **Evaluation**: A coefficient greater than 1 indicates greater effectiveness than phenol. - **Limitations**: Less relevant with the development of more effective agents than phenol. - **Use-Dilution Test**: - **Process**: Metal cylinders contaminated with bacteria are exposed to different dilutions of a disinfectant. The highest dilution that prevents growth after incubation is considered most effective. - **Status**: Current standard in the U.S.; however, some question its relevance for today's pathogens. - **Kelsey-Sykes Capacity Test**: - **Process**: Bacteria are exposed to a disinfectant, and samples are periodically moved to a growth medium. Lack of bacterial growth indicates effective disinfection time. - **Usage**: Common in the EU as a measure of a disinfectant\'s effectiveness over time. - **In-Use Test**: - **Process**: Swabs are taken from surfaces before and after disinfectant application, then incubated to detect microbial growth. - **Advantages**: Provides realistic results for specific environments but is time-consuming. - **Concerns Over Resistant Microbes**: - **Trend**: Overuse of disinfectants and antiseptics (found in various consumer products) may contribute to the development of resistant microbial strains. - **Implications**: Resistance in pathogens like *M. tuberculosis*, *P. aeruginosa*, *E. coli*, and *S. aureus* highlights the need for cautious use of antimicrobial chemicals. **CONTROLLING MICROBIAL GROWTH IN THE ENVIRONMENT WITH ANTIMICROBIAL DRUGS** - **Definition of Antimicrobial Drugs**: - Drugs affecting any physiological process are termed *drugs*, while those treating diseases are *chemotherapeutic agents*. Specifically, *antimicrobial agents* combat infections. - **Key Terms**: - **Antibiotics**: Naturally occurring antimicrobial chemicals produced by microorganisms. - **Semisynthetics**: Chemically modified antibiotics, optimized for efficacy or administration. - **Synthetics**: Fully lab-developed antimicrobial drugs. - **History of Antimicrobial Agents**: - **Early 1900s Medicine**: Limited to diagnosis and prognosis, with minimal options for pathogen inhibition. - **Paul Ehrlich**: Proposed *chemotherapy* and envisioned "magic bullets" targeting pathogens without harming the host; discovered arsenic compounds effective against syphilis. - **Alexander Fleming**: Discovered penicillin in 1928, noting its antibacterial effects from the *Penicillium* mold. - **Sulfanilamide**: Discovered by Gerhard Domagk in 1932, became the first widely used antimicrobial agent effective against various bacterial infections. - **Selman Waksman**: Discovered other antibiotic sources, particularly *Streptomyces* bacteria; coined the term *antibiotics* for naturally produced antibacterial agents. - **Modern Development**: - **Antibiotics Today**: Typically refers to antibacterial agents, including synthetics and semisynthetics. - **Common Usage**: Most antimicrobials used today are natural or semisynthetic, with synthetic options also developed for specific needs. **Mechanisms of Antimicrobial Action** - **Selective Toxicity**: The principle that effective antimicrobial agents are more toxic to the pathogen than to the host, enabled by structural or metabolic differences between them. Greater differences allow easier development of specific drugs. - **Categories of Antimicrobial Agents**: - **Antibacterial Drugs**: Most diverse, targeting bacteria's unique structures/metabolisms compared to eukaryotic hosts. - **Antifungal, Antiprotozoan, and Anthelmintic Drugs**: Fewer available due to similarities between pathogens (eukaryotic chemoheterotrophs) and animal hosts. - **Antiviral Drugs**: Limited options because viruses rely on host cell machinery, making targeted treatment challenging without harming the host. - **Mechanisms of Action**: - **Inhibit Cell Wall Synthesis**: Target fungal and bacterial cells with cell walls, sparing animals, which lack cell walls. - **Inhibit Protein Synthesis**: Exploit differences between prokaryotic and eukaryotic ribosomes to target bacterial translation. - **Disrupt Cytoplasmic Membranes**: Act on unique components of microbial membranes. - **Inhibit Metabolic Pathways**: Target pathways absent in humans, selectively impacting pathogens. - **Inhibit Nucleic Acid Synthesis**: Prevent replication and transcription in pathogens. - **Block Host Recognition/Attachment**: Prevent pathogens from attaching to or recognizing host cells. **Inhibition of Cell Wall, Protein Synthesis, and Other Cellular Processes as Antimicrobial Mechanisms** **1. Inhibition of Cell Wall Synthesis** - **Bacterial Cell Walls**: Composed of peptidoglycan with NAM-NAG chains. - **Beta-Lactams (e.g., penicillins, cephalosporins)**: Bind to enzymes that cross-link NAM subunits, weakening cell walls and causing cell lysis. - **Semisynthetic Beta-Lactams (e.g., methicillin)**: Modified for improved stability, absorption, and spectrum. - **Vancomycin, Cycloserine, Bacitracin**: Target specific parts of peptidoglycan or its transport across the membrane. - **Mycobacteria (e.g., tuberculosis)**: Drugs like isoniazid target the unique mycolic acid in their walls. - **Fungal Cell Walls**: Echinocandins (e.g., caspofungin) inhibit glucan synthesis, weakening fungal cell walls and causing osmotic rupture. **2. Inhibition of Protein Synthesis** - **Targeting Ribosomal Subunits**: - **30S Inhibitors**: - *Aminoglycosides* alter shape, preventing mRNA reading. - *Tetracyclines* block the tRNA docking site. - **50S Inhibitors**: - *Chloramphenicol* blocks the peptide bond formation site. - *Macrolides* prevent ribosome movement along mRNA. - **Antisense Nucleic Acids**: Complementary to pathogen mRNA, blocking translation (e.g., fomivirsen for cytomegalovirus). - **Oxazolidinones**: Inhibit translation initiation in resistant Gram-positive infections. - **tRNA Charging Inhibition**: Mupirocin blocks isoleucyl-tRNA synthetase in Gram-positive bacteria, halting protein synthesis. **3. Disruption of Cytoplasmic Membranes** - **Bacterial Membranes**: Polymyxin disrupts Gram-negative bacteria's membranes but is toxic to human kidneys. - **Fungal and Parasitic Membranes**: - *Polyenes* (e.g., amphotericin B) bind to ergosterol in fungal membranes, causing cell lysis. - *Azoles and Allylamines* inhibit ergosterol synthesis, destabilizing fungal membranes. - **Antiparasitic Drugs**: Praziquantel and ivermectin increase permeability in parasitic worms' membranes. **4. Inhibition of Metabolic Pathways** - **Pathway-Specific Drugs**: - *Atovaquone*: Disrupts electron transport in protozoa and fungi. - *Sulfonamides*: Block PABA to folic acid conversion, crucial for DNA/RNA synthesis in bacteria. - *Trimethoprim*: Inhibits a later step in folic acid synthesis. - **Antiviral Agents**: - *Amantadine*: Prevents viral uncoating in cells. - *Protease Inhibitors*: Block HIV protease, crucial for viral replication. **5. Inhibition of Nucleic Acid Synthesis** - **Bacterial DNA/RNA Targeting**: - *Quinolones* inhibit DNA gyrase, essential for bacterial DNA replication. - *Rifampin*: Binds bacterial RNA polymerase, blocking transcription. - **Nucleotide/Nucleoside Analogs**: Resemble nucleotides, disrupting viral replication (e.g., AZT for HIV). - **Protozoan DNA Targeting**: Pentamidine interferes with protozoan DNA replication. - **Reverse Transcriptase Inhibitors**: Specific to retroviruses like HIV, blocking reverse transcription without harming humans. **Prevention of Virus Attachment, Entry, or Uncoating** - **Virus-Host Attachment**: Many viruses bind to host cells using specific interactions between viral attachment proteins and complementary host cell receptors. - **Attachment Antagonists**: - **Mechanism**: Analog compounds of either attachment or receptor proteins block viral binding sites, preventing attachment and subsequent infection. - **Example**: *Pleconaril*, a synthetic antagonist, blocks receptors for picornaviruses (e.g., cold viruses, poliovirus, and coxsackievirus), hindering virus attachment and infection. - **Prevention of Viral Uncoating**: - **Example**: *Arildone* is an antiviral drug that prevents the uncoating of poliovirus by blocking the removal of its capsid, thereby stopping the replication cycle. **Clinical Considerations in Prescribing Antimicrobial Drugs** - **Ideal Antimicrobial Agent Qualities**: - Should be readily available, inexpensive, chemically stable, easy to administer, non-toxic, non-allergenic, and selectively toxic to pathogens. - No current antimicrobial has all these qualities, so clinical evaluation is necessary. - **Spectrum of Action**: - **Broad-Spectrum Drugs**: Effective against a wide range of pathogens (e.g., tetracycline works against Gram-negative, Gram-positive, chlamydias, and rickettsias). - **Narrow-Spectrum Drugs**: Target only a few types of pathogens (e.g., penicillin is more effective against Gram-positive bacteria due to limited penetration in Gram-negative bacteria). - **Considerations**: Broad-spectrum drugs can lead to secondary infections or superinfections by disrupting normal microbiota. For example, using erythromycin for strep throat could cause Candida albicans overgrowth due to reduced microbial antagonism. **Methods for Assessing Antimicrobial Effectiveness** - **Purpose**: Determining the most effective antimicrobial agent and its potency against a specific pathogen. 1. **Diffusion Susceptibility Test (Kirby-Bauer Test)**: - Procedure: A pathogen-inoculated Petri plate is treated with paper disks containing antimicrobials. - Outcome: Zone of inhibition (clear area around the disk) indicates effectiveness. - Evaluation: Diameter of the zone is measured and compared to a standard table to classify the pathogen as susceptible, intermediate, or resistant to each drug. 2. **Minimum Inhibitory Concentration (MIC) Test**: - Purpose: Determines the smallest concentration of a drug that inhibits pathogen growth. - Method: Serial dilutions of the antimicrobial agent are combined with bacteria in broth; turbidity indicates growth, while clear solutions show inhibition. - Variants: The Etest uses a gradient strip on an inoculated plate to find the MIC directly. 3. **Minimum Bactericidal Concentration (MBC) Test**: - Objective: Establishes the drug concentration required to kill the bacteria (bactericidal effect). - Method: Samples from clear MIC tubes or zones of inhibition are transferred to drug-free media; any bacterial regrowth indicates bacteriostatic levels, while no growth signifies the minimum bactericidal concentration (MBC). **Clinical Considerations for Prescribing Antimicrobial Drugs** 1. **Routes of Administration**: - **Topical/Local**: Used for external infections (e.g., athlete\'s foot), applied directly. - **Oral**: Easiest, no needles required; however, blood concentration of the drug remains lower, and patient compliance with prescribed schedules can be inconsistent. - **Intramuscular (IM)**: Administered via needle into muscle tissue, achieving a moderate drug concentration. - **Intravenous (IV)**: Delivers drugs directly into the bloodstream, leading to a high initial concentration, though it diminishes quickly without continuous administration. *Considerations*: The route of administration depends on factors such as drug absorption rate, infection site, and potential barriers (e.g., blood-brain barrier in brain infections). 2. **Safety and Side Effects**: - **Toxicity**: Many antimicrobials can have toxic side effects on organs such as the kidneys or liver. Physicians must consider these effects, particularly for vulnerable groups like pregnant women. The therapeutic index (TI) and therapeutic window indicate a drug's safety margin. - **Allergic Reactions**: Some individuals may experience mild to severe allergic responses, including anaphylactic shock. Patients with mild reactions to drugs such as penicillin may outgrow their sensitivity. - **Disruption of Normal Microbiota**: Broad-spectrum antibiotics can disrupt microbial balance, allowing opportunistic infections. For example, Clostridium difficile infections can result from long-term antibiotic use. **Resistance to Antimicrobial Drugs** 1. **Development of Resistance in Populations**: - Resistance can emerge in bacteria through genetic mutations or by acquiring resistance genes on R plasmids via horizontal gene transfer (transformation, transduction, or conjugation). - In the absence of antimicrobials, resistant bacteria often grow slower due to the energy cost of maintaining resistance mechanisms. When antimicrobials are present, however, sensitive bacteria are killed, allowing resistant ones to thrive and become dominant. 2. **Mechanisms of Resistance**: - **Enzyme Production**: Resistant bacteria may produce enzymes like beta-lactamases that deactivate drugs, especially beta-lactam antibiotics (e.g., penicillins). - **Altered Entry Channels**: Changes in cell membrane proteins can prevent drugs from entering cells. This is common in Gram-negative bacteria. - **Target Alteration**: Bacteria may alter drug targets, so the drug no longer binds effectively. This can happen with protein synthesis inhibitors like erythromycin. - **Metabolic Pathway Modification**: Resistant bacteria can alter their metabolic processes, such as producing additional enzymes or abandoning certain pathways, to reduce a drug\'s effectiveness. - **Efflux Pumps**: Bacteria may use ATP-powered pumps to expel drugs from the cell, conferring resistance to multiple drugs. - **Biofilm Formation**: Biofilms slow drug penetration and alter bacterial metabolism, making antimicrobials less effective. - **Decoy Proteins**: Some bacteria, like *Mycobacterium tuberculosis*, produce proteins that act as decoys for drug targets, blocking drug effects without altering the actual target. 3. **Spread of Resistance**: - Resistance often spreads via gene transfer in crowded conditions like biofilms. Multiple resistance genes can transfer simultaneously, enhancing the spread of multi-drug resistance. **Multiple Resistance, Cross Resistance, and Strategies to Combat Resistance** 1. **Multiple and Cross Resistance**: - **Multiple Resistance**: Pathogens can develop resistance to several drugs, often by acquiring R plasmids carrying multiple resistance genes. This occurs frequently in healthcare settings where various antimicrobials are routinely used, leading to strains resistant to three or more drugs (commonly referred to as \"superbugs\"). - **Cross Resistance**: When resistance to one drug confers resistance to other drugs with similar structures. For example, resistance to one aminoglycoside like streptomycin can lead to resistance to other aminoglycosides. 2. **Strategies to Prevent or Retard Resistance**: - **Maintaining Proper Drug Concentration**: Ensuring adequate concentration of the drug in the patient's system for a sufficient duration to completely inhibit pathogens and prevent survival of partially resistant cells. - **Combination Therapy**: Using two or more antimicrobials to target a pathogen, with some combinations enhancing each other's effects (synergism). This reduces the chance of resistance since pathogens would need to simultaneously develop resistance to multiple agents. - **Limiting Unnecessary Use**: Restricting antimicrobial use to cases where they are truly needed. Unnecessary prescriptions, such as for viral infections like colds, contribute to resistance without offering therapeutic benefit. - **Developing New Drugs**: Scientists continually develop new or modified drugs (e.g., second and third-generation antimicrobials) to overcome resistance. This includes searching for novel antibiotics in unique environments and designing drugs that target specific microbial structures. 3. **Future Directions in Antimicrobial Drug Discovery**: - Researchers are exploring targeted approaches, such as developing drugs that: - Inhibit bacterial secretion systems needed for infection. - Block bacterial attachment to host cells. - Disrupt biofilm signaling, weakening biofilm-associated resistance. - Specifically target bacterial RNA polymerase to interfere with transcription.