Climate Change Issue 2023 PDF
Document Details
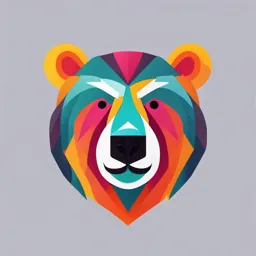
Uploaded by PatientRubellite
2023
Tags
Summary
This document explores the factors contributing to climate change, including greenhouse gases and climate feedbacks. It discusses policies and programs for adapting to climate change. The study resources are geared towards a secondary school level.
Full Transcript
1 NCF-Envirothon 2023 New Brunswick Adapting to a Changing Climate Current Environmental Issue Study Resources – Part A Table of Contents Key Topic 1: Factors Contributing to a Changing Climate 3 Key Topic 2: Measuring and Monitoring a Changing Climate 49 Key Topic 3: Risks and Impacts to Natural Re...
1 NCF-Envirothon 2023 New Brunswick Adapting to a Changing Climate Current Environmental Issue Study Resources – Part A Table of Contents Key Topic 1: Factors Contributing to a Changing Climate 3 Key Topic 2: Measuring and Monitoring a Changing Climate 49 Key Topic 3: Risks and Impacts to Natural Resources and Society from a Changing Climate 62 Key Topic 4: Policies and Programs for Adapting to a Changing Climate 2 110 Key Topic 1: Factors Contributing to a Changing Climate 1. Describe climate change and the process through which it occurs. 2. Outline the factors, both anthropogenic and natural, which influence climate and climate change. 3. Describe the major economic sectors that contribute to greenhouse gas (GHG) emissions. 4. Describe major energy sources and explain how each contributes to climate change. 5. Outline indicators of climate change. Study Resources Resource Title Source Located on Basics of Climate Change US Environmental Protection Agency, 2019 Pages 4-9 Causes of Climate Change Government of Canada, 2019 Pages 10-12 VIDEO: Climate Change 2022: Impacts, Adaptation & Vulnerability – CLICK LINK Intergovernmental Panel on Climate Change (IPCC), 2020 Page 30; 14 Minutes Sector by sector: where do global greenhouse emissions come from? Our World in Data, Hannah Ritchie, 2020 Pages 31-36 Energy and the Environment Explained US Energy Information Administration, 2021 Pages 37-48 *Please Note: Hyperlinks found in text are not considered required reading; however, included video links are required to watch.* Study Resources begin on the next page 3 Basics of Climate Change Learn about some of the key concepts related to climate change: The Greenhouse Effect Key Greenhouse Gases Other Greenhouse Gases Aerosols Climate Feedback How is the Climate Changing in the United States? Observations across the United States and world provide multiple, independent lines of evidence that climate change is happening now. Learn More About Climate Change Indicators >> The earth's climate is changing. Multiple lines of evidence show changes in our weather, oceans, and ecosystems, such as: Changing temperature and precipitation patterns.1 2 Increases in ocean temperatures, sea level, and acidity. Melting of glaciers and sea ice.3 Changes in the frequency, intensity, and duration of extreme weather events. Shifts in ecosystem characteristics , like the length of the growing season, timing of flower blooms, and migration of birds. These changes are due to a buildup of greenhouse gases in our atmosphere and the warming of the planet due to the greenhouse effect. 4 The Greenhouse Effect The greenhouse effect helps trap heat from the sun, which keeps the temperature on earth comfortable. But people’s activities are increasing the amount of heat-trapping greenhouse gases in the atmosphere, causing the earth to warm up. The earth's temperature depends on the balance between energy entering and leaving the planet’s system. When sunlight reaches the earth’s surface, it can either be reflected back into space or absorbed by the earth. Incoming energy that is absorbed by the earth warms the planet. Once absorbed, the planet releases some of the energy back into the atmosphere as heat (also called infrared radiation). Solar energy that is reflected back to space does not warm the earth. Certain gases in the atmosphere absorb energy, slowing or preventing the loss of heat to space. Those gases are known as “greenhouse gases.” They act like a blanket, making the earth warmer than it would otherwise be. This process, commonly known as the “greenhouse effect,” is natural and necessary to support life. However, the recent buildup of greenhouse gases in the atmosphere from human activities has changed the earth's climate and resulted in dangerous effects to human health and welfare and to ecosystems. 5 Key Greenhouse Gases Most of the warming since 1950 has been caused by human emissions of greenhouse gases. 4 Greenhouse gases come from a variety of human activities, including burning fossil fuels for heat and energy, clearing forests, fertilizing crops, storing waste in landfills, raising livestock, and producing some kinds of industrial products. Carbon Dioxide Carbon dioxide is the primary greenhouse gas contributing to recent climate change. Carbon dioxide enters the atmosphere through burning fossil fuels, solid waste, trees, and other biological materials, and as a result of certain chemical reactions, such as cement manufacturing. Carbon dioxide is absorbed and emitted naturally as part of the carbon cycle, through plant and animal respiration, volcanic eruptions, and ocean atmosphere exchange. The Carbon Cycle The carbon cycle is the process by which carbon continually moves from the atmosphere to the earth and then back to the atmosphere. On the earth, carbon is stored in rocks, sediments, the ocean, and in living organisms. Carbon is released back into the atmosphere when plants and animals die, as well as when fires burn, volcanoes erupt, and fossil fuels (such as coal, natural gas, and oil) are combusted. The carbon cycle ensures there is a balanced concentration of carbon in the different reservoirs on the planet. But a change in the amount of carbon in one reservoir affects all the others. Today, people are disturbing the carbon cycle by burning fossil fuels, which release large amounts of carbon dioxide into the atmosphere, and through land use changes that remove plants, which absorb carbon from the atmosphere. Methane Both natural and human activities produce methane. For example, natural wetlands, agricultural activities, and fossil fuel extraction and transport all emit methane. Nitrous Oxide Nitrous oxide is produced mainly through agricultural activities and natural biological processes. Fossil fuel burning and industrial processes also create nitrous oxide. 6 F-Gases Chlorofluorocarbons, hydrochlorofluorocarbons, hydrofluorocarbons, perfluorocarbons, and sulfur hexafluoride, together called F-gases , are often used in coolants, foaming agents, fire extinguishers, solvents, pesticides, and aerosol propellants. Global Warming Potential Different greenhouse gases can remain in the atmosphere for different amounts of time, ranging from a few years to thousands of years. In addition, some gases are more effective than others at making the planet warmer. Learn more about Global Warming Potential (GWP) , a measure of climate impacts based on how long each greenhouse gas remains in the atmosphere and how strongly it absorbs energy. Other Greenhouse Gases Ground-Level Ozone Ground-level ozone is created by a chemical reaction between emissions of nitrogen oxides and volatile organic compounds from automobiles, power plants, and other industrial and commercial sources in the presence of sunlight. In addition to trapping heat, ground-level ozone is a pollutant that can cause respiratory health problems and damage crops and ecosystems. Water Vapor Water vapor is another greenhouse gas and plays a key role in climate feedbacks because of its heat-trapping ability. Warmer air holds more moisture than cooler air. Therefore, as greenhouse gas concentrations increase and global temperatures rise, the total amount of water vapor in the atmosphere also increases, further amplifying the warming effect.5 For more information on greenhouse gases, see Greenhouse Gas Emissions. 7 Aerosols Aerosols in the atmosphere can affect climate. Aerosols are microscopic (solid or liquid) particles that are so small that instead of quickly falling to the surface like larger particles, they remain suspended in the air for days to weeks. Human activities, such as burning fossil fuels and biomass, contribute to emissions of these substances, although some aerosols also come from natural sources such as volcanoes and marine plankton. Unlike greenhouse gases, the climate effects of aerosols vary depending on what they are made of and where they are emitted. Depending on their color and other factors, aerosols can either absorb or reflect sunlight. Aerosols that reflect sunlight, such as particles from volcanic eruptions or sulfur emissions from burning coal, have a cooling effect. Those that absorb sunlight, such as black carbon (a part of soot), have a warming effect. Not only can black carbon directly absorb incoming and reflected sunlight, but it can also absorb infrared radiation.6 Black carbon can also be deposited on snow and ice, darkening the surface and thereby increasing the snow's absorption of sunlight and accelerating melt.7 While reductions in all aerosols can lead to more warming, targeted reductions in black carbon emissions can reduce global warming. Warming and cooling aerosols can also interact with clouds, changing their ability to form and dissipate, as well as their reflectivity and precipitation rates. Clouds can contribute both to cooling, by reflecting sunlight, and warming, by trapping outgoing heat. Climate Feedbacks Climate feedbacks are natural processes that respond to global warming by setting or further increasing change in the climate system. Feedbacks that set the change in climate are called negative feedbacks. Feedbacks that amplify changes are called positive feedbacks. Water vapor appears to cause the most important positive feedback. As the earth warms, the rate of evaporation and the amount of water vapor in the air both increase. Because water vapor is a greenhouse gas, this leads to further warming. The melting of Arctic sea ice is another example of a positive climate feedback. As temperatures rise, sea ice retreats. The loss of ice exposes the underlying sea surface, which is darker and absorbs more sunlight than ice, increasing the total amount of warming. Less snow cover during warm winters has a similar effect. Clouds can have both warming and cooling effects on climate. They cool the planet by reflecting sunlight during the day, and they warm the planet by slowing the escape of heat to space (this is most apparent at night, as cloudy nights are usually warmer than clear nights). 8 Climate change can lead to changes in the coverage, altitude, and reflectivity of clouds. These changes can then either amplify (positive feedback) or dampen (negative feedback) the original change. The net effect of these changes is likely an amplifying, or positive, feedback due mainly to increasing altitude of high clouds in the tropics, which makes them better able to trap heat, and reductions in coverage of lower-level clouds in the mid-latitudes, which reduces the amount of sunlight they reflect. The magnitude of this feedback is uncertain due to the complex nature of cloud/climate interactions.8 9 Causes of climate change What is the most important cause of climate change? Human activity is the main cause of climate change. People burn fossil fuels and convert land from forests to agriculture. Since the beginning of the Industrial Revolution, people have burned more and more fossil fuels and changed vast areas of land from forests to farmland. Burning fossil fuels produces carbon dioxide, a greenhouse gas. It is called a greenhouse gas because it produces a “greenhouse effect”. The greenhouse effect makes the earth warmer, just as a greenhouse is warmer than its surroundings. Carbon dioxide is the main cause of human-induced climate change. It stays in the atmosphere for a very long time. Other greenhouse gases, such as nitrous oxide, stay in the atmosphere for a long time. Other substances only produce short-term effects. Not all substances produce warming. Some, like certain aerosols, can produce cooling. What are climate forcers? Carbon dioxide and other substances are referred to as climate forcers because they force or push the climate towards being warmer or cooler. They do this by affecting the flow of energy coming into and leaving the earth’s climate system. Small changes in the sun’s energy that reaches the earth can cause some climate change. But since the Industrial Revolution, adding greenhouse gases has been over 50 times more powerful than changes in the Sun's radiance. The additional greenhouse gases in earth’s atmosphere have had a strong warming effect on earth’s climate. Future emissions of greenhouse gases, particularly carbon dioxide, will determine how much more climate warming occurs. What can be done about climate change? Carbon dioxide is the main cause of human-induced global warming and associated climate change. It is a very long-lived gas, which means carbon dioxide builds up in the atmosphere with ongoing human emissions and remains in the atmosphere for centuries. Global warming can only be stopped by reducing global emissions of carbon dioxide from human fossil fuel combustion and industrial processes to zero, but even with zero emissions, the global temperature will remain 10 essentially constant at its new warmer level. Emissions of other substances that warm the climate must also be substantially reduced. This indicates how difficult the challenge is. What is climate change? Climate change is a long-term shift in weather conditions identified by changes in temperature, precipitation, winds, and other indicators. Climate change can involve both changes in average conditions and changes in variability, including, for example, extreme events. The earth's climate is naturally variable on all time scales. However, its long-term state and average temperature are regulated by the balance between incoming and outgoing energy, which determines the Earth's energy balance. Any factor that causes a sustained change to the amount of incoming energy or the amount of outgoing energy can lead to climate change. Different factors operate on different time scales, and not all of those factors that have been responsible for changes in earth's climate in the distant past are relevant to contemporary climate change. Factors that cause climate change can be divided into two categories - those related to natural processes and those related to human activity. In addition to natural causes of climate change, changes internal to the climate system, such as variations. In ocean currents or atmospheric circulation, can also influence the climate for short periods of time. This natural internal climate variability is superimposed on the long-term forced climate change. Does climate change have natural causes? The Earth's climate can be affected by natural factors that are external to the climate system, such as changes in volcanic activity, solar output, and the Earth's orbit around the Sun. Of these, the two factors relevant on timescales of contemporary climate change are changes in volcanic activity and changes in solar radiation. In terms of the Earth's energy balance, these factors primarily influence the amount of incoming energy. Volcanic eruptions are episodic and have relatively short-term effects on climate. Changes in solar irradiance have contributed to climate trends over the past century but since the Industrial Revolution, the effect of additions of greenhouse gases to the atmosphere has been over 50 times that of changes in the Sun's output. Human causes Climate change can also be caused by human activities, such as the burning of fossil fuels and the conversion of land for forestry and agriculture. Since the beginning of the Industrial Revolution, these human influences on the climate system have increased substantially. In addition to other environmental impacts, these activities change the land surface and emit various substances to the atmosphere. These in turn can influence both the amount of incoming energy and the amount of outgoing energy and can have both warming and cooling effects on the climate. The dominant product of fossil fuel combustion is carbon dioxide, a greenhouse gas. The overall effect of human activities since the Industrial Revolution has been a warming effect, 11 driven primarily by emissions of carbon dioxide and enhanced by emissions of other greenhouse gases. The build-up of greenhouse gases in the atmosphere has led to an enhancement of the natural greenhouse effect. It is this human-induced enhancement of the greenhouse effect that is of concern because ongoing emissions of greenhouse gases have the potential to warm the planet to levels that have never been experienced in the history of human civilization. Such climate change could have far-reaching and/or unpredictable environmental, social, and economic consequences. Short-lived and long-lived climate forcers Carbon dioxide is the main cause of human-induced climate change. It has been emitted in vast quantities from the burning of fossil fuels and it is a very long-lived gas, which means it continues to affect the climate system during its long residence time in the atmosphere. However, fossil fuel combustion, industrial processes, agriculture, and forestry-related activities emit other substances that also act as climate forcers. Some, such as nitrous oxide, are long-lived greenhouse gases like carbon dioxide, and so contribute to long-term climate change. Other substances have shorter atmospheric lifetimes because they are removed fairly quickly from the atmosphere. Therefore, their effect on the climate system is similarly short-lived. Together, these short-lived climate forcers are responsible for a significant amount of current climate forcing from anthropogenic substances. Some short-lived climate forcers have a climate warming effect (‘positive climate forcers') while others have a cooling effect (‘negative climate forcers'). If atmospheric levels of short-lived climate forcers are continually replenished by ongoing emissions, these continue to exert a climate forcing. However, reducing emissions will quite quickly lead to reduced atmospheric levels of such substances. A number of short-lived climate forcers have climate warming effects and together are the most important contributors to the human enhancement of the greenhouse effect after carbon dioxide. This includes methane and tropospheric ozone – both greenhouse gases – and black carbon, a small solid particle formed from the incomplete combustion of carbon-based fuels (coal, oil and wood for example). Other short-lived climate forcers have climate cooling effects, most notably sulphate aerosols. Fossil fuel combustion emits sulphur dioxide into the atmosphere (in addition to carbon dioxide) which then combines with water vapour to form tiny droplets (aerosols) which reflect sunlight. Sulphate aerosols remain in the atmosphere for only a few days (washing out in what is referred to as acid rain), and so do not have the same longterm effect as greenhouse gases. The cooling from sulphate aerosols in the atmosphere has, however, offset some of the warming from other substances. That is, the warming we have experienced to date would have been even larger had it not been for elevated levels of sulphate aerosols in the atmosphere. 12 13 14 15 16 17 18 19 20 21 22 23 24 25 26 27 28 29 Climate Change 2022: Impacts, Adaptation & Vulnerability - Full video (https://youtu.be/SDRxfuEvqGg) 30 Sector by sector: where do global greenhouse gas emissions come from? Globally, we emit around 50 billion tonnes of greenhouse gases each year. Where do these emissions come from? We take a look, sector-by-sector. by Hannah Ritchie September 18, 2020 31 To prevent severe climate change we need to rapidly reduce global greenhouse gas emissions. The world emits around 50 billion tonnes of greenhouse gases each year [measured in carbon dioxide equivalents (CO2eq)].1 To figure out how we can most effectively reduce emissions and what emissions can and can’t be eliminated with current technologies, we need to first understand where our emissions come from. In this post I present only one chart, but it is an important one – it shows the breakdown of global greenhouse gas emissions in 2016.2 This is the latest breakdown of global emissions by sector, published by Climate Watch and the World Resources Institute.3,4 The overall picture you see from this diagram is that almost three-quarters of emissions come from energy use; almost one-fifth from agriculture and land use [this increases to one-quarter when we consider the food system as a whole – including processing, packaging, transport and retail]; and the remaining 8% from industry and waste. To know what’s included in each sector category, I provide a short description of each. These descriptions are based on explanations provided in the IPCC’s Fifth Assessment Report AR5) and a methodology paper published by the World Resources Institute.5,6 Emissions come from many sectors: we need many solutions to decarbonize the economy It is clear from this breakdown that a range of sectors and processes contribute to global emissions. This means there is no single or simple solution to tackle climate change. Focusing on electricity, or transport, or food, or deforestation alone is insufficient. Even within the energy sector – which accounts for almost three-quarters of emissions – there is no simple fix. Even if we could fully decarbonize our electricity supply, we would also need to electrify all of our heating and road transport. And we’d still have emissions from shipping and aviation – which we do not yet have low-carbon technologies for – to deal with. To reach net-zero emissions we need innovations across many sectors. Single solutions will not get us there. Let’s walk through each of the sectors and sub-sectors in the pie chart, one-by-one. 32 Energy (electricity, heat and transport): 73.2% Energy use in industry: 24.2% Iron and Steel (7.2%): energy-related emissions from the manufacturing of iron and steel. Chemical & petrochemical (3.6%): energy-related emissions from the manufacturing of fertilizers, pharmaceuticals, refrigerants, oil and gas extraction, etc. Food and tobacco (1%): energy-related emissions from the manufacturing of tobacco products and food processing (the conversion of raw agricultural products into their final products, such as the conversion of wheat into bread). Non-ferrous metals: 0.7%: Non-ferrous metals are metals which contain very little iron: this includes aluminium, copper, lead, nickel, tin, titanium and zinc, and alloys such as brass. The manufacturing of these metals requires energy which results in emissions. Paper & pulp (0.6%): energy-related emissions from the conversion of wood into paper and pulp. Machinery (0.5%): energy-related emissions from the production of machinery. Other industry (10.6%): energy-related emissions from manufacturing in other industries including mining and quarrying, construction, textiles, wood products, and transport equipment (such as car manufacturing). Transport: 16.2% This includes a small amount of electricity (indirect emissions) as well as all direct emissions from burning fossil fuels to power transport activities. These figures do not include emissions from the manufacturing of motor vehicles or other transport equipment – this is included in the previous point ‘Energy use in Industry’. Road transport (11.9%): emissions from the burning of petrol and diesel from all forms of road transport which includes cars, trucks, lorries, motorcycles and buses. Sixty percent of road transport emissions come from passenger travel (cars, motorcycles and buses); and the remaining forty percent from road freight (lorries and trucks). This means that, if we could electrify the whole road transport sector, and transition to a fully decarbonized electricity mix, we could feasibly reduce global emissions by 11.9%. Aviation (1.9%): emissions from passenger travel and freight, and domestic and international aviation. 81% of aviation emissions come from passenger travel; and 19% from freight.7 From passenger aviation, 60% of emissions come from international travel, and 40% from domestic. Shipping (1.7%): emissions from the burning of petrol or diesel on boats. This includes both passenger and freight maritime trips. 33 Rail (0.4%): emissions from passenger and freight rail travel. Pipeline (0.3%): fuels and commodities (e.g. oil, gas, water or steam) often need to be transported (either within or between countries) via pipelines. This requires energy inputs, which results in emissions. Poorly constructed pipelines can also leak, leading to direct emissions of methane to the atmosphere – however, this aspect is captured in the category ‘Fugitive emissions from energy production’. Energy use in buildings: 17.5% Residential buildings (10.9%): energy-related emissions from the generation of electricity for lighting, appliances, cooking etc. and heating at home. Commercial buildings (6.6%): energy-related emissions from the generation of electricity for lighting, appliances, etc. and heating in commercial buildings such as offices, restaurants, and shops. Unallocated fuel combustion (7.8%) Energy-related emissions from the production of energy from other fuels including electricity and heat from biomass; on-site heat sources; combined heat and power (CHP); nuclear industry; and pumped hydroelectric storage. Fugitive emissions from energy production: 5.8% Fugitive emissions from oil and gas (3.9%): fugitive emissions are the often-accidental leakage of methane to the atmosphere during oil and gas extraction and transportation, from damaged or poorly maintained pipes. This also includes flaring – the intentional burning of gas at oil facilities. Oil wells can release gases, including methane, during extraction – producers often don’t have an existing network of pipelines to transport it, or it wouldn’t make economic sense to provide the infrastructure needed to effectively capture and transport it. But under environmental regulations they need to deal with it somehow: intentionally burning it is often a cheap way to do so. Fugitive emissions from coal (1.9%): fugitive emissions are the accidental leakage of methane during coal mining. 34 Energy use in agriculture and fishing (1.7%) Energy-related emissions from the use of machinery in agriculture and fishing, such as fuel for farm machinery and fishing vessels. Direct Industrial Processes: 5.2% Cement (3%): carbon dioxide is produced as a byproduct of a chemical conversion process used in the production of clinker, a component of cement. In this reaction, limestone (CaCO3) is converted to lime (CaO), and produces CO2 as a byproduct. Cement production also produces emissions from energy inputs – these related emissions are included in ‘Energy Use in Industry’. Chemicals & petrochemicals (2.2%): greenhouse gases can be produced as a byproduct from chemical processes – for example, CO2 can be emitted during the production of ammonia, which is used for purifying water supplies, cleaning products, and as a refrigerant, and used in the production of many materials, including plastic, fertilizers, pesticides, and textiles. Chemical and petrochemical manufacturing also produces emissions from energy inputs – these related emissions are included in ‘Energy Use in Industry’. Waste: 3.2% Wastewater (1.3%): organic matter and residues from animals, plants, humans and their waste products can collect in wastewater systems. When this organic matter decomposes it produces methane and nitrous oxide. Landfills (1.9%): landfills are often low-oxygen environments. In these environments, organic matter is converted to methane when it decomposes. Agriculture, Forestry and Land Use: 18.4% Agriculture, Forestry and Land Use directly accounts for 18.4% of greenhouse gas emissions. The food system as a whole – including refrigeration, food processing, packaging, and transport – accounts for around one-quarter of greenhouse gas emissions. We look at this in detail here. Grassland (0.1%): when grassland becomes degraded, these soils can lose carbon, converting to carbon dioxide in the process. Conversely, when grassland is restored (for example, from 35 cropland), carbon can be sequestered. Emissions here therefore refer to the net balance of these carbon losses and gains from grassland biomass and soils. Cropland (1.4%): depending on the management practices used on croplands, carbon can be lost or sequestered into soils and biomass. This affects the balance of carbon dioxide emissions: CO2 can be emitted when croplands are degraded; or sequestered when they are restored. The net change in carbon stocks is captured in emissions of carbon dioxide. This does not include grazing lands for livestock. Deforestation (2.2%): net emissions of carbon dioxide from changes in forestry cover. This means reforestation is counted as ‘negative emissions’ and deforestation as ‘positive emissions’. Net forestry change is therefore the difference between forestry loss and gain. Emissions are based on lost carbon stores from forests and changes in carbon stores in forest soils. Crop burning (3.5%): the burning of agricultural residues – leftover vegetation from crops such as rice, wheat, sugar cane, and other crops – releases carbon dioxide, nitrous oxide and methane. Farmers often burn crop residues after harvest to prepare land for the resowing of crops. Rice cultivation (1.3%): flooded paddy fields produce methane through a process called ‘anaerobic digestion’. Organic matter in the soil is converted to methane due to the low-oxygen environment of waterlogged rice fields. 1.3% seems substantial, but it’s important to put this into context: rice accounts for around one-fifth of the world’s supply of calories, and is a staple crop for billions of people globally.8 Agricultural soils (4.1%): Nitrous oxide – a strong greenhouse gas – is produced when synthetic nitrogen fertilizers are applied to soils. This includes emissions from agricultural soils for all agricultural products – including food for direct human consumption, animal feed, biofuels and other non-food crops (such as tobacco and cotton). Livestock & manure (5.8%): animals (mainly ruminants, such as cattle and sheep) produce greenhouse gases through a process called ‘enteric fermentation’ – when microbes in their digestive systems break down food, they produce methane as a by-product. This means beef and lamb tend to have a high carbon footprint, and eating less is an effective way to reduce the emissions of your diet. Nitrous oxide and methane can be produced from the decomposition of animal manures under low oxygen conditions. This often occurs when large numbers of animals are managed in a confined area (such as dairy farms, beef feedlots, and swine and poultry farms), where manure is typically stored in large piles or disposed of in lagoons and other types of manure management systems ‘Livestock’ emissions here include direct emissions from livestock onlythey do not consider impacts of land use change for pasture or animal feed. 36 Energy and the Environment Explained BASICS Natural gas has many qualities that make it an efficient, relatively clean burning, and economical energy source. However, the production and use of natural gas have some environmental and safety issues to consider. Natural gas is a relatively clean burning fossil fuel Burning natural gas for energy results in fewer emissions of nearly all types of air pollutants and carbon dioxide (CO2) than burning coal or petroleum products to produce an equal amount of energy. About 117 pounds of CO2 are produced per million British thermal units (MMBtu) equivalent of natural gas compared with more than 200 pounds of CO2 per MMBtu of coal and more than 160 pounds per MMBtu of distillate fuel oil. The clean burning properties of natural gas have contributed to increased natural gas use for electricity generation and as a transportation fuel for fleet vehicles in the United States. Natural gas is mainly methane—a strong greenhouse gas Some natural gas leaks into the atmosphere from oil and natural gas wells, storage tanks, pipelines, and processing plants. The U.S. Environmental Protection Agency estimates that in 2019, methane emissions from natural gas and petroleum systems and from abandoned oil and natural gas wells were the source of about 29% of total U.S. methane emissions and about 3% of total U.S. greenhouse gas emissions.1 The oil and natural gas industry takes steps to prevent natural gas leaks. Natural gas exploration, drilling, and production affects the environment When geologists explore for natural gas deposits on land, they may disturb vegetation and soil with their vehicles. Drilling a natural gas well on land may require clearing and leveling an area around the well site. Well drilling activities produce air pollution and may disturb people, wildlife, and water resources. Laying pipelines that transport natural gas from wells usually requires 37 clearing land to bury the pipe. Natural gas production can also produce large volumes of contaminated water. This water requires proper handling, storage, and treatment so that it does not pollute land and other waters. Natural gas wells and pipelines often have engines to run equipment and compressors, which produce air pollutants and noise. In areas where natural gas is produced at oil wells but is not economical to transport for sale or contains high concentrations of hydrogen sulfide (a toxic gas), it is burned (flared) at well sites. Natural gas flaring produces CO2, carbon monoxide, sulfur dioxide, nitrogen oxides, and many other compounds, depending on the chemical composition of the natural gas and on how well the natural gas burns in the flare. However, flaring is safer than releasing natural gas into the air and results in lower overall greenhouse gas emissions because CO2 is not as strong a greenhouse gas as methane. BASICS Biofuels may have fewer effects on the environment than fossil fuels Production and use of biofuels is considered by the U.S. government to have fewer or lower negative effects on the environment compared to fossil-fuel derived fuels. There are also potential national economic and security benefits when biofuel use reduces the need to import petroleum fuels. Government programs that promote and/or require biofuels use, such as the U.S. Renewable Fuel Standard (RFS) and California's Low Carbon Fuel Standard (LCFS), define the types of biofuels and processes or low-carbon pathways by which biofuels can be produced in order for them to qualify for use under the programs. While biofuels have environmental benefits, their production and use do have effects on the environment. Pure ethanol and biodiesel are nontoxic and biodegradable, and if spilled, they break down into harmless substances. However, fuel ethanol contains denaturants to make fuel ethanol undrinkable. Similar to petroleum fuels, biofuels are flammable (especially ethanol) and must be transported carefully. When burned, pure biofuels generally produce fewer emissions of particulates, sulfur dioxide, and air toxics than their fossil-fuel derived counterparts. Biofuel-petroleum blends also generally 38 result in lower emissions relative to fuels that do not contain biofuels. Biodiesel combustion may result in slightly higher amounts of nitrogen oxides relative to petroleum diesel. Ethanol and ethanol-gasoline mixtures burn cleaner and have higher octane levels than gasoline that does not contain ethanol, but they also have higher evaporative emissions from fuel tanks and dispensing equipment. These evaporative emissions contribute to the formation of harmful, ground-level ozone and smog. Gasoline requires extra processing to reduce evaporative emissions before blending with ethanol. Burning biofuels results in emissions of carbon dioxide (CO2), a greenhouse gas. However, according to international convention, CO2 emissions from biofuel combustion are excluded from national greenhouse gas emissions inventories because growing the biomass feedstocks used for biofuel production may offset the CO2 produced when biofuels are burned. The effect that biofuel use has on net CO2 emissions depends on how the biofuels are produced and whether or not emissions associated with cropland cultivation are included in the calculations. Growing plants for fuel is a controversial topic because some people believe the land, fertilizers, and energy used to grow biofuel crops should be used to grow food crops instead. In some parts of the world, large areas of natural vegetation and forests have been cleared or burned to grow soybeans and palm oil trees to make biodiesel. The processes for producing ethanol, renewable diesel, renewable heating oil, and renewable aviation fuel require a heat source, and most producers of these biofuels currently use fossil fuels. Some U.S. ethanol producers burn corn stalks for heat and ethanol producers in Brazil use sugar cane stalks (called bagasse) to produce heat and electricity. The U.S. government is supporting efforts to produce biofuels with methods that use less energy than conventional fermentation and that use cellulosic biomass, which requires less cultivation, fertilizer, and pesticides than corn or sugar cane. Cellulosic ethanol feedstock includes native prairie grasses, fast-growing trees, sawdust, and even waste paper. However, there is currently no commercial cellulosic ethanol production in the United States because of technical and economic challenges. Lipid feedstocks—waste/used cooking oil and animal fats/tallow and grease—have relatively low carbon intensities as feedstocks for biofuels production and they have been used to meet the targets for advanced biofuels under the federal RFS program. The total process (or lifecycle) emissions for lipid feedstocks are low because lipids were previously used for another purpose and the emissions related to transportation of these biofuels feedstocks only account for emissions that occur after the waste oil/grease is collected. Because of their potentially lower carbon intensities, some state governments provide more support for biofuels production from lipid feedstocks than for raw, unused vegetable oil feedstocks. In California, lipids account for the majority of the feedstocks for U.S. non-fuel ethanol biofuels production and also for the majority of credits generated under California's LCFS. The federal RFS currently does not differentiate between lipid and vegetable oil feedstocks as it does with cellulosic and other renewable fuels. At scale, hydrogenated lipid-based biofuels production requires a significant 39 amount of hydrogen, which if produced from fossil fuels, may increase process emissions and thus increase their carbon intensity. Last updated: April 13, 2022 BASICS Using biomass for energy has positive and negative effects Biomass and biofuels made from biomass are alternative energy sources to fossil fuels—coal, petroleum, and natural gas. Burning either fossil fuels or biomass releases carbon dioxide (CO2), a greenhouse gas. However, the plants that are the source of biomass for energy capture almost the same amount of CO2 through photosynthesis while growing as is released when biomass is burned, which can make biomass a carbon-neutral energy source.1 Burning wood Using wood, wood pellets, and charcoal for heating and cooking can replace fossil fuels and may result in lower CO2 emissions overall. Wood can be harvested from forests, from woodlots that have to be thinned, or from urban trees that fall down or have to be cut down. Wood smoke contains harmful pollutants such as carbon monoxide and particulate matter. Modern wood-burning stoves, pellet stoves, and fireplace inserts can reduce the amount of particulates from burning wood. Wood and charcoal are major cooking and heating fuels in poor countries, but if people harvest the wood faster than trees can grow, it causes deforestation. Planting fastgrowing trees for fuel and using fuel-efficient cooking stoves can help slow deforestation and improve the environment. Burning municipal solid waste (MSW) or wood waste Burning municipal solid waste (MSW), or garbage, in waste-to-energy plants could result in less waste buried in landfills. On the other hand, burning garbage produces air pollution and releases the chemicals and substances in the waste into the air. Some of these chemicals, which are mostly related to the combustion of non-biomass materials in garbage, can be hazardous to people and the environment if they are not properly controlled. 40 The U.S. Environmental Protection Agency (EPA) applies strict environmental rules to waste-toenergy plants , which require waste-to-energy plants to use air pollution control devices such as scrubbers, fabric filters, and electrostatic precipitators to capture air pollutants. Scrubbers clean emissions from waste-to-energy facilities by spraying a liquid into the combustion gases to neutralize the acids present in the stream of emissions. Fabric filters and electrostatic precipitators also remove particles from the combustion gases. The particles—called fly ash—are then mixed with the ash that is removed from the bottom of the waste-to-energy furnace. A waste-to-energy furnace burns at high temperatures (1,800°F to 2,000°F), which break down the chemicals in MSW into simpler, less harmful compounds. Disposing ash from waste-to-energy plants Ash from waste-to-energy plants can contain high concentrations of various metals that were present in the original waste. Textile dyes, printing inks, and ceramics, for example, may contain lead and cadmium. Separating waste before burning can solve part of the problem. Because batteries are the largest source of lead and cadmium in municipal waste, they should not be included in regular trash. Florescent light bulbs should also not be put in regular trash because they contain small amounts of mercury. The EPA tests ash from waste-to-energy plants to make sure that it is not hazardous. The test looks for chemicals and metals that could contaminate ground water. Some MSW landfills use ash that is considered safe as a cover layer for their landfills, and some MSW ash is used to make concrete blocks and bricks. Collecting landfill gas or biogas Biogas forms as a result of biological processes in sewage treatment plants, waste landfills, and livestock manure management systems. Biogas is composed mainly of methane (a greenhouse gas) and CO2. Many facilities that produce biogas capture it and burn the methane for heat or to generate electricity. This electricity is considered renewable and, in many states, contributes to meeting state renewable portfolio standards (RPS). This electricity may replace electricity generation from fossil fuels and can result in a net reduction in CO2 emissions. Burning methane produces CO2, but because methane is a stronger greenhouse gas than CO2, the overall greenhouse effect is lower. Biofuels Biofuels are generally cleaner burning than petroleum fuels made from crude oil, but production and use of biofuels do have effects on the environment. Biofuels may be considered carbon41 neutral because the plants that are used to make biofuels (such as corn and sugarcane for ethanol and soy beans and oil palm trees for biodiesel) absorb CO2 as they grow and may offset the CO2 emissions when biofuels are produced and burned. BASICS Although electricity is a clean and relatively safe form of energy when it is used, the generation and transmission of electricity affects the environment. Nearly all types of electric power plants have an effect on the environment, but some power plants have larger effects than others. The United States has laws that govern the effects that electricity generation and transmission have on the environment. The Clean Air Act regulates air pollutant emissions from most power plants. The U.S. Environmental Protection Agency (EPA) administers the Clean Air Act and sets emissions standards for power plants through various programs such as the Acid Rain Program. The Clean Air Act has helped to substantially reduce emissions of some major air pollutants in the United States. The effect of power plants on the landscape All power plants have a physical footprint (the location of the power plant). Some power plants are located inside, on, or next to an existing building, so the footprint is fairly small. Most large power plants require land clearing to build the power plant. Some power plants may also require access roads, railroads, and pipelines for fuel delivery, electricity transmission lines, and cooling water supplies. Power plants that burn solid fuels may have areas to store the combustion ash. Many power plants are large structures that alter the visual landscape. In general, the larger the structure, the more likely it is that the power plant will affect the visual landscape. Fossil fuel, biomass, and waste burning power plants In the United States, about 60% of total electricity generation in 2020 was produced from fossil fuels (coal, natural gas, and petroleum), materials that come from plants (biomass), and municipal and industrial wastes. The substances that occur in combustion gases when these fuels are burned include: Carbon dioxide (CO2) Carbon monoxide (CO) 42 Sulfur dioxide (SO2) Nitrogen oxides (NOx) Particulate matter (PM) Heavy metals such as mercury Nearly all combustion byproducts have negative effects on the environment and human health: CO2 is a greenhouse gas, which contributes to the greenhouse effect. SO2 causes acid rain, which is harmful to plants and to animals that live in water. SO 2 also worsens respiratory illnesses and heart diseases, particularly in children and the elderly. NOx contribute to ground-level ozone, which irritates and damages the lungs. PM results in hazy conditions in cites and scenic areas and coupled with ozone, contributes to asthma and chronic bronchitis, especially in children and the elderly. Very small, or fine PM, is also believed to cause emphysema and lung cancer. Heavy metals such as mercury are hazardous to human and animal health. Power plants reduce air pollution emissions in various ways Air pollution emission standards limit the amounts of some of the substances that power plants can release into the air. Some of the ways that power plants meet these standards include: Burning low-sulfur-content coal to reduce SO2 emissions. Some coal-fired power plants cofire wood chips with coal to reduce SO2 emissions. Pretreating and processing coal can also reduce the level of undesirable compounds in combustion gases. Different kinds of particulate emission control devices treat combustion gases before they exit the power plant: o Bag-houses are large filters that trap particulates. o Electrostatic precipitators use electrically charged plates that attract and pull particulates out of the combustion gas. o Wet scrubbers use a liquid solution to remove PM from combustion gas. Wet and dry scrubbers mix lime in the fuel (coal) or spray a lime solution into combustion gases to reduce SO2 emissions. Fluidized bed combustion also results in lower SO2 emissions. NOx emissions controls include low NOx burners during the combustion phase or selective catalytic and non-catalytic converters during the post combustion phase. Many U.S. power plants produce CO2 emissions The electric power sector is a large source of U.S. CO2 emissions. Electric power sector power plants that burned fossil fuels or materials made from fossil fuels, and some geothermal power plants, were the source of about 28% of total U.S. energy-related CO2 emissions in 2020. 43 Some power plants also produce liquid and solid wastes Ash is the solid residue that results from burning solid fuels such as coal, biomass, and municipal solid waste. Bottom ash includes the largest particles that collect at the bottom of the combustion chamber of power plant boilers. Fly ash is the smaller and lighter particulates that collect in air emission control devices. Fly ash is usually mixed with bottom ash. The ash contains all the hazardous materials that pollution control devices capture. Many coal-fired power plants store ash sludge (ash mixed with water) in retention ponds. Several of these ponds have burst and caused extensive damage and pollution downstream. Some coal-fired power plants send ash to landfills or sell ash for use in making concrete blocks or asphalt. Nuclear power plants produce different kinds of waste Nuclear power plants do not produce greenhouse gases or PM, SO2, or NOx, but they do produce two general types of radioactive waste: Low-level waste, such as contaminated protective shoe covers, clothing, wiping rags, mops, filters, reactor water treatment residues, equipment, and tools, is stored at nuclear power plants until the radioactivity in the waste decays to a level safe for disposal as ordinary trash, or it is sent to a low-level radioactive waste disposal site. High-level waste, which includes the highly radioactive spent (used) nuclear fuel assemblies, must be stored in specially designed storage containers and facilities (see Interim storage and final disposal in the United States). BASICS The environmental effects of geothermal energy depend on how geothermal energy is used or how it is converted to useful energy. Direct use applications and geothermal heat pumps have almost no negative effects on the environment. In fact, they can have a positive effect by reducing the use of energy sources that may have negative effects on the environment. 44 Geothermal power plants have low emission levels Geothermal power plants do not burn fuel to generate electricity, but they may release small amounts of sulfur dioxide and carbon dioxide. Geothermal power plants emit 97% less acid raincausing sulfur compounds and about 99% less carbon dioxide than fossil fuel power plants of similar size. Geothermal power plants use scrubbers to remove the hydrogen sulfide naturally found in geothermal reservoirs. Most geothermal power plants inject the geothermal steam and water that they use back into the earth. This recycling helps to renew the geothermal resource and to reduce emissions from the geothermal power plants. BASICS Hydropower generators produce clean electricity, but hydropower does affect the environment Most dams in the United States were built mainly for flood control, municipal water supply, and irrigation water. Although many of these dams have hydroelectric generators, only a small number of dams were built specifically for hydropower generation. Hydropower generators do not directly emit air pollutants. However, dams, reservoirs, and the operation of hydroelectric generators can affect the environment. A dam that creates a reservoir (or a dam that diverts water to a run-of-river hydropower plant) may obstruct fish migration. A dam and reservoir can also change natural water temperatures, water chemistry, river flow characteristics, and silt loads. All of these changes can affect the ecology and the physical characteristics of the river. These changes may have negative effects on native plants and on animals in and around the river. Reservoirs may cover important natural areas, agricultural land, or archeological sites. A reservoir and the operation of the dam may also result in the relocation of people. The physical impacts of a dam and reservoir, the operation of the dam, and the use of the water can change the environment over a much larger area than the area a reservoir covers. Manufacturing the concrete and steel in hydropower dams requires equipment that may produce emissions. If fossil fuels are the energy sources for making these materials, then the emissions from the equipment could be associated with the electricity that hydropower facilities 45 generate. However, given the long operating lifetime of a hydropower plant (50 years to 100 years) these emissions are offset by the emissions-free hydroelectricity. Greenhouse gases (GHG) such as carbon dioxide and methane form in natural aquatic systems and in human-made water storage reservoirs as a result of the aerobic and anaerobic decomposition of biomass in the water. The exact amounts of GHG that form in and are emitted from hydropower reservoirs is uncertain and depend on many site specific and regional factors. Solar energy technologies and power plants do not produce air pollution or greenhouse gases when operating. Using solar energy can have a positive, indirect effect on the environment when solar energy replaces or reduces the use of other energy sources that have larger effects on the environment. However, there are environmental issues related to the production and use of solar energy technologies. Solar energy technologies require use of materials, such as metals and glass, that are energy intensive to make. The environmental issues related to the production of these materials could be associated with solar energy systems when conducting life-cycle or so called cradle-to-grave environmental analysis. Studies conducted by a number of organizations and researchers have concluded that PV systems can produce the equivalent amount of energy that was used to manufacture the systems within 1 to 4 years. Most PV systems have operating lives of up to 30 years or more. There are hazardous chemicals used to make photovoltaic (PV) cells and panels that must be carefully handled to avoid release to the environment. Some types of PV cell technologies use heavy metals, and these types of cells and PV panels may require special handling when they reach the end of their useful life. Some solar thermal systems use potentially hazardous fluids to transfer heat, and leaks of these materials could be harmful to the environment. U.S. environmental laws regulate the use and disposal of hazardous materials. The U.S. Department of Energy is supporting various efforts to address end-of-life issues related to solar energy technologies, including the recovery and recycling of the materials used to manufacture PV cells and panels. Several states have enacted laws that encourage recycling of PV panels. As with any type of power plant, large solar power plants can affect the environment at or near their locations. Clearing land for construction and the placement of the power plant may have long-term effects on the habitats of native plants and animals. However, installing solar energy 46 systems on land with marginal agricultural value or integrating solar energy systems on farms may provide a variety of economic and environmental benefits to farmers. Some solar power plants may require water for cleaning solar collectors and concentrators or for cooling turbine generators. Using large volumes of ground water or surface water for cleaning collectors in some arid locations may affect the ecosystems that depend on these water resources. In addition, the beam of concentrated sunlight a solar power tower creates can kill birds and insects that fly into the beam. BASICS Wind is an emissions-free source of energy Wind is a renewable energy source. Overall, using wind to produce energy has fewer effects on the environment than many other energy sources. Wind turbines do not release emissions that can pollute the air or water (with rare exceptions), and they do not require water for cooling. Wind turbines may also reduce the amount of electricity generation from fossil fuels, which results in lower total air pollution and carbon dioxide emissions. An individual wind turbine has a relatively small physical footprint. Groups of wind turbines, sometimes called wind farms, are located on open land, on mountain ridges, or offshore in lakes or the ocean. Wind turbines have some negative effects on the environment Modern wind turbines can be very large machines, and they may visually affect the landscape. A small number of wind turbines have also caught fire, and some have leaked lubricating fluids, but these occurrences are rare. Some people do not like the sound that wind turbine blades make as they turn in the wind. Some types of wind turbines and wind projects cause bird and bat deaths. These deaths may contribute to declines in the population of species also affected by other human-related impacts. The wind energy industry and the U.S. government are researching ways to reduce the effect of wind turbines on birds and bats. Most wind power projects on land require service roads that add to the physical effects on the environment. Producing the metals and other materials used to make wind turbine components 47 has impacts on the environment, and fossil fuels may have been used to produce the materials. Although most of the materials used to make wind turbines can be reused or recycled, turbine blades, as most are currently constructed, cannot be recycled. Researchers at the National Renewable Energy Laboratory (NREL) established an approach to manufacturing wind turbine blades, employing a thermoplastic resin system. These thermoplastic resins enable a manufacturing process that allows wind turbine blades to be recycled at their end of life and also reduces the energy required to manufacture blades. Last updated: December 17, 2021 48 Key Topic 2: Measuring and Monitoring a Changing Climate 6. Describe the history of international collaboration on climate change and analyze the successes and shortcomings. 7. Describe the various sources of scientific data which are used as evidence of climate change and explain how we know this data to be reliable. 8. Evaluate climate data and draw conclusions based on that data. 9. Explain the use of modelling in forecasting climate and the sources of uncertainty in climate projections. Study Resources Resource Title Source Located on A Short History of International Climate Change Negotiations – from Rio to Glasgow Mark Maslin, University College London, 2021 Pages 50-56 Climate Models US National Oceanic and Atmospheric Administration (NOAA), 2022 Pages 57-59 Climate Change Projections Government of Canada, Canadian Centre for Climate Services, 2021 Pages 60-61 *Please Note: Hyperlinks found in text are not considered required reading; however, included video links are required to watch.* Study Resources begin on the next page 49 A Short History of International Climate Change Negotiations – from Rio to Glasgow 25 January 2021 Despite decades of intense and continuous international negotiations on climate change, progress has been slow. Professor Mark Maslin reflects on the history of negotiations and why there is now hope that states will substantially cut down greenhouse gas emissions. The last 30 years have been a period of intense and continuous international negotiation to deal with climate change. During the same 30 years, humanity has doubled the amount of anthropogenic carbon dioxide in the atmosphere. In 1989 Margaret Thatcher, the Prime Minster of the UK, gave an address to the UN outlining the science of climate change, the threat it posed to all nations, and the actions needed to avert the crisis. She summed up by saying: “We should work through this great organisation and its agencies to secure world-wide agreements on ways to cope with the effects of climate change, the thinning of the ozone layer, and the loss of precious species” (Margaret Thatcher Foundation, 2020). This sentiment was echoed in similar speeches by George Bush Senior, President of the United States, including one in 1992 when he outlined his ‘Clear Skies’ and ‘Global Climate Change’ initiatives at the National Oceanic and Atmospheric Administration. This was because by the end of the 1980s the threat of climate change had finally been recognized. This was due to the global temperature record ‘hockey stick’ upturn at the end of the 1980s (Maslin, 2021). This led to the rediscovery of the underpinning science of climate change that had been essentially carried out and settled by the mid-1960s (Weart, 2008). This was combined with our increased knowledge of how past climate was controlled by changes in atmospheric CO2 and significant improvements in supercomputer modelling of our climate system (Maslin, 2021). There was also the emergence of global environmental awareness in the late 1980s driven by a series of catastrophic local pollution events and the discovery of the ozone hole over Antarctica (Corfee-Morlot et al., 2007). By the beginning of the 1990s climate change had become a global issue - even if it was still a highly disputed one (Oreskes and Conway, 2012). The Intergovernmental Panel on Climate Change (IPCC) was set up in 1988 and produced its very first science report in 1990. Two years later, with support from leaders from all around the world, the UN held the Rio Earth Summit - officially called the United Nations Conference on Environment and Development (UNCED) - to help member states cooperate on sustainability and protecting the world’s environment. The Summit was a huge success and led to the Rio Declaration on Environment and Development, the local sustainability initiative called Agenda 21 and Forest Principles (Gupta, 2014). It also set up the United Nations Convention to Combat Desertification, the Convention on Biological Diversity and the Framework Convention on Climate Change (UNFCCC) that underlies the negotiations to limit global greenhouse gas 50 emissions. The Rio Earth Summit also laid the foundations for the Millennium Development Goals and the subsequent Sustainable Development Goals. The United Nations Framework Convention on Climate Change (UNFCCC) officially came into force on 21 March 1994. As of March 2020, the UNFCCC has 196 parties. Enshrined within the UNFCCC are a number of principles including agreement by consensus of all parties and differential responsibilities (Gupta, 2014). The latter is because the UNFCCC acknowledges that different countries have emitted different amounts of greenhouse gases (GHGs) and therefore need to make greater or lesser efforts to reduce their emissions. For example, per capita emissions of CO2 in the USA are ten times greater than in India. The UNFCCC pays heed to the principle of contraction and convergence - the idea that every country must reduce its emissions and that all countries must converge on net zero emissions. The net zero emissions target emerged from the important IPCC 1.5˚C global warming report published in 2018 which clearly showed that to achieve 1.5˚C there had to be zero carbon emissions by about 2050 and then negative carbon emissions for the rest of the century (IPCC, 2018). Kyoto 1997 Since the UNFCCC was set up, the nations of the world, ‘the parties’, have been meeting annually at the ‘Conference of the Parties’ (COP) to move negotiations forward. Only five years after the UNFCCC was created, at COP3 in December 1997, the first international agreement was drawn up, the Kyoto Protocol (Gupta, 2014). This stated the general principles for a worldwide treaty on cutting GHG emissions and, more specifically, that all developed nations would aim to cut their emissions by 5.2% relative to their 1990 levels by 2008-12. The Kyoto Protocol was ratified and signed in Bonn on 23 July 2001, making it a legal treaty. The USA, under the leadership of President Bush, withdrew from the climate negotiations in March 2001 and so did not sign the Kyoto Protocol at the Bonn meeting. With the USA producing about onequarter of the world’s carbon dioxide pollution at this time, this was a big blow for the treaty. Moreover, the targets set by the Kyoto Protocol were reduced during the Bonn meeting to make sure that Japan, Canada, and Australia would join. Australia finally made the Kyoto Protocol legally binding in December 2007. In order to balance out the historic legacy of emissions by developed countries, the treaty did not include developing countries, but it was assumed that developing countries would join the post-2012 agreement. The Kyoto Protocol came into force in February 2005, after Russia ratified the treaty, thereby meeting the requirement that at least 55 countries representing more than 55 per cent of the global emissions were participating (Gupta, 2014). Copenhagen 2009 There were huge expectations of COP15 (Copenhagen) in 2009 despite coming a year after the global financial crash. New quantitative commitments were expected to ensure a post-2012 agreement in order to move seamlessly on from the Kyoto Protocol. Barack Obama had just become President of the USA, raising hopes of a more positive approach. The EU had prepared an unconditional 20% reduction of emissions by 2020 on a 1990 baseline and a conditional target rising to 30% if other developed countries adopted binding targets. Most other developed 51 countries had something to offer. Norway was willing to reduce emissions by 40% and Japan by 25% from a 1990 baseline. Even the USA offered a 17% reduction on a 2005 baseline, which was an equivalent drop of 4% on a 1990 baseline. But the Copenhagen conference went horribly wrong. First the Danish government had completely underestimated the interest in the conference and provided a venue that was too small. So in the second week, when all the highpowered country ministers and their support arrived, there was not enough room, meaning that many NGOs were denied access to the negotiations. Second, it was clear that the negotiators were not ready for the arrival of the ministers and that there was no agreement. This led to the leaking of ‘The Danish Text’, subtitled ‘The Copenhagen Agreement’, and the proposed measures to keep average global temperature rise to 2°C above pre-industrial levels (Gupta, 2014). It started an argument between developed and developing nations as it was brand new text that had just appeared in the middle of the conference. Developing countries accused the developed countries of working behind closed doors and making an agreement that suited them without seeking consent from the developing nations (Byrne and Maslin, 2015). Lumumba Stanislaus Di-Aping, chairman of the G77, said, ‘it’s an incredibly imbalanced text intended to subvert, absolutely and completely, two years of negotiations. It does not recognize the proposals and the voice of developing countries’ (Guardian, 2009). The final blow to getting an agreement on binding targets came from the USA. Barack Obama, arriving only two days before the end of the conference, convened a meeting of the USA with the BASIC (Brazil, South Africa, India, and China) countries which excluded other UN nations, and created the Copenhagen Accord (Maslin, 2021). This recognized the scientific case for keeping temperature rises below 2°C, but did not contain a baseline for this target, nor commitments for reduced emissions that would be necessary to achieve it. Earlier proposals that would have aimed to limit temperature rises to 1.5°C and cut CO2 emissions by 80 per cent by 2050 were dropped. The agreement made was non-binding and countries had until January 2010 to provide their own voluntary targets. It was also made clear that any country that signed up to the Copenhagen Accord was also stepping out of the Kyoto Protocol. Hence the USA was able to move away from the binding targets of Kyoto Protocol, which should have been enforced until 2012, and a weak voluntary commitment approach was adopted. The Bolivian delegation summed up the way the Copenhagen Accord was reached - ‘anti-democratic, anti-transparent and unacceptable’ (Guardian, 2009). It was also not clear what legal status the Copenhagen Accord had as it was only ‘noted’ by the parties, not agreed, as only 122, subsequently rising to 139 countries, agreed to it (Bryne and Maslin, 2015). Trust in the UNFCCC negotiations took another blow when in January 2014 it was revealed that the US Government negotiators had information during the conference obtained by eavesdropping on meetings of other conference delegations. Documents leaked by Edward Snowden showed how the US National Security Agency (NSA) had monitored communications between countries before and during the conference. The leaked documents show that the NSA provided US delegates with advance details of the Danish plan to ‘rescue’ the talks should they founder, and also about China’s efforts before the conference to coordinate its position with that of India (Guardian, 2014). 52 Paris 2015 The failure of COP15 in Copenhagen and its voluntary commitments cast a long shadow over the successive COP meetings, compounded by the revelation by Wikileaks that US aid funding to Bolivia and Ecuador was reduced because of their opposition to the Copenhagen Accord Guardian (2010). It took over five years for the negotiations to recover from the mess created by Barack Obama and the USA negotiators. At COP16 in Cancun and COP17 in Durban the UNFCCC negotiations were slowly put back on track with the aim of getting legally binding targets. Significant progress was made in the REDD+ (Reduced Emissions from Deforestation and Forest Degradation), including safeguards for local people. It was, however, at COP18 in Doha in December 2012 that a second commitment period starting in January 2013 was agreed, to last eight years. This ensured that all Kyoto mechanisms and accounting rules remained intact for this period, and that parties could review their commitments with a view to increasing them. All this laid the foundations for the possibility of a future global climate agreement, which was agreed at COP21 in Paris in 2015. The climate negotiations in Paris 2015 were a huge success primarily because the French hosts understood the grand game of international negotiation and used every trick in the book to get countries to work together to achieve an agreement signed by all (Lewis, 2015). The agreement states that the parties will hold temperatures to “well below 2°C above pre-industrial levels and to pursue efforts to limit the temperature increase to 1.5°C above pre-industrial levels”. Paris was a high-stakes game of geopolitical poker. Surprisingly, the least powerful countries did much better than expected. The climate talks were subject to a series of shifting alliances going beyond the usual income-rich northern countries and income-poor global south countries. Central to this was, firstly, the US-Chinese diplomacy as both agreed to limit emissions. Secondly, a new grouping of countries called the Climate Vulnerable Forum forced the 1.5°C target higher up the political agenda, so much so that it is mentioned in the key aims of the agreement (Lewis, 2015). Political support from the Paris Agreement allowed the IPCC to write the seminal 1.5˚C global warming report which was published in 2018. This report documented the significant increase in the impact between a 1.5˚C and 2.0˚C world (IPCC, 2018). It also documented how a 1.5˚C world could be achieved - which in essence shows that the world must have net zero carbon emissions by 2050 and then carbon must be taken out of the atmosphere for the rest of the century. The quicker the world gets to net zero the less carbon needs to be extracted from the atmosphere between 2050 and 2100 (Goodall, 2020). The Paris Agreement was just the start of the process because taking into account all the country pledges and assuming that they will be fulfilled then the world would still warm by about 3˚C (Maslin, 2019). The role of global environmental social movements There have been three main waves of environmental social movements. The first was in the late 1980s and early 1990s and provided global support for the Rio Summit. The second wave was in 2008 and 2009, focusing on the hope of a major climate deal at the Copenhagen climate conferences. In the UK it was very successful and lead to the Climate Change Act in 2008 (Bryne, 2019). As we know, Copenhagen ended in abject failure due to the lack of international leadership, sabotage by the US, lobbying by powerful climate change deniers and the global 53 worries about dealing with the 2008 global financial crash (Maslin, 2021). For almost 10 years the global environmental movement was held back due to the focus on the global economy. This all changed in 2018. The third wave of the global environmental social movement started in 2018 (Figueres and Rivett-Carnac, 2020). In May 2018 Extinction Rebellion was set up in the UK and launched in October 2018 with over 100 academics calling for action on climate change. The aim of Extinction Rebellion is to use non-violent civil disobedience to compel governments around the world to avoid tipping points in the climate system and biodiversity loss to avoid both social and ecological collapse (Lewis and Maslin, 2018). In November 2018 and April 2019 they brought central London to a standstill, and Extinction Rebellion has now spread to at least 60 other cities around the world. In August 2018, Greta Thunberg - at the age of 15 - started to spend her school days outside the Swedish Parliament holding a sign saying Skolstrejk för klimatet (School strike for climate) calling for stronger action on climate change. Soon other students all around the world started similar school strikes once a month on a Friday and they called the movement ‘Fridays for Future’ (Thunberg, 2019). It has been estimated that by the end of 2019 there were over 4500 strikes across over 150 countries, involving 4 million school children and this has rising further in 2020 (Fridays for Future, 2020). In 2018 and 2019 three extremely influential IPCC reports were published. First, in 2018, was the Special Report on Global Warming of 1.5°C which documented what the world needed to do if global temperature rise was to be kept at only 1.5˚C (IPCC, 2018). It also showed the positive and negative interactions of climate change mitigation and the Sustainable Development Goals. The second was the special report on the land and how climate change would impact desertification, land management, food security, and the terrestrial ecosystems (IPCC, 2019a). The third was the IPCC Special Report on the Ocean and Cryosphere showing the impacts of climate change on the speed of melting of ice sheets, mountain glaciers and sea ice, and their implications of sea level rise and marine ecosystems (IPCC, 2019b). This new social movement and the very latest science inspired many corporations to take a leading role (Hawken, 2018). Microsoft has set the agenda for the technology sector with the ambitious target to become carbon negative by 2030. By 2050 they want to remove all the carbon pollution from the atmosphere that they and their supply chain have emitted since the founding of the company in 1975. Sky has set the agenda for the media sector; as they are already carbon neutral they have pledged that they and their supply chain will go carbon negative by 2030. BP has also declared that it will be carbon neutral by 2050 by eliminating or offsetting over 415 million tons of carbon emissions. These companies form part of a group of over 850 global companies that have pledged to adopt Science Based Targets, meaning, in effect, that they will all have achieved net zero carbon emissions by 2050 (CDP, 2020). 54 Given all this pressure in 2019, governments all around the world started to declare that we are, in fact, in a climate emergency and action has to be taken. At the time of the publication of this article, over 1,400 local governments and over 35 countries have made climate emergency declarations. Despite the fact that in 2020 the whole world was focused on dealing with the Covid-19 pandemic, climate change remained a major issue (Jones and Maslin, 2020). Glasgow 2021 This new wave of public global environmental concern meant there were great expectations for COP26 in Glasgow at the end of 2020, co-hosted by the UK and Italy. But due to the Covid-19 pandemic, the resultant lockdowns, and the major impact on both Italy and Britain, this pivotal meeting was postponed until November 2021. This meeting is critical because it is the third meeting of the parties to the 2015 Paris Agreement (CMA3) and is the first global stock-take outlined in the Paris Agreement. COP26 will review the progress made since 2015 and encourage greater commitments and pledges from countries to cut their greenhouse gas emissions. Importantly this will be the first COP meeting where ‘net zero’ carbon emissions targets will be the primary global ambition, and the discussion will be about how fast this can be achieved and which countries will lead (Hawken, 2018; Figueres and Rivett-Carnac, 2020; Mann, 2021). Despite 2020 and 2021 being dominated by the Covid-19 pandemic, the geopolitical landscape around climate change has seismically shifted. First, in June 2019, the UK parliament amended the Climate Change Act (2008) to require the government to reduce the UK’s net emissions of greenhouse gases by 100% relative to 1990 levels by 2050. Second, the European Commission is proposing that the EU reduces its GHG emissions by at least 55% by 2030 from 1990 levels, instead of the 40% cut agreed six years ago. This target would be written into EU law and made binding on all 27-member states. This is a major step towards the EU pledge of matching the UK ambition of reaching carbon neutrality by 2050. Third, in September 2020 China’s President Xi Jinping announced via video-link to the UN General Assembly in New York that the country would aim to reach peak emissions before 2030, followed by a long-term target to become carbon neutral by 2060. China is the world’s largest carbon emitter accountable for around 28% of global GHG emissions and up to now has not committed to a long-term emissions goal. Under the Paris Agreement, China had pledged to cut the carbon intensity of its economy by 6065% against a 2005 baseline. This announcement came after long and detailed discussions between China and the EU concerning climate change. COP26 marks the re-engagement with the USA, second largest emitter of around 15% of global GHG emissions. In 2017 the Paris Agreement had a major setback. President Trump declared he was taking the USA out of the Agreement, as he believed it was unfair and biased towards developing countries. In accordance with Article 28 of the Paris Agreement, a country cannot give notice of withdrawal from the Agreement before three years of its start date in the relevant country. So, the earliest possible effective withdrawal date by the United States was November 4, 2020—one day after the 2020 U.S. presidential election. President Biden has already rejoined the Paris Agreement and is a clear advocate of collective international action to deal with 55 climate change. He has appointed John Kerry as United States Special Presidential Envoy for Climate, which has been made a cabinet position. The new President faces additional challenges because over the four years of the Trump presidency nearly 100 environmental rules and regulations have been rescinded or are in the process of being removed. These included rolling back the Obama administration's fuel efficiency and emissions standards for vehicles, reductions in their coal emissions standards for coal-fired power plants, and weakening the efficient lighting regulation, meaning less efficient light bulbs can still be purchased after 2020. President Biden is already undoing many of these changes through executive orders. For example President Trump also gave the executive orders to approve two controversial oil pipelines, Keystone XL and Dakota Access. In 2018, plans were announced to allow drilling in nearly all US waters, creating a huge expansion of offshore oil and gas leases. In 2019, the Trump administration completed plans for opening the entire coastal plain of the Arctic National Wildlife Refuge to drilling. All of these are in the process of being rescinded. For the first time in over a decade there is now hope that the nations of the world can cut greenhouse gas emissions significantly and start the journey to a cleaner, greener, safer, healthier and more sustainable world. Conclusion In the last 30 years the amount of human-emitted carbon dioxide has doubled. This represents a collective failure of the world’s leaders to focus on this issue. As a consequence, the ambition of the climate change negotiations has increased. The Kyoto Protocol aimed for developed countries to cut emissions by 5.2% relative to their 1990 levels, while the Glasgow COP26 will aim to get all countries to agree to be net carbon zero as early as possible in this century. No one is underestimating how difficult but important it is to get a deal in Glasgow. 56 Climate Models How We Use Models Models help us to work through complicated problems and understand complex systems. They also allow us to test theories and solutions. From models as simple as toy cars and kitchens to complex representations such as flight simulators and virtual globes, we use models throughout our lives to explore and understand how things work. Climate Models, and How They Work This image shows the concept used in climate models. Each of the thousands of 3-dimensional grid cells can be represented by mathematical equations that describe the materials in it and the way energy moves through it. The advanced equations are based on the fundamental laws of physics, fluid motion, and chemistry. To "run" a model, scientists specify the climate forcing (for instance, setting variables to represent the amount of greenhouse gases in the atmosphere) and have powerful computers solve the equations in each cell. Results from each grid cell are passed to neighboring cells, and the equations are solved again. Repeating the process through many time steps represents the passage of time. Image 57 source: NOAA (http://celebrating200years.noaa.gov/breakthroughs/climate_model/modeling_schematic.html). Climate models are based on well-documented physical processes to simulate the transfer of energy and materials through the climate system. Climate models, also known as general circulation models or GCMs, use mathematical equations to characterize how energy and matter interact in different parts of the ocean, atmosphere, land. Building and running a climate model is complex process of identifying and quantifying Earth system processes, representing them with mathematical equations, setting variables to represent initial conditions and subsequent changes in climate forcing, and repeatedly solving the equations using powerful supercomputers. Check out The Very, Very Simple Climate Model » (https://scied.ucar.edu/simple-climate-model) Climate Model Resolution Climate models separate Earth's surface into a three-dimensional grid of cells. The results of processes modeled in each cell are passed to neighboring cells to model the exchange of matter and energy over time. Grid cell size defines the resolution of the model: the smaller the size of the grid cells, the higher the level of detail in the model. More detailed models have more grid cells, so they need more computing power. Climate models also include the element of time, called a time step. Time steps can be in minutes, hours, days, or years. Like grid cell size, the smaller the time step, the more detailed the results will be. However, this higher temporal resolution requires additional computing power. How are Climate Models Tested? Once a climate model is set up, it can be tested via a process known as “hind-casting.” This process runs the model from the present time backwards into the past. The model results are then compared with observed climate and weather conditions to see how well they match. This testing allows scientists to check the accuracy of the models and, if needed, revise its equations. Science teams around the world test and compare their model outputs to observations and results from other models. Using Scenarios to Predict Future Climate Once a climate model can perform well in hind-casting tests, its results for simulating future climate are also assumed to be valid. To project climate into the future, the climate forcing is set to change according to a possible future scenario. Scenarios are possible stories about how quickly human population will grow, how land will be used, how economies will evolve, and the atmospheric conditions (and therefore, climate forcing) that would result for each storyline. In 2000, the Intergovernmental Panel on Climate Change (IPCC) issued its Special Report on Emissions Scenarios (SRES) 58 (http://www.ipcc.ch/ipccreports/sres/emission/index.php?idp=0), describing four scenario families to describe a range of possible future conditions. Referred to by letter-number combinations such as A1, A2, B1, and B2, each scenario was based on a complex relationship between the socioeconomic forces driving greenhouse gas and aerosol emissions and the levels to which those emissions would climb during the 21st century. The SRES scenarios have been in use for more than a decade, so many climate model results describe their inputs using the letter-number combinations. In 2013, climate scientists agreed upon a new set of scenarios that focused on the level of greenhouse gases in the atmosphere in 2100. Collectively, these scenarios are known as Representative Concentration Pathways or RCPs. Each RCP indicates the amount of climate forcing, expressed in Watts per square meter, that would result from greenhouse gases in the atmosphere in 2100. The rate and trajectory of the forcing is the pathway. Like their predecessors, these values are used in setting up climate models. Learn more about RCPs » (http://www.skepticalscience.com/rcp.php?t=1) Results of Current Climate Models Around the world, different teams of scientists have built and run models to project future climate conditions under various scenarios for the next century. So the groups can make a fair comparison of their results, they run the same experiment. Because each climate model is slightly different, the results show a range of projections. Though yearly values projected for temperature and precipitation differ among the models, the trend and magnitude of change is fairly consistent. Global climate model results from groups around the world project that global temperature will continue to increase. They also show that human decisions and behavior we choose today will determine how dramatically climate will change in the future. How are Climate Models Different from Weather Prediction Models? Unlike weather forecasts, which describe a detailed picture of the expected daily sequence of conditions starting from the present, climate models are probabilistic, indicating areas with higher chances to be warmer or cooler and wetter or drier than usual. Climate models are based on global patterns in the ocean and atmosphere, and records of the types of weather that occurred under similar patterns in the past. 59 Climate Change Projections How computer models help us understand climate The most powerful computers on Earth are used to run climate models. Scientists use these models to understand how Earth’s climate works and to make predictions about how it might change in the future. Climate models have successfully helped reconstruct climates of the distant and recent past, answering important questions, such as “what caused the last ice age?”. The proven ability of climate models to describe Earth’s past and present climates gives us confidence that they can simulate the planet’s future climate, too. There are many research centres and thousands of climate scientists creating and fine-tuning computerized climate models worldwide. For example, the Met Office Hadley Centre for Climate Science and Services in England is one of the world’s leading climate research institutions. It has over 200 staff dedicated to climate research and uses clusters of supercomputers to create some of the most effective climate models in the world. Here in Canada, the federal Canadian Centre for Climate Modelling and Analysis (CCCma) has been creating excellent climate models since the early 1980s. Climate scientists at institutions like the Hadley Centre and the CCCma combine scientific research and advanced computing to predict what the climate will look like in the decades to come. General Circulation Models The first computer models describing global warming were created in the 1960s. They calculated changes in the temperature of the planet by modelling the balance between the energy coming in from the sun and the energy escaping Earth’s atmosphere back into space. The scientific community has been improving on these first models for over 50 years now. Modern climate models are called "General Circulation Models" or “Earth Systems Models.” They address much more than the sun-Earth energy balance. Working from the foundations of physics and chemistry, they take thousands of factors into account to model the entire climate system, including solar radiation, greenhouse gas emissions, volcanic eruptions, cloud formation, ocean currents, chemical reactions in the atmosphere, land use changes, and much more. New climate models are constantly improving our understanding of the climate system and our improving understanding of the climate system is improving the models. The overall conclusions of the models have not changed—human greenhouse gas emissions are driving global warming. How do we know climate models work? 60 Models allow scientists to test their understanding of how the climate works and how it might change in the future. But do the models do a good job of simulating the real world? Climate models are extensively tested by “hindcasting”, which means modelling the climate of the past. Climate models are considered successful only if they can recreate to a high degree the averages, extremes, and seasonal patterns that match up with observed climate. There is one major difference between predicting climate and predicting weather. A weather forecast attempts to create very precise hour-by-hour predictions on a very small scale. Climate models, on the other hand, effectively combine many simulations of possible weather to produce the accumulated story of what average and extreme conditions might happen. In other words, the goal of a climate model is to project changes in climates over years, decades and longer, whereas weather predictions are interested in what is expected to happen in the coming days. Climate models do create simulations of day-to-day weather over many years, but in no way are they meant to be used to state what the weather is expected to be in the future, on any particular day or sequence of days. Instead, these daily simulations are interpreted statistically, resulting in statements about the probability of particular weather conditions being observed in the future. They do not produce weather forecasts; they produce climate projections. And importantly, scientists keep track of how well models perform as the years pass. Since 1990, for example, the observed rate of global warming is well within the original range projected by climate models. Why do we use so many climate models? Canada is one of dozens of countries that has independently produced its own climate models. International organizations help coordinate all of these modelling experiments and gather them into an “ensemble”, or collection of many different models. Why do this? Although each model is carefully designed to be consistent and plausible, working with an ensemble of many models lets us look at a range of future projections instead of just one. Working with ensembles lets us do a better job of taking natural climate variability into account, helps eliminate the effects of modelling uncertainty, and means that our conclusions are not biased by the weaknesses or strengths of any one model on its own. Comparing the results of many different models makes one thing very clear: all climate models clearly indicate that temperatures will continue to rise as greenhouse gas emissions accumulate in the atmosphere. 61 Key Topic 3: Risks and Impacts to Natural Resources and Society from a Changing Climate 10. Explain the consequences of climate changes on aquatic, forest, wildlife and soil ecosystems. 11. Describe the social and economic impacts of climate change. Study Resources Resource Title Source Located on Climate Impacts on Ecosystems US Environmental Protection Agency, 2017 Pages 63-66 Impacts to Canadian agriculture from climate changes Agriculture and Agri-Food Canada, 2020 Pages 67-70 Impacts on ecosystems and fisheries Fisheries and Oceans Canada, 2019 Pages 71-74 5 Ways Climate Change Impacts Forests North Carolina State University, Forestry and Environmental Resources Research, 2021 Pages 75-78 How Climate Change Impacts the Economy Renee Cho, Columbia Climate School, 2019 Pages 79-84 This is how climate change could impact the global economy Natalie Marchant, Article in World Economic Forum, 2021 Pages 85-86 Earth Observation to Mitigate Impacts of Climate Change and Support Sustainable Business Decisions L3HARRIS GEOSPATIAL, 2022 Pages 87-94 Social Dimensions of Climate Change The World Bank, 2022 Pages 95-96 The Disproportionate Impact of Climate Change on Indigenous Communities Paige Laduzinsky, KCET, 2019 Pages 97101 Southeast Asia to bear the brunt of worsening global climate, IPCC warns Robin Hicks, Eco-Business, 2021 Pages 102105 Climate change triggers mounting food insecurity, poverty, and displacement in Africa World Meteorological Organization (WMO), 2021 Pages 106109 *Please Note: Hyperlinks found in text are not considered required reading; however, included video links are required to watch.* Study Resources begin on the next page 62 Climate Impacts on Ecosystems Key Points Climate change can alter where species live, how they interact, and the timing of biological events, which could fundamentally transform current ecosystems and food webs. Climate change can overwhelm the capacity of ecosystems to mitigate extreme events and disturbance, such as wildfires, floods, and drought. Mountain and arctic ecosystems and species are particularly sensitive to climate change. Projected warming could greatly increase the rate of species extinctions, especially in sensitive regions. Overview Climate is an important environmental influence on ecosystems. Changing climate affects ecosystems in a variety of ways. For instance, warming may force species to migrate to higher latitudes or higher elevations where temperatures are more conducive to their survival. Similarly, as sea level rises, saltwater intrusion into a freshwater system may force some key species to relocate or die, thus removing predators or prey that are critical in the existing food chain. Climate change not only affects ecosystems and species directly, it also interacts with other human stressors such as development. Although some stressors cause only minor impacts when acting alone, their cumulative impact may lead to dramatic ecological changes. For instance, climate change may exacerbate the stress that land development places on fragile coastal areas. Additionally, recently logged forested areas may become vulnerable to erosion if climate change leads to increases in heavy rain storms. Changes in the Timing of Seasonal Life Cycle Events For many species, the climate where they live or spend part of the year influences key stages of their annual life cycle, such as migration, blooming, and reproduction. As winters have become shorter and milder, the timing of these events has changed in some parts of the country: Range Shifts As temperatures increase, the habitat ranges of many North American species are moving north and to higher elevations. In recent decades, in both land and aquatic environments, plants and animals have moved to higher elevations at a median rate of 36 feet (0.011 kilometers) per decade, and to higher latitudes at a median rate of 10.5 miles (16.9 kilometers) per decade. 63 While this means a range expansion for some species, for others it means movement into less hospitable habitat, increased competition, or range reduction, with some species having nowhere to go because they are already at the top of a mountain or at the northern limit of land suitable for their habitat. These factors lead to local extinctions of both plants and animals in some areas. As a result, the ranges of vegetative biomes are projected to change across 520% of the land in the United States by 2100. For example, boreal forests are invading tundra, reducing habitat for the many unique species that depend on the tundra ecosystem, such as caribou, arctic foxes, and snowy owls. Other observed changes in the United States include a shift in the temperate broadleaf/conifer forest boundary in the Green Mountains of Vermont; a shift in the shrubland/conifer forest boundary in New Mexico; and an upward elevation shift of the temperate mixed/conifer forest boundary in Southern California. As rivers and streams warm, warmwater fish are expanding into areas previously inhabited by coldwater species. As waters warm, coldwater fish, including many highly-valued trout and salmon species, are losing their habitat, with projections of 47% habitat loss by 2080. In certain regions in the western United States, losses of western trout populations may exceed 60 percent, while in other regions, losses of bull trout may reach about 90 percent. Range shifts disturb the current state of the ecosystem and can limit opportunities for fishing and hunting. See the Agriculture and Food Supply Impacts page for information about how habitats of marine species have shifted northward as waters have warmed. Food Web Disruptions The impact of climate change on a particular species can ripple through a food web and affect a wide range of other organisms. For example, the figure below shows the complex nature of the food web for polar bears. Not only is the decline of sea ice impairing polar bear populations by reducing the extent of their primary habitat, it is also negatively impacting them via food web effects. Declines in the duration and extent of sea ice in the Arctic leads to declines in the abundance of ice algae, which thrive in nutrient-rich pockets in the ice. These algae are eaten by zooplankton, which are in turn eaten by Arctic cod, an important food source for many marine mammals, including seals. Seals are eaten by polar bears. Hence, declines in ice algae can contribute to declines in polar bear populations. 64 Buffer and Threshold Effects Ecosystems can serve as natural buffers from extreme events such as wildfires, flooding, and drought. Climate change and human modification may restrict ecosystems’ ability to temper the impacts of extreme conditions, and thus may increase vulnerability to damage. Examples include reefs and barrier islands that protect coastal ecosystems from storm surges, wetland ecosystems that absorb floodwaters, and cyclical wildfires that clear excess forest debris and reduce the risk of dangerously large fires. In some cases, ecosystem change occurs rapidly and irreversibly because a threshold, or "tipping point," is passed. One area of concern for thresholds is the Prairie Pothole Region in the north-central part of the United States. This ecosystem is a vast area of small, shallow lakes, known as "prairie potholes" or "playa lakes." These wetlands provide essential breeding habitat for most North American waterfowl species. The pothole region has experienced temporary droughts in the past. However, a permanently warmer, drier future may lead to a threshold change—a dramatic drop in the prairie potholes that host waterfowl populations, which subsequently provide highly valued hunting and wildlife viewing opportunities. Similarly, when coral reefs become stressed from increased ocean temp