Chapter 6 Oils PDF
Document Details
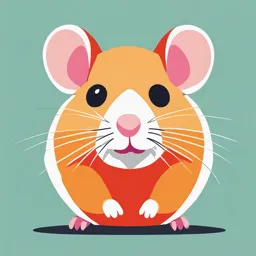
Uploaded by BoomingCourage7332
2008
Frank D. Gunstone
Tags
Summary
This chapter focuses on the chemical properties of oils and fats, highlighting important reactions like hydrogenation and oxidation. It discusses various methods of hydrogenation, emphasizing their impact on the nutritional and physical properties of oils, as well as emphasizing the significance of these chemical processing methods in the food industry. It also covers relevant aspects of thermal changes and other reactions related to food-grade oils.
Full Transcript
Chemical Properties This chapter covers the chemical reactions that are significant in the food industry and include hydrogenation, oxidation, thermal changes in double bond systems, and some reactions of the acid/ ester function. 6.1 Hydrogenation As generally practised hydrogenation involves re...
Chemical Properties This chapter covers the chemical reactions that are significant in the food industry and include hydrogenation, oxidation, thermal changes in double bond systems, and some reactions of the acid/ ester function. 6.1 Hydrogenation As generally practised hydrogenation involves reaction between the unsaturated centres in an oil or fat in the presence of a metallic catalyst. This is a heterogeneous reaction, involving solid, liquid, and gas, taking place on the solid catalyst surface at an appropri- ate temperature and pressure. After hydrogenation, the product has changed physical, chemical, and nutritional properties. Sometimes compromises have to be made between these. Each year millions of tonnes of soybean and other unsaturated vegetable oils containing oleic, linoleic, and linolenic acids as well as diminishing levels of fish oils with more complex patterns of unsaturation are subject to hydrogenation. This reaction is prac- tised in three major ways: (1) Brush hydrogenation is a short reaction, applied particularly to soybean and rapeseed/canola oils, designed to reduce the level of linolenic esters (18:3) to around 4%, thereby increas- ing shelf life. This is not a very extensive hydrogenation proc- ess. Apart from the lowering of the level of linolenate the fatty acids are little changed and production of trans isomers will be minimal. The partially reduced linolenate will be con- verted not to linoleate but to a mixture of 18:2 isomers and Oils and Fats in the Food Industry: Food Industry Briefing Series. Frank D. Gunstone. © 2008 Blackwell Publishing Ltd, ISBN: 978-1-4051-7121-2 71 72 perhaps some 18:1 (not oleate). Table 2.5 contains data INDUSTRY on the composition of various products when soybean oil of iodine value (IV) 132 is hydrogenated progressively to oil of IV 110 (brush hydrogenation), 97, 81, and 65. (2) Partial hydrogenation is an important way of processing oils and fats to extend their range of use. The technique, first applied to such materials by the German chemist Normann, has been in operation for over 100 years and has been sub- ject to continuous improvement during that time. The main objective is to convert a liquid oil (vegetable or fish) into a semi-solid fat that can be used as a component of a spread. FOOD Compared to brush hydrogenation this is a more extensive reaction whereby the content of polyunsaturated fatty acid is much reduced and a considerable proportion of trans 18:1 is formed. This results in a rise in melting point (because trans esters are higher melting than their cis isomers) that affects spreadability, oral response, and baking performance. THE Two other changes have also to be considered. There is an increase in oxidative stability through the complete or partial removal of the polyunsaturated fatty acids that are so easily oxidised and there is a decrease in nutritional value through IN the destruction of essential fatty acids and the formation of saturated acids and of unsaturated acids with trans con- figuration. During hydrogenation linoleate is reduced first FATS to a mixture of cis and trans 18:1 isomers referred to as ‘oleate’ and then to stearate. The level of stearic acid may also increase (Table 2.5) but it has been argued that this is not a serious cholesterol-raising fatty acid. Along with rumi- nant fats, partially hydrogenated vegetable oils are the most AND important source of trans acids and, following the concern over these acids, there is now a requirement in some coun- tries to indicate their level on the product label. As a conse- quence other ways to achieve the desired physical properties have been explored. These include modifications of the OILS hydrogenation process to give less trans compounds and using interesterification of suitable blends as an alternative approach. Concern over trans acids is based on the fact that they raise LDL (low-density lipoproteins) levels and lower HDL (high-density lipoproteins) levels. This has been reported to be mainly a US problem because 75% of the fats consumed in that country are derived from soybean oil with its high 73 levels of polyunsaturated fatty acid. According to US legis- 6 lation samples containing less than 0.5 g of non-conjugated trans acids per 14 g serving may be claimed as zero trans. CHAPTER (3) Complete hydrogenation is a still more extensive process in which virtually all unsaturated acids are converted to their saturated analogues. This produces ‘hardstock’ which can be blended with a liquid (unsaturated) oil and subsequently interesterified. Hardstock is rich in stearic acid formed from the unsaturated C18 acids originally present. It usually has an IV of around 2. It contains only low levels of unsaturated acid and therefore very little trans acids. However, it may be neces- sary for the word ‘hydrogenated’ to appear on the label. At the molecular level one or more of the following changes may occur during this reaction: hydrogenation (saturation) of unsat- urated centres, stereomutation of natural cis olefins to their higher- melting trans isomers, double bond migration, and conversion of polyunsaturated fatty acids to monounsaturated and saturated acids. These are the consequences of reaction between a liquid (fatty oil) and a gas (hydrogen) occurring at a solid surface (the catalyst). In the sequence below the horizontal line shows the con- version of diene to monoene and of monoene to saturated acid/ ester via the half hydrogenated states represented as DH and MH. The steps shown vertically are the reverse processes whereby DH reverts to D or a diene isomer and MH reverts to M or a monoene isomer. It is during these reverse stages that trans and positional isomers are formed. There are six stages altogether and it is import- ant to understand the relative rates of these. In the conversion of D to M the first step is rate-determining and the second step is fast. Levels of DH will therefore be low and the conversion of DH back to D is slow and only important in the unusual situation that hydrogen is present in very low concentration. In the conversion of M to S the final stage is slow and rate-determining. This makes it more likely that there will be considerable recycling of M and MH leading to formation of stereochemical and positional isomers. D DH M MH S D M The catalyst used on a commercial scale is nickel on an inert sup- port at a 17–25% level, encased in hardened fat. This preserves 74 the activity of the nickel in a form that is easily and safely handled. INDUSTRY Hydrogenation is generally conducted at 180–200C and 3 bar pressure in vessels containing up to 30 tonnes of oil. To minimise the use of catalyst it is desirable to use refined oil and the highest quality of hydrogen. Through improvements in the quality of cata- lyst and in equipment the requirement for catalyst has been grad- ually reduced. In 1960, 0.2% nickel was required but by the end of the century this was reduced 4- to 8-fold to between 0.025% and 0.05%. Reaction may proceed in a batch-wise manner with up to 8–10 batches in a 24-hour day or in a semi-continuous fashion at rate of 25–100 tonnes per day. The reaction is exothermic and appro- FOOD priate cooling is required as well as stirring to distribute the heat. Several significant variables have to be considered: The nature of the oil being treated. The extent of hydrogenation which is desired. The selectivity to be achieved in terms of PUFA–MUFA-saturated THE ratios and the ratio of cis to trans isomers. The quality and quantity of catalyst in terms of pore length, pore diameter, activity, and amount used. The reaction conditions of temperature, pressure, and degree IN of agitation. The competition between hydrogenation (a change incorporat- FATS ing hydrogen) and isomerisation (a change not involving additional hydrogen in the product) depends on the availability of hydrogen at the catalyst surface in relation to the demand. A plentiful supply of hydrogen will promote hydrogenation, and an inadequate supply of hydrogen will allow isomerisation to become more significant. AND The availability of hydrogen at the catalyst surface is enhanced by increased pressure and increased agitation. The demand for hydro- gen is increased with higher temperatures, higher catalyst quantity, higher catalyst activity, and more highly unsaturated oils. The progress of the reaction can be followed in a number of ways OILS that vary in simplicity, in speed of completion, and in the informa- tion they provide. They include: the volume of hydrogen used which will measure saturation but not isomerisation, iodine value meas- ured by an accelerated technique that will provide similar informa- tion, refractive index, solid fat content measured by low-resolution 1 H-NMR, solid fat index measured by dilatometry, slip melting point, or gas chromatography of methyl esters. 75 Koetsier in Gunstone and Padley (1997) has summarised data 6 on the solubility of hydrogen in vegetable oil. This information is obviously important for hydrogenation. He cites solubility values CHAPTER (maximum concentration in oil at a given temperature and pres- sure) from two sources at 1 bar and 100–200C of 2.60–3.36 and 2.76–3.40 mol/m3. The concentration of hydrogen is thus much lower than the concentration of unsaturated centres and for a fish oil of iodine value hydrogenated at 5 bar and 180C Koetsier gives concentrations of ⬃7000 and 16 mol/m3, respectively, for the olefinic groups and the hydrogen. 6.2 Atmospheric oxidation Unsaturated fats like other unsaturated products such as rubber and paints deteriorate as a consequence of reaction with oxygen (air) that leads, in fat-containing foods, to oxidative rancidity (another form of rancidity results from hydrolysis). Oxidative deterioration is of two kinds (autoxidation and photo-oxidation). These processes lead first to similar, but not identical, unstable allylic hydroperoxides which decompose to volatile short-chain molecules (mainly alde- hydes) responsible for the undesirable odours and flavours associ- ated with oxidative rancidity (Figure 6.1). These compounds have low but differing threshold levels so that only small quantities are necessary to produce their undesirable effects. Since the oxidation processes are influenced by heat, light, the presence of pro-oxidants (copper and iron) and antioxidants (natural or synthetic), the pres- ence of already-oxidised material, and of air, attention must be given Olefinic acids/esters (methyl oleate, linoleate, etc., glycerol esters, oils and fats) ↓ Allylic hydroperoxides (highly reactive species) ↓ Volatile compounds of lower molecular weight (aldehydes, etc.) which provide odour and flavour, often at low concentration. Compounds with the same chain length: rearrangement products, products of further oxidation, and products of reaction with other components in the reaction system. Compounds of higher molecular weight such as dimers and polymers. Figure 6.1 Formation and further reactions of allylic hydroperoxides. 76 to all these factors in the handling, transport, and storage of oils INDUSTRY and fats and of foods containing these. The level and nature of unsaturation is an important factor in the rate of oxidation (Table 6.1). This is the reason for the instability of (highly unsaturated) fish oils and accounts for the short shelf life of oils containing linolenic acid (soybean and rapeseed/canola oils) and the preference for oils with reduced levels of this acid avail- able through breeding or through brush hydrogenation. The figures in Table 6.1 indicate that the polyunsaturated acids (linoleic, lino- lenic, etc.) oxidise much quicker than the monounsaturated oleic acid. Oxidative deterioration is strongly linked with the number of FOOD doubly activated allylic groups present in PUFA. An index of the oxi- disability of vegetable and fish oils has been expressed as: Oxidisability (diene) 2(triene) 3(tetraene) 4(pentaene) 5(hexaene) THE It is not possible to prevent these oxidation reactions but they can be inhibited and the induction period (Section 4.6) should be extended as much as possible. The difficulties of inhibiting oxidation are further complicated by the fact that many foods (and biological IN systems) are emulsions of lipids and aqueous systems and attention has to be given to the distribution of antioxidants and pro-oxidants between these two phases and at the interface between them. FATS The detailed structure of the hydroperoxides that can result from oleate, linoleate, or other polyunsaturated acid is important as this controls the chemical structure of the volatile short-chain compounds, each of which has its own flavour/odour and its own threshold level. One of the problems with linolenic esters is that AND trienes oxidise quicker than dienes and the resulting volatile alde- hydes have lower threshold values. When an oxidised fatty mol- ecule cleaves one fragment will remain attached to glycerol (the core aldehyde) while the methyl end of the molecule will provide OILS Table 6.1 Relative rates of autoxidation and photo-oxidation of oleate, linoleate, and linolenate (autoxidation of methyl oleate 1) Reaction Oxygen 18:1 18:2 18:3 Autoxidation Triplet 1 27 77 Photo-oxidation Singlet 3 104 4 104 7 104 Ratio of reaction rates 30,000 1500 900 77 the volatile component. The latter can be removed through refining 6 but the short-chain fragment still attached to glycerol will probably remain in the oil. The sequence in Figure 6.2 shows the formation CHAPTER of octanal from a glycerol ester containing oleic acid via the 11-hydroperoxide. Other aldehydes from oxidised oleate, linoleate, and linolenate are listed in Table 6.2. Autoxidation is a radical chain process. That means that the intermediates are radicals (odd electron species) and that like other chain processes there are three stages: initiation, propa- gation, and termination (Figure 6.2). The initiation step (not fully understood) is followed by a propagation sequence that continues, perhaps for many cycles, until stopped by one of the termination processes. The process will be accelerated by more initiation steps (involving metal ions, higher temperatures, or a poor sample con- taining already oxidised oil) and by less termination steps result- ing in more of the propagation cycle. More important, the process will be inhibited by having fewer initiation steps or more termin- ation steps thereby having fewer and shorter propagation cycles. This can be achieved by starting with good quality oil and by the Table 6.2 The major hydroperoxides produced from oleate, linoleate, and linolenate during autoxidation and photo-oxidation and the volatile alde- hydes resulting from these Ester Hydroperoxide Double bond Volatile aldehyde* Oleate 8 9 11:1 (2) 9 10 10:1 (2) 10 8 9:0 11 9 8:0 Linoleate 9 10,12 10:2 (2,4) 10 8,12 9:1 (3) 12 9,13 7:1 (2) 13 9,11 6:0 Linolenate 9 10,12,15 10:3 (2,4,7) 10 8,12,15 9:2 (3,6) 12 9,13,15 7:2 (2,4) 13 9,11,15 6:1 (3) 15 9,12,16 4:1 (3) 16 9,12,14 3:0 * Numbers before the colon indicate the number of carbon atoms in each aldehyde molecule and numbers after the colon indicate the number of unsaturated centres. Numbers in brackets indicate double bond position with respect to the aldehyde function. 78 Glyc-OCO(CH2)7CH CH(CH2)7CH3 Glycerol oleate ↓ INDUSTRY Glyc-OCO(CH2)7CH CHCH(OOH)(CH2)6CH3 Allylic hydroperoxide ↓ Glyc-OCO(CH2)8CHO OHC(CH2)6CH3 Core aldehyde and volatile aldehyde Figure 6.2 Formation of a typical core aldehyde and a short-chain volatile aldehyde (octanal) from a glycerol ester through the appropriate allylic hydroperoxide. Other aldehydes result from other hydroperoxides (Table 6.2). Initiation RH → R Resonance-stabilised alkyl radical FOOD Propagation R O2 → RO2 Fast reaction to give a peroxy radical RO2 RH → RO2H R Rate-determining step Termination RO2 RO2 → stable products RO2 R → stable products R R → stable products THE Figure 6.3 Olefin autoxidation. RH represents an olefinic compound in which H is attached to an allylic carbon atom. RO2H is a hydroperoxide. presence of appropriate antioxidants (see below). The initiation and IN propagation steps involve breaking a CH in the glycerol ester. The energy required to remove hydrogen from a saturated methylene group, an allylic methylene group, and a doubly allylic methylene FATS group (as at C11 in linoleate) is 100, 75, and 50 kcal, respectively. These values relate to the relative ease of oxidation of saturated, oleate, and linoleate (Table 6.1). The allyl radical first produced is resonance stabilised so that the radical centre is spread over dif- ferent carbon atoms giving rise to the various hydroperoxides listed AND in Table 6.2. In the oxidation of olefinic lipids there is normally an induction period during which reaction is very slow and deterior- ation is not significant, followed by a quicker stage of undesirable oxidation. One purpose of antioxidants is to extend this induction period. OILS Sterols are also subject to oxidation. Cholesterol (Section 1.5) contains a cyclic double bond (5) and two tertiary carbon atoms in its side chain (C-20 and C-25), all sites where oxidation may occur. Some cholesterol oxides are produced as part of the nor- mal metabolism of cholesterol to bile acids. At higher levels these affect human health by contributing to the development of athero- sclerosis. Oxidised animal-based foods represent a primary source 79 cis-RCH CHCH2R' 1O2 → trans-RCH(OOH)CH CHR' 6 Figure 6.4 Reaction of olefin with singlet oxygen to give allylic hydroper- CHAPTER oxides with double bonds in a different position and of changed configuration. of oxidised cholesterol. Such products are not present in fresh foods but are formed during handling prior to consumption, mainly through autoxidation. Cholesterol esters are predominantly of lino- leic acid while free cholesterol is associated with polyunsaturated fatty acids in phospholipids in cell membranes. In both cases oxi- dation can be initiated in the polyunsaturated fatty acids and then involve the cholesterol molecule. This holds in the animal (before being prepared as food) and in the human, and in both oxidation can be retarded by appropriate dietary antioxidants. Processing conditions should also be adapted to minimise oxidation as, for example, in the preparation of spray-dried eggs. Between 0.5% and 1.0% of dietary cholesterol may be oxidised and the primary oxidation products include 7-␣-hydroxy-, 7--hydroxy, and 7-keto-cholesterol, cholesterol ␣- and -epoxides, 3,5,6-trihydroxycholesterol, and 20- and 25-hydroxycholesterol (Cuppett, 2003). Photo-oxidation is a quicker reaction between olefin and a light- activated form of oxygen. The activation process requires a sen- sitiser such as chlorophyll, riboflavin, myoglobin, erythrosine, rose bengal, or methylene blue. The sensitiser absorbs energy from a photon and this energy is eventually passed to oxygen, raising it from the triplet to the more reactive singlet state. Singlet oxygen reacts rapidly with double bonds by an ene reaction to give an allylic hydroperoxide. Photo-oxidation differs from autoxidation in that it is faster (Table 6.1) and its rate is related to the number of double bonds rather than to the number of doubly allylic functions. It is inhibited by appropriate quencher molecules like carotene rather than by the range of compounds that inhibit autoxidation. Antioxidants are materials present in oils and fats to pro- tect them against autoxidation. They may be natural compounds already present in the oils such as tocols (Section 1.6) and ferulic acid esters (structure below) or they may be natural or synthetic compounds added by the technologist. There is not enough nat- ural antioxidant to meet demand so synthetic compounds must be used in some cases. Some of the natural antioxidants may be lost 80 during refining so that refined oils are generally less stable than INDUSTRY crude oils. The eight different tocols have differing antioxidant activity so that total tocol content is not an adequate measure of antioxidant activity. (Table 6.3 and Section 1.5) Antioxidants may also be lost during food preparation so prepared foods may be less stable than the ingredients from which they are made. Autoxidation can be inhibited (induction period extended and shelf life length- ened) but it cannot be reversed so antioxidants should be added as early as possible after the refining process. The amount of anti- oxidant employed must be the optimum. There are legal limits for the synthetic compounds and tocopherols may act as pro-oxidants FOOD at higher concentrations. In the case of emulsions, as opposed to bulk oils, it is important to consider the distribution of antioxidants and pro-oxidants between the oil and water phases. To avoid the reaction of existing hydroperoxides with metal ions which generates more radical species, it is important to keep hydroperoxides and metal ions apart and this may be assisted by controlling the pH of THE the emulsion and by selection of appropriate emulsifiers. Some plants have other natural antioxidants in their leaves or seeds. Familiar examples include oat oil (with ␣-tocopherol, IN Table 6.3 Vitamin E content (mg/100 g) of some vegetable oils and of butter and lard FATS Tocopherols Tocotrienols Oil ␣  ␥ ␦ Total ␣  ␥ ␦ Total Grand IU total Soybean 10 – 59 26 96 0 96 24 Corn 11 5 60 2 78 0 78 20 AND Rapeseed 17 35 1 53 0 53 30 Sunflower 49 5 1 55 0 55 73 Groundnut 13 22 2 37 0 37 23 Cottonseed 39 39 78 0 78 64 Safflower 37 17 24 80 0 80 61 Palm 26 32 7 65 14 3 29 7 53 118 49 OILS Coconut Trace Trace 1 Trace 2 Trace 3 4 1 Olive 20 1 1 22 0 22 30 Wheat germ 121 65 24 25 235 2 17 19 254 233 Rice 12 4 5 21 18 2 57 77 98 30 Butter 2 2 2 3 Lard 1 1 1 1 2 2 IU represents total vitamin E content calculated on a weighted basis for the effect of each tocol. Source: Adapted from Stone and Papas in Gunstone (2003). See also Table 1.3. 81 ␣-tocotrienol, and avenathramides), sesame oil (with sesamin, 6 sesamolin, and sesaminol, all of which are derivatives of sesamol – 3,4-methylenedioxyphenol), and ricebran oil (with tocotrienols, ave- CHAPTER nasterols, and oryzanols which are sterol esters of ferulic acid). 4-OH-3-OMeC6H3CH CHCOOH ferulic acid Antioxidants are also present in herbs and spices and while these can sometimes be used as extracts their food use is limited by strong flavours that may or may not be acceptable in other foods. Tea leaves are a rich source of antioxidants (catechins) as are many fruits and vegetables containing flavonoids. Dietary con- sumption of these as whole foods provides a good source of the antioxidants required by the body to counter oxidative damage to protein and to DNA caused by radicals produced through lipid oxidation. This applies also to vegetables containing carotenes. Rosemary leaves contain powerful antioxidants such as carnosic acid, carnosol, and rosmarinic acid and rosemary extracts are avail- able for use as antioxidants. Vitamin C (ascorbic acid) acts as an oxygen scavenger, removing traces of residual oxygen in a packed and sealed product. It is water-soluble but can be used in a lipid- soluble form as ascorbyl palmitate. Phospholipids show ill-defined antioxidant activity possibly through activity as a chelating agent and/or emulsifier. The supply of natural antioxidants is insufficient to meet demand so some use of synthetic antioxidants is obligatory. Even so-called natural vitamin E may have been submitted to a chemical reaction (permethylation) in which tocols with only one or two methyl groups have been converted to their trimethyl derivative (␣-tocopherol, Figure 1.5). The synthetic compounds that can be used as antioxidants in food are strictly controlled, as is the level at which they may be used. The matter is complicated in that not all countries have agreed to the same list of acceptable compounds. This becomes important for materials that are traded between countries having different permitted antioxidants. Obviously the antioxidants must be non-toxic and that must apply also to the products produced from them as a result of their antioxidant activity. The four important synthetic antioxidants discussed here are solid compounds and may be conveniently used as solutions in pro- pylene glycol, monoacylglycerols, or vegetable oils. They are mono 82 OH OH OH OH INDUSTRY But But But HO OH But OMe Me COOC3H7 OH BHA BHT PG TBHQ (E320) (E321) (E310) (no E number) Figure 6.5 The structures and E numbers of synthetic antioxidants. TBHQ has no E number because it is not a permitted antioxidant in the EU. FOOD or dihydric phenols (represented as ArOH) and react readily with a peroxy radical to give a phenoxy radical (ArO ) stabilised by exten- sive delocalisation of the odd electron over the aromatic system. THE The E numbers indicate that they may be used in Europe within prescribed limits (Figure 6.5). Butylated hydroxyanisole (BHA, E320) shows good solubility in fat and reasonable stability in fried and baked products. It is very IN effective with animal fats and less so with vegetable oils. It shows marked synergism with BHT (butylated hydroxytoluene) and PG (propyl gallate) and can be used at a maximum level of 200 ppm. FATS BHT, E321 is less soluble than BHA and is not soluble in the propylene glycol frequently used as a solvent for antioxidants. It is synergistic with BHA but not with PG and can be used to a max- imum level of 200 ppm. Synergism is the term used to describe the observation where AND the efficacy of two or more components is greater than the sum of the effects for individual components and indicates some co-operative activity. PG, E310 is less soluble than BHA or BHT. It does not gener- ally survive cooking as it decomposes at 148ºC. Nevertheless it is OILS effective when used with BHA and may be used up to 100 ppm. Tertbutyl hydroquinone (TBHQ) is acceptable in USA and many other countries but not in EU-27 and hence does not have an E number. It is very effective with vegetable oils, has good solubil- ity, and is stable at high temperatures. It is frequently used during oil transport and storage and can be subsequently removed during deodorisation. 83 6.3 Thermal changes 6 CHAPTER Unsaturated centres may undergo undesirable changes when heated. This is particularly important when the fatty acyl chains contain three or more methylene interrupted olefinic centres and when the temperature exceeds 180ºC. This problem may arise dur- ing deodorisation of soybean and rapeseed oils containing up to 10% of linolenic acid (18:3) or of fish oils with eicosapentaenoic acid (EPA) (20:5) and docosahexaenoic acid (DHA) (22:6). At ele- vated temperatures the risk of oxidative change is enhanced but even in the absence of air undesirable changes occur at elevated temperatures. Studies on the refining of vegetable oils have shown that stereoisomerism of linolenic acid with three double bonds occurs from 220ºC upwards and is quicker than for linoleate with only two double bonds. The changes that may occur during expos- ure of polyunsaturated fatty acids to higher temperatures include: cyclisation (formation of five- and six-membered carbocyclic ring systems); geometrical isomerism (conversion of the natural all-cis pol- yunsaturated fatty acid to isomers with both cis and trans double bonds); polymerisation (producing dimers, trimers, and oligomers of enhanced molecular weight). All these changes are undesirable on nutritional grounds since the nutritional value of the polyunsaturated fatty acid depends on them retaining their all-cis pattern of unsaturation. Special analyt- ical methods may be required to detect these changes. 6.4 Reactions of the carboxyl/ester function Oils and fats are glycerol esters and the ester functions are react- ive centres which can be modified in various ways. For example, they can be hydrolysed. The suffix -lysis means splitting so that hydrolysis implies splitting with water and the final products are fatty acids and glycerol. This reaction generally requires a cata- lyst which may be acidic, basic, or enzymatic. When catalysed by 84 a lipase the reaction may be described as lipolysis. Hydrolysis is INDUSTRY an important reaction in the oleochemical industry but in the food industry the conversion of glycerol esters to other esters is more important. Esters can be made from fatty acids and alcohols but it is often more convenient to transform existing esters (such as triacyl- glycerols) to other esters by reaction with an alcohol (alcoholysis), an acid (acidolysis), or another ester (interesterification) using a catalyst that may be chemical or a lipase. Two important alcoholysis procedures are methanolysis and glyc- erolysis. The former is used in the conversion of glycerol esters to FOOD methyl esters and is used on a mg scale for analytical purposes (gas chromatography) and on a multi-tonne scale to produce esters for biofuel or as an intermediate in the production of fatty alcohols. Glycerolysis involves reaction of triacylglycerols with glycerol to pro- duce monoacylglycerols and diacylglycerols. The former, as such or after further modification, are much used in the food industry as THE emulsifying agents (Section 8.11). Acidolysis is less commonly employed but it may be used to incorporate (say) lauric acid (12:0) into a C16/18 oil. Interesterification can be carried out on a single oil, itself a non- IN random mixture of triacylglycerols, or on a blend of oils. An old example of the first of these is the interesterification (randomisa- tion) of lard. This fat is unusual in having a high level of palmitic FATS acid in the sn-2 position. During reaction with sodium methoxide the lard fatty acids become randomly distributed. Overall there is no change in fatty acid composition but a change in triacyl- glycerol composition. As a consequence of this modification the randomised lard is a better shortening. More commonly, interest- AND erification is applied to a blend of oils. The oils that are mixed can differ in their level of unsaturation (an unsaturated oil and a hydro- genated oil), in their range of chain length (such as a lauric oil and a C16/18 oil), or in the presence of a less common fatty acid such as ␥-linolenic acid, EPA, or DHA in one of the components. OILS Mixtures of esters may be interesterified with an appropriate basic catalyst. When this happens the natural non-random mixture is ultimately converted to a randomised mixture. The change will be even more marked with a mixture of two different types of oils such as a lauric oil and a non-lauric oil. Alteration is particularly appar- ent in the fatty acids present in the sn-2 position. Before interest- erification of a vegetable oil these will be mainly unsaturated acids 85 but after complete randomisation the fatty acids at all three pos- 6 itions will be the same. These changes have important effects on the physical (particularly melting behaviour) and nutritional proper- CHAPTER ties of the modified fat. Interesterification is used in newer methods of producing spreads with a reduced content of trans acids. Hydrogenation producing large amounts of trans acids, can be replaced by interesterification of a soft fat with a hard fat. Most spreads produced in Europe now have a very low level of trans acid though this may be less true for cooking fats and industrial spreads. When randomisation is com- plete triacylglycerol composition can be calculated from the fatty acid composition since the amount of an individual triacylglycerol (ABC) will depend only on the proportions of each of these acids (a%, b%, c%, respectively). The level of this single glycerol ester will be 100 [a/100 b/100 c/100]% and the level of all isomers having one A, one B, and one C acyl chain will be six times this fig- ure since there are six stereoisomers meeting this requirement. Ester–ester interchange can be achieved without a catalyst at temperatures above 200C but is usually carried out at 20–100C with a basic catalyst such as sodium hydroxide, sodium meth- oxide, or sodium potassium alloys. At ⬃80C the reaction takes 30–60 min and may be carried out on a multi-tonne scale. The oil should be free of water, carboxylic acid, and hydroperoxide as these compounds will destroy the catalyst. The true catalyst is thought to be either a diacylglycerol anion [ROCOCH2CH(OCOR)CH2O] formed by interaction of a triacylglycerol molecule with sodium methoxide or the enolate [ROCOCH2CH(OCOR)CH2OCO CHR] resulting from removal of a proton from the ␣-methylene function. The product will contain some free acid if NaOH is used as catalyst or methyl ester, if the catalyst is NaOMe and must be refined to remove these. The amount of catalyst must be as low as possible to minimise these losses. Directed interesterification is a modification of the normal pro- cess when the reaction is conducted at a lower temperature (25– 35C). Under these conditions the less soluble triacylglycerols crystallise from the solution. This disturbs the equilibrium in the liquid phase and this will be continually re-established. The conse- quence is to raise the levels of SSS and of UUU triacylglycerols in the final interesterified product. With enzymatic catalysts, interesterification leads to structured lipids of the types described below. 86 Nutritional and other physiological properties of fats (triacyl- INDUSTRY glycerols) depend on their detailed structure. Until now, use has been made of materials provided by agriculture modified in minor ways such as fractionation, partial hydrogenation, and interesterification as described in earlier sections of this chapter. Individual triacyl- glycerols can be synthesised in the laboratory in modest quantities but these will not usually be appropriate for large-scale human consumption. By exploiting the specificity of lipases it is now pos- sible to produce large quantities of oils and fats approximating to a specification designed to optimise some important physical and/or nutritional property. FOOD The cost of the enzyme is generally so high that their use is only economic for high-value products but these difficulties are being overcome as enzyme producers develop immobilised enzymes of greater stability with a longer useful life. At the same time our understanding of enzyme structure allows changes to be made leading to enhanced selectivity. Beyond this there is growing willing- THE ness to pay more for fats for which approved health claims can be made. Enzymatic reactions are considered to be more ‘natural’ or ‘greener’. Lipases show several different kinds of specificity which can be IN exploited. The most common is 1,3-regiospecificity in which reac- tion is confined to the sn-1 and 3 positions of a triacylglycerol with no change at the sn-2 position. Lipases may also show specificity FATS for selected fatty acids with which they associate. This specificity may depend on the unsaturated centres and especially the position of the double bond closest to the carboxyl group or may be related to chain length. There are many reports of preparations of structured triacyl- AND glycerols which can be achieved in one-step or two-step processes. In the former, an oil or fat has some of its sn-1/3 acyl chains replaced by different fatty acids. The acyl donor may be an ester such as an alkyl ester or triacylglycerol mixture or an acid (acidolysis). The reaction is illustrated in very simple form in the following equation. OILS In reality the reaction is more complicated since neither reactant will be the individual species indicated in the equation. Gl-ABC D (as acid or ester) L Gl-DBC Gl-ABD Gl-DBD The products represent racemic triacylglycerols with acyl chains A–D. The sn-2 position is unaffected by this process. 87 Good results have been obtained with lipases such as those 6 from Rhizomucor miehei (Lipozyme), Rhizopus delemar, and Candida antarctica. Incorporation of reactant is usually in the range 40–65%. CHAPTER This procedure is simpler than the two-step reaction but the prod- ucts are less pure. In the two-step process triacylglycerols are selectively deacylated at the sn-1 and 3 positions by enzyme-catalysed ethanolysis and pure 2-monoacylglycerol is isolated. The monoacylglycerol is then acylated at the free hydroxyl positions using a 1,3 specific lipase and an appropriate acyl donor which may be free acid, alkyl ester, or vinyl ester. Gl-ABC → Gl-(OH)B(OH) → Gl-DBD Two-step synthesis of a structured triacylglycerol proceeding through a 2-monoacylglycerol which is isolated and purified before the second step. Gl stands for the glycerol residue; OH represents the free OH groups in a monoacylglycerol; and A, B, C, and D are acyl groups. There is a greater control of the reaction when this is conducted in a carefully selected solvent but this involves additional hand- ling and cost and the aim is to produce bulk products having the desired properties as simply and cheaply as possible. Typically a plug-in reactor (1 m3), containing Lipozyme TL IM prepared from Thermomyces lanuginose lipase is supplied by the enzyme producer. Two oils such as palm oil (or palm stearin) and palm kernel (or coconut) oil are passed through the reactor and emerge 1 h later as interesterified oil. The reaction occurs at 70C which is 30C lower than for chemical interesterification, no downstream processing is required, and the product has no acids with trans unsaturation. This approach is being used to produce spreads with a low level of trans acids. Many laboratory experiments are concerned with attempts to pro- duce triacylglycerols of the type MLM where M is an easily metab- olised fatty acid of medium-chain length (frequently C8) and L is a long-chain acid including nutritionally important fatty acids such as EPA or DHA. The nature of the fatty acid in the sn-2 position is controlled by the selection of starting material. Vegetable oils will provide sources of oleic and linoleic acid in this position and fish oils will be used for the long-chain polyunsaturated fatty acids. In a preliminary stage 88 the levels of these important acids may be enhanced prior to inter- INDUSTRY esterification (Section 2.7). Human milk fat is unusual in that it is rich in triacylglycerols containing a saturated acid (palmitic) in the sn-2 position. This is unusual among natural fats so a product with this structural fea- ture called ‘Betapol’ has been developed for addition to infant for- mula. In theory, tripalmitin is reacted with unsaturated acids in the presence of a 1,3-stereospecific lipase (from Rhizomucor miehei). In practice the reactants are a palm stearin rich in tripalmitin and a mixture of canola and sunflower oils rich in oleic acid. FOOD THE IN FATS AND OILS