Biochemistry I CHM219 Fall 2021 PDF
Document Details
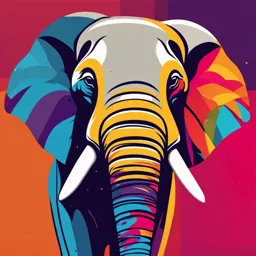
Uploaded by GleefulTanzanite2537
C. Biruni Üniversitesi
2021
Dr. Esra Aydemir
Tags
Summary
These notes cover Biochemistry I CHM219 Fall 2021, focusing on carbohydrates including sugars, saccharides, glycans, monosaccharides, and related topics. The document also provides an outline for the topics.
Full Transcript
BIOCHEMISTRY I CHM219 Assist Prof. Dr. ESRA AYDEMİR Carbohydrates:Sugars, Saccharides, Glycans Outline: Monosaccharides Derivatives of the Monosaccharides Oligosaccharides Polysaccharides Glycoproteins Oligosaccharides as Cell Markers Biosynthesis of Glycoconjugates—Amino...
BIOCHEMISTRY I CHM219 Assist Prof. Dr. ESRA AYDEMİR Carbohydrates:Sugars, Saccharides, Glycans Outline: Monosaccharides Derivatives of the Monosaccharides Oligosaccharides Polysaccharides Glycoproteins Oligosaccharides as Cell Markers Biosynthesis of Glycoconjugates—Amino Sugars Glycoconjugates of Interest Monosaccharides Carbohydrates have numerous functions in biochemistry: generating and storing biological energy molecular recognition (as in the immune system) cellular protection (as in bacterial and plant cell walls) cell signaling cell adhesion biological lubricants controlling protein trafficking maintaining biological structure (e.g., cellulose). Representative carbohydrates: The three compounds shown here are composed entirely of C, H, and O, with glucose forming the monomer for the oligomer and the polymer. a) Glucose, a monosaccharide. b) Maltose, a disaccharide containing two glucose units. Representative carbohydrates: c) A portion of a molecule of amylose, a glucose polymer found in starch. The energy cycle of life: In photosynthesis, plants use the energy of sunlight to combine carbon dioxide and water into carbohydrates, releasing oxygen in the process. In respiration, both plants and animals oxidize the carbohydrates made by plants, releasing energy and re-forming CO2 and H2O. Trioses are the simplest monosaccharides. The two triose tautomers illustrate the difference between aldose and ketose monosaccharides, also called more descriptively aldotriose and ketotriose, respectively. Carbon numbering begins in all aldoses with the aldehyde carbon and in ketoses with the end carbon closest to the ketone group. The enediol intermediate through which they are interconverted is unstable and cannot be isolated. The most compact way to represent enantiomers is to use a Fischer projection. In a Fischer projection the bonds that are drawn horizontally are imagined as coming toward you; those drawn vertically are receding. D and L forms of a monosaccharide are nonsuperimposable mirror images and are called enantiomers. The most important naturally occurring saccharides are the D-enantiomers. The enantiomers of glyceraldehyde: The configuration of groups around the chiral carbon 2 distinguishes D-glyceraldehyde from L-glyceraldehyde. The two molecules are mirror images and cannot be superimposed on one another. R–S nomenclature: The R–S system describes absolute stereochemical configuration according to a set of defined rules. Priorities for groups common in carbohydrate chemistry are: SH > OR > OH > NH2 > CO2H > CHO > CH2OH > CH3 > H We view the molecule with the group of lowest priority away from us (H in our example). If the priority of the remaining three groups decreases clockwise, the absolute configuration is called R (from Latin rectus, “right”). If priority decreases counterclockwise, the configuration is S (from Latin sinister, “left”). R–S nomenclature: In this notation, D-glyceraldehyde is R-glyceraldehyde, and L-glyceraldehyde is S-glyceraldehyde. Stereochemistry of aldotetroses: These molecules have two chiral carbons and thus have two diastereomeric forms, threose and erythrose, each with a pair of enantiomers. When monosaccharides contain more than one chiral carbon, the prefix D or L designates the configuration about the carbon farthest from the carbonyl group. Isomers differing in orientation about other carbons are called diastereomers and given different names. Stereochemical relationships of the D-aldoses: Stereochemical relationships of the D-ketoses: Monosaccharides with five or more carbons exist preferentially in five- or six-membered ring structures (Haworth projections), resulting from internal hemiacetal formation. Two anomeric forms, and are possible, a and b. An epimer at the hemiacetal carbon in a cyclic saccharide, an atom called the anomeric carbon. The anomeric carbon is the carbon derived from the carbonyl carbon compound (the ketone or aldehyde functional group) of the open-chain form of the carbohydrate molecule. Conformational isomers: These models show two of the possible ring conformations for b- D-ribofuranose. In both of them, C-1, O, and C-4 define a plane. In the C-2 endo conformation (a), C-2 is above the plane. In the C-3 endo conformation (b), C-3 is above the plane. These isomers are the two most common conformations for ribose and deoxyribose in nucleic acids. A C-3 exo conformation would look like the figure in (b), but C-3 would be flipped below the plane. The hexoses also exist primarily in ring forms under physiological conditions. As with the aldopentoses, two kinds of rings are found: five-membered furanoses and six-membered pyranoses. In each case, a and b anomers are possible. An example, illustrated by Haworth projections, follows: The four most common hexoses: These Haworth projections represent the D enantiomers. Only the b anomers are shown. Hexoses can exist in boat and chair conformations. Usually the chair is more stable. A summary of terminology describing the structure of sugar molecules: Conformational isomers are distinguished from configurational isomers in that the former can interconvert without breaking and re- forming bonds. Not shown are epimers, stereoisomers differing in their configuration about only one asymmetric carbon atom. Derivatives of the Monosaccharides Sugar phosphates are important intermediates in metabolism, functioning as activated compounds in syntheses. Oxidation of monosaccharides can proceed in several ways, depending upon the oxidizing agent used. For example, mild oxidation of an aldose with alkaline Cu(II) (Fehling’s solution) produces the aldonic acids, as in the following example: Enzyme-catalyzed oxidation of monosaccharides gives other products, including uronic acids such as glucuronic acid, in which oxidation has occurred at carbon 6. Uronic acids are, important constituents of certain natural polysaccharides. Free aldonic acids, such as gluconic acid, are in equilibrium in solution with lactones, which are cyclic esters, in this case involving the C1 carboxyl and the C5 hydroxyl. Found in the pentose-phosphate pathway. Reduction of the carbonyl group on a sugar gives rise to the class of polyhydroxy compounds called alditols. Important naturally occurring ones are erythritol, D- mannitol, and D-glucitol, often called sorbitol. Two amino derivatives of simple sugars are widely distributed in natural polysaccharides: glucosamine and galactosamine, derived from glucose and galactose, respectively. Amino sugars are found in many polysaccharides. Elimination of water between the anomeric hydroxyl of a cyclic monosaccharide and the hydroxyl group of another compound yields an O-glycoside. The acetal bond formed is referred to as a glycosidic bond. A simple example is the formation of methyl-a-D-glucopyranoside: Oligosaccharides Two naturally occurring glycosides: Ouabain and amygdalin are highly toxic glycosides produced by plants. Distinguishing Features of Different Disaccharides: Four major features distinguish disaccharides from one another: 1. The two specific sugar monomers involved, and their stereoconfigurations. The monomers may be of the same kind, as the two D-glucopyranose residues in maltose, or they may be different, as the D-glucopyranose and D-fructofuranose residues in sucrose. 2. The carbons involved in the linkage. The most common linkages are 1 1 (as in trehalose), 1 2 (as in sucrose), 1 4 (as in lactose, maltose, and cellobiose), and 1 6 (as in gentiobiose). Note that all of these disaccharides involve the anomeric hydroxyl of at least one sugar as a participant in the bond. Distinguishing Features of Different Disaccharides: 3. The order of the two monomer units, if they are different kinds. The glycosidic linkage involves the anomeric carbon on one sugar, but in most cases the other is free. The two ends of the molecule can be distinguished by their chemical reactivity. For example, the glucose residue in lactose, having a free anomeric carbon and thus a potential free aldehyde group, could be oxidized by Fehling’s solution; the galactose residue could not be. Lactose is therefore a reducing sugar, and the glucose residue is at its reducing end. The other end is called the nonreducing end. In sucrose, neither residue has a potential free aldehyde group; both anomeric carbons are involved in the glycosidic bond. Therefore, sucrose is a nonreducing sugar. Distinguishing Features of Different Disaccharides: 4. The configuration of the anomeric hydroxyl group of each residue. This feature is especially important for the anomeric carbon(s) involved in the glycosidic bond. The configuration may be either a or b. This difference may seem small, but it has a major effect on the shape of the molecule, and the difference in shape is recognized readily by enzymes. For example, different enzymes are needed to catalyze the hydrolysis of maltose and cellobiose, even though both are dimers of D-glucopyranose. To be continued… Writing the Structure of Disaccharides: A convenient way to describe the structures of these and more complex oligosaccharides has been devised. The rules are as follows: 1. The sequence is written starting with the non-reducing end at the left. 2. Anomeric and enantiomeric forms are designated by prefixes (e.g., a-, D-). 3. The ring configuration is indicated by a suffix (p for pyranose, f for furanose). 4. The atoms between which glycosidic bonds are formed are indicated by numbers in parentheses between residue designations (e.g., (1 4) means a bond from carbon 1 of the residue on the left to carbon 4 of the residue on the right). Writing the Structure of Disaccharides: For example, we can write the structure of sucrose as: a-D-Glcp(12)-b-D-Fruf Often, the D- and p or f are omitted under normal circumstances. For example, the structure of maltose can be written as: Glca(14)Glc Formation of the glycosidic bond between two monomers in an oligosaccharide is a condensation reaction, involving the elimination of a molecule of water. Thus, we might expect the synthesis of lactose to proceed as follows: Like the phosphodiester bond in nucleic acid and amide bond in proteins, the glycosidic bond is metastable. Enzymes control its hydrolysis. For glycan biosynthesis those activated monomers are usually nucleotide-linked sugars. The activated sugar molecule in lactose biosynthesis is uridine diphosphate galactose (UDP-galactose or UDPGal), a nucleotide-linked sugar formed by reaction of uridine triphosphate with galactose-1-phosphate. Glycan biosynthesis is carried out by glycosyltransferases, enzymes that transfer an activated glycosyl moiety, such as UDP-glucose, to a specific position on a carbohydrate acceptor. Enzymatic formation of lactose: The reaction shown occurs in the formation of milk in mammary tissue. Galactose is phosphorylated by ATP, then transferred to uridine diphosphate (UDP). UDP-galactose transfers galactose to glucose, with the accompanying cleavage of a phosphate bond. The reaction is catalyzed by the enzyme lactose synthase. Polysaccharides The principal storage polysaccharides are amylose and amylopectin, which together constitute starch in plants, and glycogen, which is stored in animal and microbial cells. Both starch and glycogen are stored in granules within cells Glycogen is deposited in the liver, which acts as a central energy storage organ in many animals. Glycogen is also abundant in muscle tissue, where it is more immediately available for energy release. Glycogen and the components of starch—amylose and amylopectin—are storage polysaccharides. Amylose is linear; amylopectin and glycogen are branched. Amylopectin, a branched glucan: The branches in glycogen are somewhat more frequent and shorter than those in amylopectin, and glycogen is usually of higher molecular weight, but in most respects the structures of these two polysaccharides are very similar. The secondary structure of amylose: The orientation of successive glucose residues favors helix generation. Note the large interior core. Hydrogen bonds (not shown) stabilize the helix. Cellulose structure: The b(14) linkages of cellulose generate a planar structure. The parallel cellulose chains are linked together by a network of hydrogen bonds. Cellulose and chitin are examples of structural polysaccharides. Unlike starches, which have a(14) links, these fibrous polymers have b(14) linkages. Organization of plant cell walls: Microfibrils of cellulose are embedded in a matrix of hemicellulose. Note that the fibers are laid down in a crosshatched pattern to give strength in all directions. The major structural polysaccharides in vertebrate animals are the glycosaminoglycans, formerly called mucopolysaccharides. Important examples are the chondroitin sulfates and keratan sulfates of connective tissue, the dermatan sulfates of skin, and hyaluronic acid. All are polymers of repeating disaccharide units, in which one of the sugars is either N- acetylgalactosamine or N-acetylglucosamine or one of their derivatives. All are acidic (anionic), through the presence of either sulfate or carboxylate groups. Repeating structures of some glycosaminoglycans: In each case, the repeating unit is a disaccharide, of which two are shown for each structure. A highly sulfated glycosaminoglycan is heparin. Heparin appears to be a natural anticoagulant and is found in many body tissues. It binds strongly to a blood protein, antiprothrombin III, and the complex inhibits enzymes of the blood clotting process. Therefore, heparin is used medicinally to inhibit clotting in blood vessels. The cell wall of a Gram-positive bacterium, S. aureus, consists of a thick peptidoglycan layer made up of polysaccharide chains and short peptides. The peptides are cross-linked by glycine pentapeptides. The cell wall of a Gram-negative bacterium, E. coli, has a thin peptidoglycan layer and an outer lipid membrane. The cross-links here are between tetrapeptides attached to the N-acetylmuramic acid (NAM) residues in adjacent chains The peptidoglycan layer of Gram- positive bacteria: Cross-links between the peptides are formed by pentaglycine chains between the a-amino group of the lysine (*) on one chain and the C-terminal carboxyl group of the alanine (**) on an adjacent chain. The structure of a lipotechoic acid: D-Alanyl and NAG groups are arranged irregularly on the chain, which is anchored in the membrane by lipid. Glycoproteins Oligosaccharides and proteins can be linked to form glycoproteins in two ways: o O-linked glycans are attached via threonine or serine hydroxyls. o N-linked glycans via asparagine amino groups. The ABO blood group antigens: The O oligosaccharide does not elicit antibodies in most humans. The A and B antigens are formed by addition of GalNAc or Gal, respectively, to the O oligosaccharide. Each of the A and B antigens can elicit a specific antibody. R can represent either a protein molecule or a lipid molecule. The blood group substances are a set of antigenic oligosaccharides attached to the surfaces of red cells. The structure of the influenza virus: The 13,600-nucleotide RNA genome is packaged within the sphere, about 120 nm in diameter. The spikes on the virion exterior include the hemagglutinin molecule and a spike that terminates in four neuraminidase molecules. Rational design of neuraminidase inhibitors: Structures of sialic acid, zanamivir, and oseltamivir. Partial model of the neuraminidase-zanamivir complex, showing amino acid residues that are close to the binding site for the inhibitor. Oligosaccharides as Cell Markers Some animal cells have an extremely thick coating of polysaccharides called a glycocalyx (literally “sugar coat”). Glycocalyx oligosaccharides interact with other substances: o With bacteria in the intestine. o With collagen of the intercellular matrix in some other tissues. Imaging cell surface glycoproteins in living cells: Zebrafish embryos were allowed to incorporate anazido derivative of GalNAc. At fixed intervals the embryos were treated with a fluorescent reagent that couples to the azido group in the incorporated analog. Biosynthesis of Glycoconjugates—Amino Sugars Biosynthesis of UDP-N- acetylglucosamine from glucosamine-6-phosphate: Biosynthesis of N-acetylneuraminic acid (sialic acid) from UDP-N- acetylglucosamine: Structures of the major types of asparagine-linked (N-linked) oligosaccharides: The red boxes contain the pentasaccharide core common to all known N-linked structures. Glycoconjugates of Interest Biosynthesis of the lipid-linked oligosaccharide intermediate: The five sequential mannosyl transfer reactions from GDP-mannose are catalyzed by separate glycosyltransferases, as are the four mannosyl transfers from dolichol phosphate mannose. The latter is synthesized in turn from GDP- mannose. The acceptor site on the polypeptide chain is an asparagine residue two positions to the N side of a serine or threonine. The whole process occurs in the endoplasmic reticulum, with translocation to the lumen of the ER occurring after transfer of the fifth mannose residue. Schematic pathway of oligosaccharide processing on newly synthesized glycoproteins: Synthesis of the linear peptidoglycan molecule of S. aureus: The peptidoglycan molecule is synthesized by addition of N-acetylglucosamine and five glycyl residues to N- acetylmuramylpentapeptide, with undecaprenol phosphate acting as carrier. Sites of inhibition by the antibiotics bacitracin and vancomycin are identified. Following synthesis, the peptidoglycan is transported through the cell membrane to the cell wall and added to the end of a chain in the peptidoglycan layer. The cross-linking reaction in peptidoglycan synthesis (left) and inhibition of the transpeptidase enzyme, E, by penicillin (right): Cross-links between adjacent peptidoglycan chains are formed by the action of a transpeptidase enzyme, as shown at the left. At the right is shown how penicillin, a structural analog of the natural substrate, reacts with the active form of the enzyme to form an inactive covalent complex that resembles the enzyme–substrate complex. Biosynthesis of the repeating oligosaccharide unit of the O antigen of Salmonella typhimurium: The first four reactions occur within the inner membrane. Transfer of the activated tetrasaccharide unit to the unactivated terminus of a growing polysaccharide unit occurs on the outside of the outer membrane.