Chemistry - Unit 5 R23 Past Paper PDF
Document Details
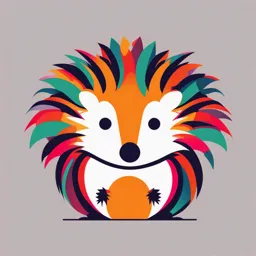
Uploaded by TopnotchSodium6871
Tags
Summary
This document contains lecture notes on instrumental methods and applications in chemistry, focusing on various spectroscopy techniques like UV-visible and IR spectroscopy, and chromatographic methods like HPLC. The document also includes information on the principle, instrumentation, and applications of these techniques, as well as examples.
Full Transcript
UNIT V: INSTRUMENTAL METHODS AND APPLICATIONS (9 hours) (a) Electromagnetic spectrum. (1 hr) (b) Absorption of radiation: Beer-Lambert’s law. UV-Visible Spectroscopy, electronic transition, Instrumentation, (2 hr) (c) IR spectroscopy, fundamental modes and selection rules, Instrum...
UNIT V: INSTRUMENTAL METHODS AND APPLICATIONS (9 hours) (a) Electromagnetic spectrum. (1 hr) (b) Absorption of radiation: Beer-Lambert’s law. UV-Visible Spectroscopy, electronic transition, Instrumentation, (2 hr) (c) IR spectroscopy, fundamental modes and selection rules, Instrumentation. (3 hr) (d) Chromatography-Basic Principle, Classification (1 hr) (e) HPLC: Principle, Instrumentation and Applications. (2 hr) 1 Electromagnetic Radiation 2 Regions of Electromagnetic Radiation Frequency () Wavelength () Radio-waves Microwaves Infra-red Visible Ultra-violet X-ray -ray Region Region Region Region Region Region Region Frequency 106 - 1010 1010 - 1012 1012 - 1014 1014 - 1015 1015 - 1016 1016 - 1018 1018- 1020 (HZ) Wavelength 10m – 1 cm 1 cm – 100µm 100µm – 1µm 700 – 400 nm 400-10 nm 10nm – 100pm – 100pm 1 pm NMR, ESR Rotational Vibrational Electronic Electronic Spectroscopy spectroscopy Spectroscopy Spec. Energy 0.001 – 10 Order of some Some 104 Some 100 Some 100s 107- 109 109- 1011 J/mole 100 J/mole J/mole kJ/mole kJ/mole J/mole J/mole UHF TV, cellular, Table lamp, telephones. Tube light (400 (300 MHz and 3 GHz) nm -800 nm or 400–790 THz) FM Radio, VHF TV. Microwave ovens, Sun Lamp X-ray AM Radio Police radar, satellite 3 stations-- (3 to 30 GHz) THE ELECTROMAGNETIC SPECTRUM 4 Energy is quantized Planck suggests that radiation (light, energy) can only come in quantized packets that are of size hν. Planck’s constant Planck, 1900 Energy (J) E h h = 6.626 × 10-34 J·s Frequency (s-1) Note that we can specify the energy by specifying any one of the following: 1. The frequency, n (units: Hz or s-1): E h 2. The wavelength, λ, (units: m or cm or mm): hc Recall: c E 3. The wavenumber, ~ (units: cm-1 or m-1) 1 E hc~ Recall: ~ 5 The interaction of radiation with matter 6 http://hyperphysics.phy-astr.gsu.edu/hbase/mod3.html WHAT IS SPECTROSCOPY? Atoms and molecules interact with electromagnetic radiation (EMR) in a wide variety of ways. Atoms and molecules may absorb and/or emit EMR. Absorption of EMR stimulates different types of motion in atoms and/or molecules. The patterns of absorption (wavelengths absorbed and to what extent) and/or emission (wavelengths emitted and their respective intensities) are called ‘spectra’. Spectroscopy is the study of the interaction of light with matter. The fundamental measurement obtained in spectroscopy is a spectrum, which is a plot of measured light intensity versus some property of light. 7 UV-Vis spectroscopy Electronic absorption spectroscopy 8 UV-Visible Spectroscopy THE BEER- LAMBERT LAW I I T t %T t x 100 For a light absorbing Io Io Io medium, the light light intensity (I) intensity falls exponentially with Io It sample depth. For a light absorbing l medium, the light intensity falls It cuvette exponentially with increasing sample concentration. l Sample depth 9 UV-Visible Spectroscopy cl AA cl log AA log1010TT Absorbance The negative logarithm of T is called the absorbance (A) and this is directly proportional to sample depth (called pathlength, l) and sample concentration (c). The equation is called the Beer-Lambert law. is called the molar absorption coefficient and has units of dm3 Concentration mol-1 cm-1 10 11 UV-Visible Spectroscopy-Basics Ultraviolet radiation stimulates electronic transitions. Absorption spectroscopy from 160 nm to 780 nm Measurement of absorption or transmittance Identification of inorganic and organic species 12 UV-Visible Spectroscopy-Principle Electronic transitions – Molecular Orbital Theory In order to absorb light in the region from 200 - 800 nm (which is where the spectra are measured), the molecule must contain either pi bonds or atoms with non-bonding orbitals. A non-bonding orbital is a lone pair on, say, oxygen, nitrogen or a halogen. Molecules or parts of molecules that absorb light strongly in the UV-vis region are called chromophores. 13 Electronic Transitions-Conjugated π Systems If the molecule is exposed to light of a wavelength with energy equal to ΔE, the HOMO-LUMO energy gap, this wavelength will be absorbed and the energy used to excite one of the electrons from the HOMO to the LUMO. This is referred to as a electronic transition. For example, in H2 molecule, the ΔE for this electronic transition corresponds to the light with a wavelength of 111 nm. When a double-bonded molecule such as ethylene absorbs light, it undergoes a π - π* transition. Because π- π* energy gaps are narrower than σ - σ* gaps, ethylene absorbs light at 165 nm - a longer wavelength than molecular hydrogen. 14 Electronic Transitions-Conjugated π Systems The electronic transitions of both molecular hydrogen and ethylene are too energetic to be accurately recorded by a standard UV spectrophotometers. Usually, a UV-Visible spectrophotometer can record the spectra ranging approximately between 220 – 800 nm. UV-vis spectroscopy is a powerful tool for most organic and biological chemists is in the study of molecules with conjugated π systems. In these groups, the energy gap for π -π* transitions is smaller than for isolated double bonds, and thus the wavelength absorbed is longer. 15 Electronic Transitions-Conjugated π 1,3-butadiene is an example of conjugated Systems double bond system absorbs UV light with a wavelength of 217 nm. As conjugated pi systems become larger, the energy gap for a π - π* transition becomes increasingly narrow, and the wavelength of light absorbed correspondingly becomes longer. The absorbance due to the π - π* transition in 1,3,5-hexatriene occurs at 258 nm, corresponding to a ΔE of 111 kcal/mol. In molecules with extended pi systems, the HOMO-LUMO energy gap becomes so small that absorption occurs in the visible rather then the UV region of the electromagnetic spectrum. The conjugated pi system in 4-methyl-3- penten-2-one gives rise to a strong UV absorbance at 236 nm due to a π - π* transition. However, this molecule also absorbs at 314 nm. This second absorbance is due to the transition of a non-bonding (lone pair) electron on the oxygen up to a π* anti-bonding MO. This is 4-Methyl-3-Penten-2-One referred to as an n - π* transition. 16 The nonbonding (n) MO’s are higher in energy than the highest bonding p orbitals, so the energy gap for an n→π∗ transition is smaller that that of a π - π* transition – and thus the n - π* peak is at a longer wavelength. In general, n - π* transitions are weaker (less light absorbed) than those due to π - π* transitions. Molecule Wavelength at ∆E Electronic Transitions Maximum Absorbance (Kcal/Mol) (λMax) Hydrogen 111nm (σ→σ*) 258 Ethylene 165nm (π→π*) 173 1,3-Butadiene 217nm ((π→π*) 132 1,3,5-Hexatriene 258nm (π→π*) 111 4,methyl-3-penten-2-one 236nm (π→π*) 121 314nm (n→π*) 91 β-carotene 470nm (π→π*) ? Erythrosine (Red # 3) 524nm (π→π*) ? 17 Indigotine (Blue #2) 630nm (π→π*) ? UV-Visible Spectroscopy of β- Carotene β-Carotene Beta-carotene, with its system of 11 conjugated double bonds, absorbs light with wavelengths in the blue region of the visible spectrum while allowing other visible wavelengths – mainly those in the red-yellow region - to be transmitted. This is why carrots or papaya are orange. 18 Instrumentation The instrumentation of UV-Visible spectroscopy is called a UV-Visible spectrophotometer. The spectrophotometer has a few key components, namely: The light source emits broadband electromagnetic radiation across the UV-visible spectrum. The dispersion device or monochromator - it’s a diffraction grating that separates the radiation into its component wavelength. A sample area - the radiation interacts with the sample as it passes through or reflects off. Detector - measures the reflected or transmitted radiation intensity. 19 Applications of UV-Visible Spectroscopy 1. To study kinetics of a reaction: rate of reaction, rate equation and mechanism of a reaction. 2. To study enzyme kinetics: In clinical diagnostics for the estimation of Glucose, Tissue damage 3. It is used for dissolution testing of tablets and products in the pharmaceutical industry. 4. In-vitro and In-vivo drug release kinetics can also be studied. 5. In environmental and agricultural fields the quantification of organic materials and heavy metals in fresh water can be carried out using UV-visible spectroscopy. 6. In the dye, ink and paint industries UV-visible spectroscopy is used in the quality control in the development and production of dyeing reagents, inks and paints and the analysis of intermediate dyeing reagents. 7. In the biochemical and genetic fields UV-visible spectroscopy is used in the quantification of DNA and protein/enzyme activity as well as the thermal denaturation of DNA. 20 Infrared Spectroscopy IR spectra produced by absorption of energy by a molecule in the infrared region and the transition occur between vibrational levels. This is also known as vibrational spectroscopy. An IR spectrum is a plot of per cent transmittance (or absorbance) against wave-number (frequency or wavelength). A 100 per cent transmittance in the spectrum implies no absorption of IR radiation. When a compound absorbs IR radiation, the intensity of transmitted radiation decreases. This results in a decrease of percent transmittance and hence a dip in the spectrum. The dip is often called an absorption peak or absorption band. Different types of groups of atoms (C-H, O-H, N-H, etc…) absorb infrared radiation at different characteristic wave numbers. 21 22 23 IR Spectroscopy - Principles A molecule can only absorb radiation when the incoming infrared radiation is of the same frequency as one of the fundamental modes of vibration of the molecule. A molecule to absorb infrared radiations it must possess a specific feature, i.e. an electric dipole moment of the molecule must change during the vibration. This is the selection rule for infrared spectroscopy. The dipole moment of a hetero nuclear diatomic molecule changes as the bond expands and contracts. By comparison, an example of an ‘infrared- inactive’ molecule is a homonuclear diatomic molecule because its dipole moment remains zero no matter how long the bond. 24 IR Spectroscopy - Principles The interactions of infrared radiation with matter may be understood in terms of changes in molecular dipoles associated with vibrations and rotations. In order to begin with a basic model, a molecule can be looked upon as a system of masses joined by bonds with spring-like properties. Taking first the simple case of diatomic molecules, such molecules have three degrees of translational freedom and two degrees of rotational freedom. The atoms in the molecules can also move relative to one other, that is, bond lengths can vary or one atom can move out of its present plane. This is a description of stretching and bending movements that are collectively referred to as vibrations. 25 Molecular Vibrations Stretching vibration Distance between two atom decrease or increase, but bond angle unaltered. Symmetrical Asymmetrical stretching stretching Bending or deformation vibration Bond angle decrease or increase, but bond distance remain same. 26 Twisting Wagging Scissoring Rocking Molecular vibrations For non – linear molecule non – linear molecule containing “n” atoms has (3n-6) fundamental vibration modes. Eg: H2O– (3 x 3 – 6) = 3 fundamental vibration modes. 1. Symmetric stretching, 2. Asymmetric stretching, 3. Bending stretching For linear molecule linear molecule containing “n” atoms has (3n-5) fundamental vibration modes. Eg: CO2 – (3 x 3 – 5) = 4 fundamental vibration modes. 1. Symmetric stretching, 2. Asymmetric stretching, 3. In-plane bending (scissoring, rocking) 27 4. Out of plane bending (twisting, waging) Principle of IR Spectroscopy Hooke’s Law For every bond, the wavenumber of absorption associated with bond stretching is dependent on two factors: (1) bond strength and (2) masses of the atoms sharing the bond. The impact of these two factors can be rationalized when we treat a bond as if it were a vibrating spring connecting two weights. Using vibrating spring analogy following equation is constructed derived from Hooke’s law, which enables us to approximate the frequency of vibration for a bond between two atoms of mass m1 and m2: and The equation shows that the frequency of the vibration n is proportional to the (root of) a force constant k—more or less the bond strength—and inversely proportional to the (root of) a reduced mass μred, that is, the product of the masses of the two atoms forming the bond divided by their sum. It is evident from the above equations that stronger 28 bonds vibrate faster and so do lighter atoms. Principle of IR Spectroscopy For each and every bond in a molecule, the energy gap between vibrational states is very much dependent on the nature of the bond. For example, the energy gap for a C−H bond is much larger than the energy gap for a C−O bond. Both bonds will absorb IR radiation but the C−H bond will absorb a higher energy photon. 29 IR Absorption Band Positions Use of the reduced mass in this equation allows us to treat the two atoms as one system. Since that μred appears in the denominator the smaller atoms give bonds that vibrate at higher frequencies, thereby corresponding to a higher wavenumber of absorption. A comparison of absorption wavenumbers for different groups where different atoms bonded with carbon is given. The C−H bond involves the smallest atom (H) and therefore appears at the highest wavenumber. C-H C-D C-O C-Cl 3000cm-1 2200cm-1 1100cm-1 700cm-1 While the force constant (k) which is a measure of bond strength appears in the numerator. This means that stronger bonds will vibrate at higher frequencies, thereby corresponding to a higher wavenumber of absorption. For example, compare the following bonds. The C≡N bond is the strongest of the three bonds and therefore appears at the highest wavenumber. 2200cm-1 1600cm-1 1100cm-1 30 Types of bands in IR spectra 31 INFRARED SPECTROSCOPY Ethane Chloroethane 32 Instrumentation The instrumentation of IR spectroscopy is called a IR spectrophotometer. 33 Instrumentation The instrumentation of IR spectroscopy is called a IR spectrophotometer. Light source Two common sources used as a light source are Nernst filament and Globar which are heated to more than 1000°C for this purpose. Monochromators The IR beam enters into the monochromator which is dispersed by grating or prism. The resulting beam is separated into single wavelengths. Detectors After the dispersion of the IR beam, it is passed through the sample. Each particular wavelength that is being transmitted is amplified. These wavelengths are then compared to the reference beam. Then the difference is represented on the digital graph. 34 Application Identity of the compound Resemblance of two spectra gives the nature of the compound. Eg: C=O in aldehyde and ketone Detection of functional group C=O in Acetone and diethyl ketone give absorption peak in same place But C=O in acetic acid will show peak in different place. Testing purity of the sample Pure sample give sharp and well resolved bands But impure samplegive broad and poor resolved bands 35 Application Study progress of chemical reaction IR spectrum of test solution at different time interval give the progress of the chemical reaction Determination of shape or symmetry of the molecule Linear or non linear nature can be determined Eg; NO2 gives 3 peaks at 750, 1323, 1616 cm-1 For non linear molecule = (3n – 6) = 3 peaks, so NO2 is non-linear To study tautomerism These shows the IR band for C=O, - OH, and - NH HN CH2 HC NH N CH2 C C C O OH OH 36 Application Industrial applications a. Determination of structure of chemical compounds b. Determination of molecular weight c. Crystallinity Eg; Crystalline Nylon 6,6 – at 943 cm-1 Amorphous Nylon 6,6 – at 1238 cm-1 Determination of isomers Isomers show similar spectra in group frequency region, but show different peaks in finger print region Determination of hydrogen bonding Inter and intra molecular hydrogen bonding determined using solutions of different dilution 37 Chromatography Chromatography is an important biophysical technique that enables the separation, identification, and purification of the components of a mixture for qualitative and quantitative analysis. A wide range of chromatographic procedures makes use of differences in size, binding affinities, charge, and other properties to separate materials. It is a powerful separation tool that is used in all branches of science and is often the only means of 38 separating Chromatography 39 Important Terms in Chromatography Term Definition Mobile phase or carrier solvent moving through the column Stationary phase or adsorbent substance that stays fixed inside the column Eluent fluid entering the column fluid exiting the column (that is collected in Eluate flasks) the process of washing out a compound Elution through a column using a suitable solvent mixture whose individual components have to Analyte be separated and analyzed 40 Principle of Chromatography Chromatography is based on the principle where molecules in mixture applied onto the surface or into the solid, and fluid stationary phase (stable phase) is separating from each other while moving with the aid of a mobile phase. The factors effective on this separation process include molecular characteristics related to adsorption (liquid- solid), partition (liquid-solid), and affinity or differences among their molecular weights. Because of these differences, some components of the mixture stay longer in the stationary phase, and they move slowly in the chromatography system, while others pass rapidly into the mobile phase, and leave the system faster. 41 Principle of Chromatography Three components thus form the basis of the chromatography technique. 1. Stationary phase: This phase is always composed of a “solid” phase or “a layer of a liquid adsorbed on the surface solid support”. 2. Mobile phase: This phase is always composed of “liquid” or a “gaseous component.” 3. Separated molecules The type of interaction between the stationary phase, mobile phase, and substances contained in the mixture is the basic component effective on the separation of molecules from each other. 42 Principle of Chromatography 43 Types of Chromatography Substances can be separated on the basis of a variety of methods and the presence of characteristics such as size and shape, total charge, hydrophobic groups present on the surface, and binding capacity with the stationary phase. This leads to different types of chromatography techniques, each with their own instrumentation and working principle. For instance, four separation techniques based on molecular characteristics and interaction type use mechanisms of ion exchange, surface adsorption, partition, and size exclusion. Other chromatography techniques are based on the 44 Types of Chromatography Commonly employed chromatography techniques include: 1. Column chromatography 2. Ion-exchange chromatography 3. Gel-permeation (molecular sieve) chromatography 4. Affinity chromatography 5. Paper chromatography 6. Thin-layer chromatography 7. Gas chromatography (GS) 8. Dye-ligand chromatography 9. Hydrophobic interaction chromatography 10.Pseudoaffinity chromatography 11.High-performance liquid chromatography (HPLC) 45 High-performance liquid chromatography (HPLC) Principle of HPLC This technique is based on the principle of differential adsorption where different molecules in a mixture have a varying degree of interactions with the absorbent present on the stationary phase. The molecules having higher affinity remain adsorbed for a longer time decreasing their speed of movement through the column. However, the molecules with lower affinity move with a faster movement, thus allowing the molecules to be separated in different fractions. This process is slightly different from the column chromatography as in this case; the solvent is forced under high pressures of up to 400 atmospheres instead of allowing it to drip down under gravity. 46 Principle of HPLC Steps of HPLC i. The column is prepared by taking a glass tube that is dried and coated with a thin, uniform layer of stationary phase (cellulose, silica). ii. Then the sample is prepared by adding the mixture to the mobile phase. The sample is introduced into the column from the top, and a high-pressure pump is used to pass the sample at a constant rate. iii. The mobile phase then moves down to a detector that detects molecules at a certain absorbance wavelength. iv. The separated molecules can further be analyzed for various purposes. 47 Instrumentation 48 Instrumentation The Pump The pump is positioned in the most upper stream of the liquid chromatography system and generates a flow of eluent from the solvent reservoir into the system. High-pressure generation is a “standard” requirement of pumps besides which, it should also to be able to provide a consistent pressure at any condition and a controllable and reproducible flow rate. Most pumps used in current LC systems generate the flow by back-and-forth motion of a motor-driven piston (reciprocating pumps). Because of this piston motion, it produces “pulses”. Injector An injector is placed next to the pump. The simplest method is to use a syringe, and the sample is introduced to the flow of eluent. The most widely used injection method is based on sampling loops. The use of the autosampler (auto-injector) system is also widely used that allows repeated injections in a set scheduled-timing. 49 Instrumentation Column The separation is performed inside the column. The recent columns are often prepared in a stainless steel housing, instead of glass columns. The packing material generally used is silica or polymer gels compared to calcium carbonate. The eluent used for LC varies from acidic to basic solvents. Detector Separation of analytes is performed inside the column, whereas a detector is used to observe the obtained separation. The composition of the eluent is consistent when no analyte is present. While the presence of analyte changes the composition of the eluent. What detector does is to measure these differences. This difference is monitored as a form of an electronic signal. 50 Instrumentation Recorder The change in eluent detected by a detector is in the form of an electronic signal, and thus it is still not visible to our eyes. In older days, the pen (paper)-chart recorder was popularly used. Nowadays, a computer-based data processor (integrator) is more common. There are various types of data processors; from a simple system consisting of the in-built printer and word processor while those with software that are specifically designed for an LC system which not only data acquisition but features like peak-fitting, baseline correction, automatic concentration calculation, molecular weight determination, etc. Degasser The eluent used for LC analysis may contain gases such as oxygen that are non-visible to our eyes. When gas is present in the eluent, this is detected as noise and causes an unstable baseline. Degasser uses special polymer membrane tubing to remove gases. The numerous very small pores on the surface of the polymer tube allow the air to go through while preventing any liquid to go through the pore. 51 Applications Pharmaceutical sector To identify and analyze samples for the presence of trace elements or chemicals. Separation of compounds based on their molecular weight and element composition. Detects the unknown compounds and purity of mixture. In drug development. Chemical industry In testing water samples and also checks air quality. HPLC is very much used for detecting various contaminants such as polychlorinated biphenyl (PCBs) in pesticides and oils. In various life sciences applications 52 Applications Food Industry In food spoilage and additive detection Determining the nutritional quality of food Forensic Science In forensic pathology and crime scene testing like analyzing blood and hair samples of crime place. Molecular Biology Studies In the study of metabolomics and proteomics along with nucleic acid research. HPLC is used in Protein Separation like Insulin Purification, Plasma Fractionation, and Enzyme Purification and also in various departments like Fuel Industry, biotechnology, and biochemical processes. 53 Thank you! 54