Chemistry Unit 1 Notes PDF
Document Details
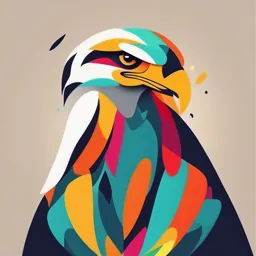
Uploaded by CleanlyLosAngeles
K.K. Wagh Institute Of Engineering Education and Research
Tags
Summary
These notes cover the fundamentals of electrochemical cells, batteries, and electroanalytical techniques in applied chemistry. Different types of cells and batteries, and their characteristics, are described, providing an overview of the subject.
Full Transcript
K.K.Wagh Institute of Engineering Education and Research, Nasik Subject: Applied Chemistry Unit I: Cells, Batteries and Electro analytical Techniques 1.1: Introduction Electrochemical cell is a system in which two ele...
K.K.Wagh Institute of Engineering Education and Research, Nasik Subject: Applied Chemistry Unit I: Cells, Batteries and Electro analytical Techniques 1.1: Introduction Electrochemical cell is a system in which two electrodes are fitted in the same electrolyte or in two different electrolytes which are joined by a salt bridge. Electrochemical cells are of two types: a) Electrolytic cell b) Galvanic or voltaic cell Electrolytic cell is a device in which electrolysis is carried out by using electricity or in which conversion of electrical energy into chemical energy. Galvanic or voltaic cell is a device in which a redox reaction is used to convert chemical energy into electrical energy. Representation of an Electrochemical cell (Galvanic cell): Universally accepted conventions for representing an electrochemical cell are as follows- i. The anode (-ve electrode) is written on the left hand side and cathode (+ve electrode) is written on the right hand side. ii. A vertical line (|) or semicolon (;) indicates a contact between two phases. The anode of the cell is represented by writing metal first and then the metal ion present in the electrolytic solution. For example, Zn|Zn2+ OR Zn;Zn2+ The molar concentration of the solution is written in brackets after the formula of the ion. Zn|Zn2+ (1M) OR Zn|Zn2+ (0.1M) iii. The cathode of the cell is represented by writing the cation of the electrolyte first and then metal, both are separated by a vertical line or semicolon. For example, Cu2+| Cu OR Cu2+;Cu OR Cu2+ (1M) | Cu iv. The salt bridge which separates the two half cells is indicated by two parallel vertical lines. v. Sometimes negative and positive signs are also put on the electrodes. The Daniell cell can be represented as: Zn | ZnSO4 (aq.) || CuSO4 (aq.) | Cu OR Zn | Zn2+|| Cu2+ | Cu OR Zn |Zn2+ (1M) || Cu2+ (1M) | Cu 1.2: Battery 1.2.1: State the Unique Difference between a Cell and a Battery Cell: A cell is a source of energy that generates only DC voltage and current of small magnitude. For example, the cells used in remote control or wristwatches can generate voltage within the 1.5 - 3 V range. Battery: The function of a battery and a cell is similar, but a battery contains several cells arranged in either series or parallel to produce a voltage of desired levels. A power bank is the best example of a battery; it is used to charge mobile phones. Any battery (actually it may have one or more than one cell connected in series) or cell that we use as a source of electrical energy is basically a galvanic cell where the chemical energy of the redox reaction is converted into electrical energy. However, for a battery to be of practical use it should be reasonably light, compact and its voltage should not vary appreciably during its use. There are mainly two types of batteries. 1.2.2: Primary and Secondary batteries. Primary batteries: Primary cells are the cells which convert chemical energy into electrical energy till the chemical components are active, once the active chemical components are used up, they cannot be recharged or reused”. For example: Dry cell or Leclanche cell, Daniel cell. Secondary batteries: These are rechargeable cells, the redox reaction converts chemical energy into electrical energy which can be reversed by passage of electric current. The electrical energy is stored in the form of chemical energy hence they are also called as storage cells. During discharge these cells behave as galvanic/electrochemical cell and during recharge as electrolytic cell. For example: Lead acid storage battery, Ni-Cd battery. Comparison between primary and secondary batteries Sr. No. Primary batteries Secondary batteries 1 Primary cells are non-rechargeable Secondary cells are rechargeable cells. cells. 2 The cell reaction is irreversible. The cell reaction is reversible. 3 Once the active components are used Electrical energy is stored in the up, they cannot be reused. form of chemical energy hence they are also called as storage cells. 4 They have short life. They have long life. 5 Its cost is low. Its cost is high 6 For example: Dry cell For example: Ni-Cd cell 1.3 Classical Batteries 1.3.1: Dry cell or Leclanche cell: This is a primary battery, popularly known as dry cell. It consists of a graphite rod (with a metal cap) at the center, which acts as the cathode of the cell. The cathode is surrounded by a thick moist paste of graphite and manganese dioxide (MnO2). The outer layer consists of a paste of ammonium chloride (NH4Cl) and zinc chloride (ZnCl2). The entire assembly is placed inside a thin zinc cylinder container, which acts as anode of the cell as shown in fig.3. The whole assembly is wrapped with polypropylene or cardboard materials, which prevents leakage, if any. Anode: Zn(s) Zn2+ + 2e– Cathode: 2MnO2 + 2NH4+ + 2e– Mn2O3+ 2NH3 + H2O Overall Reaction: Zn + 2MnO2 + 2NH4+ Zn2+ + Mn2O3+ 2NH3 + H2O [ Zn2+ + 4NH3 [Zn(NH3)4]2+ ( Formation of a complex ) ] Fig.1: A commercial dry cell In the reaction at cathode, manganese is reduced from the + 4 oxidation state to the +3 state to form Mn2O3. Ammonia produced in the reaction has an explosive nature. To avoid this explosion ammonia forms a complex with Zn2+ to give [Zn (NH3)4]Cl2. These processes disrupt the flow of current. The cell has a potential of nearly 1.5 V and the voltage decreases with usage due to accumulation of products at the electrodes. Even when the cell is not in use, the lifetime of the dry cell is not indefinite because acidic NH4Cl corrodes the zinc cathode. Dry cells are most commonly used batteries that find use in flash lights, radios, tape recorders, transistors, etc. 1.3.2: Alkaline battery An alkaline battery in which alkali is used as electrolytic solution.It is a type of primary battery that derives its energy from the reaction between zinc metal and manganese dioxide. The alkaline battery gets its name because it has an alkaline electrolyte of potassium hydroxide (KOH) instead of the acidic ammonium chloride (NH4Cl) or zinc chloride (ZnCl2) electrolyte of the zinc–carbon batteries. Other battery systems also use alkaline electrolytes, but they use different active materials for the electrodes. Compared with zinc–carbon batteries of the Leclanche cell or zinc chloride types, alkaline batteries have a higher energy density and longer shelf life, yet provide the same voltage. In an alkaline battery, the negative electrode is zinc and the positive electrode is manganese dioxide (MnO2). The alkaline electrolyte of potassium hydroxide is not consumed during the reaction, only the zinc and MnO2 are consumed during discharge. The alkaline electrolyte of potassium hydroxide remains, as there are equal amounts of OH− consumed and produced. The half-reactions are: At Anode: Zn(s) + 2OH−(aq) Zn(OH)2(s) + 2e− [Eoxidation° = +1.28V] At Cathode: 2MnO2(s) + H2O (l) + 2e− Mn2 O3(s) + 2OH−(aq) [Ereduction°= +0.15V] Overall reaction : Zn(s) + 2MnO2(s) + H2O ⇌ Zn(OH)2(s) + Mn2O3(s) [e° = +1.43 V] Alkaline batteries are used in many household items such as MP3 players, CD players, digital cameras, toys, flashlights, and radios. Fig. 2 Alkaline Battery 1.4: Secondary Batteries 1.4.1 Ni-Cd Battery: Construction 1. The nickel–cadmium battery (Ni–Cd battery or NiCad battery) is a type of rechargeable battery using nickel oxide hydroxide and metallic cadmium as electrodes, a separator is made of a thin non –woven fabric , an alkaline electrolyte and a metal case a sealing plate provided with a self –sealing safety valve. 2. In the Ni-Cd cell a cell cup made up steel coated with nickel acts as a cathode and a cell cap in contact with the anode of the cell is at the centre and anodic. 3. Anode composition: The composition of the active materials of the anode are spongy Cd with 78% Cd(OH)2, 18 % Fe, 1 % Ni and 1 % graphite which are pressed into a tablet form and wrapped in nickel wire gauge. 4. Cathode Composition: The outer cap contains a mixture of 80% NiOOH and Ni (OH) 2, 2% Co (OH) 2, 18% graphite and very minute quantities of barium compounds to increase the efficiency of the active materials and also the cycle life. 5. The two electrodes are separated by absorbent material soaked with 6M KOH as electrolyte. An insulated washer at the top separates the anode and the cathode compartments without any contact. 6. The discharging and charging reactions of Ni-Cd cell are reversible. Reaction: Reactions during discharging: At Anode: Cd (s) + 2OH- Cd(OH)2 + 2e- At Cathode: 2 NiO(OH) + 2H2O+ 2e- 2Ni(OH)2 + 2OH- Overall Cell Reaction : Cd (s) + 2 NiO(OH) + 2H2O Cd(OH)2 + 2Ni(OH)2 Reactions during charging: At Anode: 2Ni(OH)2 + 2OH- 2 NiO(OH) + 2H2O +2e At Cathode: Cd(OH)2 + 2e- Cd (s) + 2OH- Overall Cell Reaction: Cd(OH)2 + 2Ni(OH)2 Cd (s) + 2 NiO(OH) + 2H2O Fig.3: Ni-Cd battery Advantages 1) It is very reliable, sturdy and his long life battery, which can be operated effectively at high discharge rates and a wide temperature range. 2) It has good retention properties, and it can be stored for long periods of life in any condition without deterioration. 3) Little maintenance is needed for this battery. Applications 1. Ni-Cd batteries are used in photography, phones, computers, transmitters, hearing aids, emergency lights etc. 2. Vented Ni-Cd batteries also find applications in railways, for marine duties, aircraft and traction batteries. 1.4.2 Lithium ion battery A group of battery systems known as lithium – ion rocking chair, or swing batteries are receiving great attention and interest recently. In lithium ion batteries lithium compounds are used as anode. These batteries are rechargeable batteries. Lithium battery consists of an anode of lithium, dissolved as ions, into a carbon. The cathode material is made up from lithium liberating compounds, typically the three electro-active oxide materials like LiCoO2 (Lithium Cobalt oxide), LiMnO2 (Lithium Manganese oxide) or LiNiO2 (Lithium Nickel oxide). Construction of Lithium ion battery Li- ion cell has four layer structures. Cathode: A positive electrode made with Lithium Cobalt Oxide has a current collector made of thin aluminium foil. Anode: A negative electrode made with specialty carbon has a current collector of thin copper foil. A separator is a fine porous polymer film. An electrolyte made with lithium salt in an organic solvent. The electrolytes are selected in such a way that there should be an effective transport of Li-ion to the cathode during discharge. The type of conductivity of electrolyte is ionic in nature rather than electronic. Working of Lithium ion battery Reactions during discharging: During discharging, LiC6 shows dissociation to form Li+ ions. These Li+ ions thus migrate though the electrolyte towards the cathode to combine with CoO 2 to form LiCoO2. The chemical reaction that takes place inside the battery is as follows, At Anode: LiC6 Li+ + C6 + e- At Cathode: CoO2 + Li+ + e- LiCoO2 LiC6 + CoO2 LiCoO2 + C6 Reactions during Charging: During charging, lithium is present in combine form i.e. LiCoO2 at positive electrode is ionized to form Li+ ions. These Li+ ions then pass through the electrolyte towards the anode to form an intercalation compound i.e Lithium carbide. At Anode: LiCoO2 CoO2 + Li+ + e- At Cathode: Li+ + C6 + e- LiC6 LiCoO2 + C6 LiC6 + CoO2 The lithium ion is inserted and exerted into the lattice structure of anode and cathode during charging and discharging. During discharge current flows through the external circuit and bulb glows. During charging now the electrons flow in the opposite direction. The process is completely reversible. Thus the lithium ions pass back and forth between the electrodes during charging and discharging. Because of this reason, the lithium ion batteries are called as ‘rocking chair’ or ‘swing cells.’ The charged open circuit voltage of the cell is about 4.1 V. The energy density is about 250 wh/L while the specific energy is 115 wh/Kg. The cycle life is quoted at 1200 cycles with reasonably high current capability and a self-discharge rate of 10% per month. The unit cells are cylindrical, jelly roll designs, generally of the same as Ni-Cd cells. The batteries were used for portable telephones, computers and camcorders. These battery systems are under further development, particularly for use in electric vehicles and for energy storage services. Fig.4: Li-ion battery Advantages of Li-ion battery It has high energy density than other rechargeable batteries. Low weight It produces high voltage out about 4 V as compared with other batteries. They have improved safety, i.e. more resistance to overcharge. No liquid electrolyte means they are immune from leaking. Fast charge and discharge rate. Disadvantages of Li-ion battery They are expensive. They are not available in standard cell types. Applications of Li-ion battery Li-ion batteries are used in cameras, calculators, smart phones and most of the consumer electronics devices. They are used in cardiac pacemakers and other implantable device. Electric vehicle 1.5 FUEL CELLS Definition Fuel cell or flow battery is the device in which a fuel gets oxidized at anode by the oxidant O2 from cathode, converting the chemical energy of the indirect combustion into electrical energy. The fuels usable in fuel cell are gases like H2, LPG, CNG, NG and liquid like methyl alcohol on anode and O2 at cathode. Fuel | electrode | electrolyte | electrode |O2 Introduction The reactants are fed continuously and separately to the electrodes, separated by an ion conducting barrier. Catalytic oxidation of fuel occurs at anode and intermediate product formed diffuses to cathode through ion conducting medium to react with O2 to form the product of reaction. The electrons liberated at anode travel through electrical load in external circuit. A large number of such cells are stacked and connected in series to get required output. On the basis of electrolyte used in the fuel cell, the fuel cells are classified as, 1. Alkaline fuel cells. 2. Phosphoric acid fuel cell. 3. Molten carbonate fuel cell. 4. Polymer electrolyte membrane fuel cell. 5. Solid oxide fuel cell. 1) H2 | O2 Fuel Cell (Alkaline) Construction 30 to 85% aq. KOH is the electrolyte. Porous carbon or plastic electrodes with catalyst like Pt, Au, Ag and metal oxides are used in these cells. Anode is the porous graphite electrode but impregnated with catalyst Ag, The anode and cathode are in the hollow cylindrical from having inlet for supply of H2 gas O2 gas respectively. The two tubular electrodes are kept in an insulator pot filled with 30 to 85% KOH solution. There is water vapor venting small holes at the top of the pot. The H2 and O2 gases are at 4 to 5 atmospheric pressure during operation. Efforts are on to make more efficient and cheaper electrodes for fuel cells. Fig. 5 Fuel Cell Reaction The H2 - O2 fuel cells is represented as, Pt, H2, KOH, O2, Ag When the fuel cell is operated using H2 gas supply to anode and O2 gas supply for cathode, the reactions take place as below: At Anode : H2 + 2OH- → 2H2O + 2e- At Cathode: 1/2O2 + H2O + 2e-→ 2OH- __________________________________ _ Net reaction: H2 + 1/2O2 → H2O The product of the reaction is water in the beginning but when the cell warms up , it is in the form of water vapors which vent through holes on top of the container. Applications 1. Fuel cells are important for use in manned space shuttles. 2. Fuel cells are used for search light for undersea use and in remote areas for lighting. 3. If enough no. of cells is connected in parallel, they can provide sufficient voltage for transportation of vehicles, industrial operations, emergency power supply to houses and societies. One fuel cell produces 0.01 watt power. Higher power can be obtained by connection of many of them. Advantages of fuel cells 1. Fuel cells have high efficiency of energy conversion. 2. No pollutants are released. 3. Fuel cells are usable for manned space shuttles, as search light, for lighting in remote areas for hospitals, houses etc. 4. Although one unit fuel cell produces 0.01 watt of power (nearly 1 volt), if many of the units connected in series in a module, enough power output can be derived. 5. Fuel cells are the guaranteed source of electrical power. 6. Low maintenance cost. 7. A saves fossil fuels (coal, petroleum fractions, NG) and are good alternative to chemical batteries. Limitations of fuel cells 1. High initial cost. 2. Large volume of fuel cell due to storage of fuel and oxygen gas. 3. Life time of fuel cell is not predictable. 4. The electrodes (along with catalyst) are costly and better working electrodes need to be discovered. 5. Fuel cells get heated up during working. 1.6: Types of Reference electrode Reference electrode is an electrode which has stable and reproducible potential. The choice of reference electrode in a particular situation depends on the substance in the solution. It is often desirable to choose an electrode which will not require a salt bridge. In general, there are two types of reference electrodes. a) Primary reference electrode - e.g. Standard Hydrogen electrode b) Secondary reference electrode - e.g. Calomel electrode, silver-silver chloride electrode, mercury-mercury sulphate electrode, mercuric oxide electrode, Quinhydrone electrode. 1.6.1: Secondary reference electrode Calomel electrode: Calomel electrode consists of an outer glass tube fitted with a frit at the bottom to permit electrical contact with solution. Inside this glass tube there is another tube having mercury at the bottom over which a paste of mercury-mercurous chloride (Hg – Hg2Cl2) is placed. A solution of potassium chloride (KCI) is then placed over the paste (fig.1). A platinum wire sealed in glass tube helps in making the electrical contact. The electrode is connected with the help of the side tube on the left through a salt bridge with the other electrode to make a complete cell. Fig.6: Calomel Electrode Representation of calomel electrode: Hg | Hg2Cl2. KCl (x M) The potential of the calomel electrode depends upon the concentration of potassium chloride solution. If KCl solution is saturated, the electrode is known as saturated calomel electrode (SCE). If KCl solution is 1N, the electrode is known as normal calomel electrode (NCE) while for 0.1N KCl solution the electrode is known as decinormal calomel electrode (DNCE). Conc. of KCl Potential of electrode Saturated KCl 0.2415 V 1.0 N KCl 0.2800 V 0.1 N KCl 0.3338 V Calomel electrode is reversible electrode means it can act as cathode or anode. When Calomel electrode acts as anode (oxidation) following reaction takes place 2Hg 2Hg+ + 2e- 2Hg+ + 2Cl- Hg2Cl2 When Calomel electrode acts as cathode (Reduction)following reaction takes place 2Hg+ + 2e- 2Hg Hg2Cl2 2Hg++ 2Cl- This indicates that when it acts as anode thickness of Hg decreases and thickness of paste Hg & Hg2Cl2 increases. If the electrode acts as cathode, the reaction is reverse. The electrode potential of any other electrode on hydrogen scale can be measured when it is combined with calomel electrode. The emf of such a cell is measured and from the value of electrode potential of calomel electrode, the electrode potential of other electrode can be calculated. Limitations Calomel electrode: Calomel electrode cannot be used above 80oC as mercurous chloride at high temperature disproportionate into mercury and mercurous chloride. 1.6.2 Ion Selective Electrode (ISE) Ion selective is an indicator electrode which produces a potential when it is placed in a solution containing a certain ion. In this electrode a suitable non-porous membrane separates the two solutions containing same ions of different concentrations potential is developed on either side of the membranes (E1 and E2). Ion selective electrodes respond to a particular ion in solution and are almost insensitive to the other ions in the solution. Hence, they are also referred to as chemical sensors. Example- Glass electrode. Glass electrode The glass electrode consists of a very thin walled glass bulb. Glass bulb is made from a low melting point glass having high electrical conductivity, blown at the end of a glass tube. The bulb contains 0.1 M HCl solution, silver wire coated with silver chloride is sealed into the glass-tube at its lower end. The lower end of silver wire dips into the hydrochloric acid thus forms silver-silver chloride electrode. Fig.7: Glass Electrode Representation of glass electrode: Ag, AgCl/ 0.1 M HCl/ H+ (Test solution) The potential of glass electrode is determined using standard calomel electrode. When glass electrode is placed in a solution the potential develops across the glass membrane as a result of a concentration difference of H + ions on the two sides of the membrane. Advantages of glass electrode: 1. It is portable and compact 2. Equilibrium is easily attained 3. It is stable electrode and can be used in presence of strong oxidizing and reducing agents. 4. It can be used in the presence of biological fluids. 5. It can detect and estimate H+ ion in presence of other type of ions. Electro Analytical Techniques 1.7: Conductometry Introduction Conductometry deals with the measurement of electric conductance of solutions containing electrolytes such as acids, bases and aqueous salt solutions. These solutions conduct electric current due to the movement of ions towards oppositely charged electrodes. When a potential difference is applied between the two electrodes. The ability of ion to transport charge depends on the mobility of ion. Mobility of ion is affected by factors like the charge on ion, size and mass of ion and extent of salvation. The Conductometry is widely used in neutralization titrations, precipitation titrations, chemical kinetics and plant laboratories etc. 1.7.1 Important laws, Definitions used in Conductometry 1. Ohm’s law: Like metallic conductors, solutions of electrolytes also obey Ohm’s law. According to this law, the current (I) passing through a given solution is directly proportional to the potential difference between the two ends of the conductor through which the current is flowing. Thus, mathematically it is expressed as, I E E = IR …….. (1.4.1) (I=Current strength, E=Potential difference, R= Proportionality constant and is characteristic of conductor. It is called as resistance.) 𝐸 ∴R= …….. (1.4.2) 𝐼 When E is measured in volts, current ‘I’ is measured in amperes. The unit of resistance (R) is known as ohm 2. Conductance (C): the ease with which the current flows through a conductor is known as conductance. OR Reciprocal of resistance is known as conductance. 1 ∴C=𝑅 …….. (1.4.3) Unit of conductance is reciprocal of ohm or mho (ohm-1). 3. Specific Resistance (ρ): the resistance R of a conductor at a definite temperature, is directly proportional to its length (l) and inversely proportional to its area (a) of cross section. Thus, 𝑙 R𝑎 or 𝑙 ∴ R = ρ. 𝑎 …….. (1.4.4) Where ρ is constant and is known as specific resistance or resistivity of the material. Its value is characteristic of the material at a specified temperature. If l = 1 cm and a = 1 cm2 then, R = ρ. …….. (1.4.5) Thus, Specific resistance of a substance is defined as the resistance offered by a cube of unit length to the passage of electricity through it. The unit of specific resistance (ρ) is, 𝑎 ρ = R. 𝑙 𝑐𝑚2 ∴ ρ = ohm. = ohm.cm 𝑐𝑚 Unit of Specific resistance (ρ): ohm.cm 4. Specific Conductance (K): The specific conductance of any conductor is the reciprocal of specific resistance. It is denoted by the symbol K. Thus, 1 K= …….. (1.4.6) 𝜌 The specific conductance of a solution can be defined as the conductance of solution between two parallel electrodes which have cross sectional area 1 cm2 and which are kept 1 cm apart. Taking inverse of (1.4.4) we get, 1 1 1 =...….. (1.4.7) 𝑅 𝜌 𝑙/𝑎 Substituting the value of 1/ρ from (1.4.6) in (1.4.7) we get, 1 1 = 𝐾. …….. (1.4.8) 𝑅 𝑙/𝑎 𝐾 OR C= …….. (1.4.9) 𝑙/𝑎 Where, C is the conductance ∴ K = C x 𝑙⁄𝑎 …….. (1.4.10) The unit of specific conductance (K) will be as, K =C x 𝑙⁄𝑎 𝑐𝑚 ∴ K = mho. = mho.cm-1 𝑐𝑚2 Unit of Specific Conductance (K): mho.cm-1 5. Cell Constant: The ratio of (l/a) in the equation (1.4.10) is known as cell constant. It is a constant for a given cell as it depends on the geometry of the cell that is on the distance between the electrodes (l) and the cross sectional area of the electrodes (a) Cell Constant= 𝑙⁄𝑎 …….. (1.4.11) The equation (1.4.10) can be written as, Specific conductance = Measured conductance (C) x Cell constant (l/a) 𝐒𝐩𝐞𝐜𝐢𝐟𝐢𝐜 𝐂𝐨𝐧𝐝𝐮𝐜𝐭𝐚𝐧𝐜𝐞 ∴ Cell constant = 𝐌𝐞𝐚𝐬𝐮𝐫𝐞𝐝 𝐂𝐨𝐧𝐝𝐮𝐜𝐭𝐚𝐧𝐜𝐞 Unit of Cell Constant: cm-1 6. Equivalent conductance (λv): Equivalent conductance is defined as the conductance of a solution containing one gram equivalent of an electrolyte at any particular concentration, when placed between two electrodes 1cm apart. Equivalent conductance of a solution is obtained by multiplying the specific conductance of the solution and the volume in milliliters which contain 1 gm equivalent of the solute. It is denoted by λv, where V is the volume of the solution in milliliters. ∴Equivalent conductance (λv ) = specific conductance (K) x Volume in ml per gram …….. (1.4.12) The volume per gram of electrolyte is called as dilution factor of the solution. If the concentration of solution is ‘C’ gm equivalent per litre, then the volume containing 1 equivalent of the electrolyte will be, 1000 V= ml per gram equivalent. 𝐶 1000 Λv = K x V = K x …….. (1.4.13) 𝐶 Unit of λv = (ohm-1 cm-1) x cm3.gm. equiv. -1 ∴Unit of λv: ohm-1.cm2. gm. equiv. -1 7. Molar conductance (µ): Molar conductance is defined as the conductance of a solution containing 1 mole of the electrolyte, when placed between two electrodes 1cm apart. It is represented as µ. Thus, 1000 µ=K x V =K x …….. (1.4.14) 𝑀𝑜𝑙𝑎𝑟𝑖𝑡𝑦 (Where V is the volume of solution in ml containing 1 mole of the electrolyte) Unit of µ = (ohm-1 cm-1) x cm3. gm.mol-1 ∴Unit of µ: ohm-1.cm2. gm.mol-1 1.7.2 Conductometric Titration Titrations in which conductance measurements are made use of in determining the end point are called as conductometric titrations. These titrations are based on the fact that the conductance of a solution at constant temperature depends upon the number of ions present in it and their mobility. In this type of titrations, the titrant is added from a burette into a measured volume of the solution to be titrated which is taken in a conductivity cell and the conductance readings corresponding to the various additions are plotted against the volume of the titrant. In this way two linear curves are obtained. The point of intersection of two linear curves is the end point. Several phenomena like hydrolysis of reactants or products, or partial solubility of a precipitated product may cause curvature in the curves. The shapes of curves obtained in certain types of titrations are discussed below. I) Acid –Base titrations: These titrations are well adapted to the conductometric end point because of the very high conductance of the hydroxide and hydronium ions compared with the conductance of reaction products. Titration of strong acid against strong base: Consider the titration of HCl with NaOH, known volume of HCl is taken in the conductivity cell and the burette is filled with NaOH. H+ + Cl- + Na+ + OH- Na+ + Cl- + H2O HCl being a strong acid dissociates completely to produce the highly mobile H + and Cl-. Hence, initially the conductivity is high. During the titration, when NaOH is added from the burette to HCl, the highly mobile H+ are replaced by the less mobile Na+. Hence, the conductivity goes on decreasing till the end-point. At the end-point that is on complete neutralization the conductivity is due to Na + and Cl-. If further NaOH is added after the end-point, the conductivity will increase due to the presence of Na+ and OH- in the solution. If the conductance is plotted against the volume of NaOH added, we get the graph as shown in fig.5. Fig.8: conductometric titration curve for strong acid against strong base 1.8 pH – Metry Introduction The concept of pH was introduced by Danish chemist Sorensen in 1909. A pH meter is an instrument that measures the hydrogen-ion activity in solutions, indicating its acidity or basicity (alkalinity) expressed as pH.pH is defined as the negative logarithm of hydrogen ion concentration. pH= -log [ H+ ] [H+ ] = Hydrogen ion concentration pH measurements are important in chemistry,biology,agriculturre ,water treatment ,food industry etc. It is often necessary to maintain a certain pH of a solution in laboratory or in the inductrial process.This is achieved by using a buffer solution. 1.8.1 A buffer solution is an aqueous solution consisting of a mixture of a weak acid and its conjugate base, or vice versa which is resistant to changes in pH when a small amount of strong acid or strong base is added to it. There are two types of buffer solutions: 1) Acidic buffers: These are prepared using a weak acid along with a salt of weak acid and a strong base. Ex: CH3COOH + CH3COONa 2) Basic buffers: These are prepared using a weak base along with a salt of weak base and a strong acid. Ex.: NH4OH + NH4Cl 1.8.2 pH Metric Measurement : An important application of potentiometry is the determination of pH of a solution. The techniques is known as pH metry. In this technique by using pH meter equivalence or end point of acid base titration can be determined. Measurement of pH The pH of a given solution can be measured by using a pH meter. Measurement of pH of a test solution is done by measuring the potential developed in an eletrochemical cell.The eletrochemical cell consist of an indicator electrode and reference electrode.These eelctrodes are immered in the test solution and connected to a voltmeter. The indicator elelctrode is a glass eelctrode and calomele eelctrode is used as the reference electrode. The indicator elelctrode is responsive to H+ and the potential developed is proportional to the pH of the solution. Standardisation (Calibration) of PH Meter Buffer solutions of pH 4,7 and 9.2 are used for standardization of pH meter. A digital pH mater is standardized as follows: i) Connect the reference and indicator electrodes to the meter and switch on the pH meter. ii) Rinse the electrodes using distilled water. iii) If the electrodes are dipped in buffer of pH=7.0 then display should show the same reading otherwise adjust the pH reading on display to 7.0. iv) Similarly pH meter is calibrated for pH 4 as well as pH 9.2 using respective buffer solutions. Fig.9: pH meter pH metric titration of strong acid vs. strong base The equaivalence point in acid base titration can be accuarately determined using pH meter. In strong acid vs strong base titration both the acid and base dissociate completetly. H+ + Cl- + Na+ + OH- Na+ + Cl- + H2O At equaivalence point ,eqaul amounts of H+ and OH- combine to form water ,resulting in a pH of 7.0. Example : Titration of HCl with 0.1 N NaOH Procedure : A) Standardisation of pH meter : pH meter is standardised using buffer solutions with pH 4,7, and 9.2.Then it can be used for the titration. B) Acid-Base Titration : Procedure: 1. Pipette out known quantity of HCl in a beaker. 2. Immerse the glass electrode and calomel electrode in it and note down the pH. 3. Fill the burette with 0.1 N NaOH. 4. Add 0.5 ml of NaOH to the acid in the beaker. Stir well and note down the pH. 5. Continue addition of 0.5 ml of NaOH and noting the pH till a sudden increase in pH is observed. Reaction: H+ + Cl- + Na+ + OH- Na+ + Cl- + H2O Fig.10: Titration curve for pH metric titration of strong acid vs strong base. Observation: From the Graph, equivalence point for the titration is noted down as X ml X ml = 0.1 N NaOH required for neutralization of HCl taken Calculations: Using N1V1 (HCl) = N2V2 (NaOH) Concentration (normality) of HCl is calculated. 1.9 UV-Visible Spectroscopy 1.9.1 Introduction Spectroscopy is the branch of science that deals with the study of interaction of electromagnetic radiations with matter. Spectroscopy is one of the powerful tools available for the study of atom and molecular structure. The spectroscopic techniques make use of either absorbed or emitted or scattered electromagnetic radiations by atoms or molecules present in the substances, which help in qualitative and quantitative analysis of substances. Electromagnetic Radiations: Electromagnetic radiation is a form of energy that is transmitted through space with high velocity. Electromagnetic radiation consists of discrete packet of energy which is called photon. Photon consists of an oscillating electric field and an oscillating magnetic field. The electric and magnetic fields are perpendicular to each other and perpendicular to the direction of propagation of photon as shown in fig.8. Fig.11. Electromagnetic wave Electromagnetic radiation propagates in space without any material medium in the form of transverse wave. An electromagnetic radiation, being a wave it is characterized by wavelength , frequency and wave number. Wavelength: Wavelength of radiation is the distance between two crests or peaks of either electric or magnetic component. It is expressed in meters. Frequency: It is the number of waves that pass through a fixed point in unit time. It is expressed in reciprocal seconds (s-1) or hertz (Hz). Wave number : It is reciprocal of wavelength expressed as reciprocal centimeters (cm-1 ) Electromagnetic spectrum: The electromagnetic spectrum consists of the arrangement of all types of electromagnetic radiations in the order of their increasing wavelengths and decreasing frequencies. The entire range of electromagnetic radiations starting from gamma rays to radio waves are covered in electromagnetic spectra Electromagnetic radiations of different wavelengths are associated with different energies. As wavelengths goes on increasing, energy associated goes on decreasing. Fig.12: Electromagentic spectrum. Fundamental of spectroscopy: Spectroscopy deals with interaction of electromagnetic radiation with matter. Due to these interactions energy absorbed or emitted by the matter. Measurement of radiation frequency indicates the change in energy involved. Spectroscopy involves measurement of spectrum of a sample contain atom or molecules. Spectrum is graph of intensity of absorbed or emitted radiations by sample versus frequency or wavelength. The study of spectroscopy can be carried out under following heads : 1) Atomic spectroscopy: It deals with interaction electromagnetic radiation with atoms. 2) Molecular Spectroscopy: It deals with interaction electromagnetic radiation with molecule. This results in transition between rotational, vibrational and electronic energy level. Spectroscopy is of two types: absorption and emission spectroscopy 1) Absorption Spectroscopy: In absorption spectroscopy, a beam of polychromatic light falls on a sample. Part of light is absorbed by the sample and remaining part is transmitted. The intensity of transmitted light coming from sample is measured at different wavelengths by suitable photo detector. Then this information is presented as graph of intensity of absorbed radiations. 2) Emission spectroscopy: In this techniques sample is subjected to intense source of energy like electric arc etc. as a result sample is vaporized and electrons in the sample are excited to higher energy state. When these electrons come back to ground state energy level they emit radiations. These radiations are analyzed by spectrophotometer to generate spectrum that gives information about atoms present in the sample 1.9.2 Interaction with Electromagnetic Radiation with Matter When an electromagnetic radiation falls on matter, it interacts with atoms / molecules Present in the matter, which exists in discrete energy states. 1. Types of energies: Molecules possess a set of discrete energy levels like atoms. i. Rotational energy (Erot): This type of energy is associated with rotation of molecule about the axis passing through centre of gravity. ii. Vibrational energy (Evib): This type of energy is associated with vibrations of molecule such as stretching or bending of bonds. iii. Electronic energy (Eelec): This type of energy is associated with motion of electrons from ground state to excited state. Fig.13: Schematic energy level diagram a) Molecular energy levels: Total energy states of molecule include electronic, vibrational and rotational levels. Rotational energy levels are very closely spaced. Little energy is required for rotational energy transitions which occur in microwave region. Vibrational energy levels are spaced further apart. Vibrational energy transitions require more energy. These transitions occur in infrared region of spectrum. Vibrational transitions are accompanied by rotational transitions. Electronic transitions: If further higher energies are applied, Electronic transitions may take place from ground state to excited state. These transitions occur in UV-visible region of spectrum. Electronic transitions are accompanied by vibrational energy changes and rotational energy changes. 3) Absorption Spectrum The molecule accepts energy only in quanta of appropriate value to cause an excitation from one energy level to another. In absorption spectroscopy we can observe the energies absorbed by sample at varying wavelength of incident radiation and measuring decreases in intensity of transmitted radiation A plot of the energy absorbed as a function of wavelength or frequency is called absorption spectrum. Fig.11 shows an absorption spectrum in which many closely spaced transitions overlap giving a broad absorption band. At a particular wavelength the absorption is maximum. The wavelength at which there is maximum absorption is called as wavelength of maximum absorption (λmax), is characteristic of molecule that helps in structure determination. Fig.14: Typical absorption spectrum 1.9.3 UV –Visible spectroscopy UV-Visible radiations are more energetic, absorption of these radiations by sample brings about electronic excitations. The electromagnetic radiations with wavelength range 10-400 nm are called ultraviolet radiation and 400-800 nm are called visible light radiations. The ultraviolet region is subdivided into two spectral regions namely , Near UV region: from 200-400 nm Far or Vaccum UV region: below 200 nm To study transition, below 200 nm, evacuated spectrometer is required. Principle of UV-Visible spectroscopy: UV and visible electromagnetic radiations arte more energetic. Absorption of uv- visible radiations by sample (substance to be analyzed) brings about electronic excitations. Electronic excitations are accompanied by vibrational and rotational changes that results in relatively broad spectra. Absorption spectrum is a graph of absorption i.e. amount of light absorbed versus wavelength of light. Information derived from absorption spectra is useful in determination of structure of molecule. 1.9.4 Beers Law and Lamberts Law The two laws which governs the absorption of light by the molecules are 1) Lamberts law 2) Beers law Fig.15 Lamberts law Consider a beam of monochromatic light passing through a transparent solution.Let I 0 be the intensity of incident radiation.when light is passed through as solution, part of light is absorbed and remaining is transmitted. Let I is intensity of transmitted light. Transmitted light has less intensity than incident light.let x be the diameter of same holder (cuvette) which is thickness (path length) of transparent solution. Lamberts law: When a beam of monochromatic light is passed through a transparent solution, decrease in intensity of incident radiation is directly proportional to path length at constant concentration. Mathematically, 𝑑𝐼 − α 𝑑𝑥 𝐼0 OR in other words Absorbance (A) is directly proportional to path length of solution at constant concentration Aαx Beers law: When a beam of monochromatic light is passed through a transparent solution, decrease in intensity of incident radiation is directly proportional to concentration at constant path length. Mathematically, 𝑑𝐼 − α 𝑑𝐶 𝐼0 OR in other words, Absorbance (A) is directly proportional to path length of solution at constant concentration AαC Lamberts- Beers law: It states that absorbance (A) is directly proportional to the path length (x) and concentration (C) A α x. c. A= a.x.c. a= Absorptivity constant If path length x is given in cm and conc. (c) is given in mol/ litre, then proportionality constant is called as molar absorptivity coefficient or molar extinction coefficient indicated by ε. Thus Beer lamberts law can be expressed as Amount of light transmitted is given by transmittance (T) 𝐼 𝑇= 𝐼0 The relation between Absorbance (A) and Transmittance (T) is given by A = - log T 1.9.5 Absorption of UV Radiation by organic molecule leading to different electronic transition When energy is absorbed by a molecule in uv n-visible region brings changes in electronic energy of molecule because of transition if valence electrons form lower energy to higher energy. 3 types of electrons are involved in organic molecule. I) sigma (σ) electron : Electrons forming single bonds are called sigma electrons.these are involved in forming bonds in saturated hydrocarbons such as involving c-h bonds II) Pi (π) electrons: Electrons involved in forming double bonds are called pi electrons. These are involved in forming bonds in unsaturated hydrocarbons such as involving c=-c or c≡ 𝑐. III) n electrons: These are non bonded or lone pair of electrons and not involved in bonding between atoms in molecules. For eg.lone pair of electrons present on oxygen, nitrogen etc. When energy is absorbed in uv-visible region, possible electronic transitions are: σ σ*, σ π*, π σ*, π π*, n σ*, n π* out of these, σ π*, π σ*, are considered as forbidden transitions. The relative energies are in the following order: 1) σ σ* Transition: σ-electron from bonding orbital is excited to the corresponding antibonding orbital. The energy required is large for this transition. Saturated compound without lone pair of electrons show this type of transition. Ex.: Methane (CH4) Absorption maxima due to σ σ* transitions are not seen in typical UV-visible spectra. Thus saturated hydrocarbons absorb very short wavelength below 185 nm and therefore this absorption is beyond the range of ordinary UV-visible spectrophotometer. 2) π π* Transition: π-electron in a bonding orbital is excited to corresponding antibonding orbital π*. Unsaturated compound without lone pair of electrons show this type of transition. Alkenes generally absorb in the region 170-250 nm and are difficult to record by spectrophotometer. However, molecules containing two or more conjugated double bonds absorb above 200 nm and can be easily recorded. Ex.: Alkenes, Alkynes, Nitriles, aromatic compounds. Fig.16: Electronic Transitions 3) n σ* Transition: Saturated compounds containing atoms with lone pairs like O,N, S and halogens are capable of this type of transition. These transitions usually require less energy than σ σ* transitions. Ex.: Ethanol, Amines, Thiols. 4) n π* Transition: an electron from nonbonding orbital is promoted to antibonding π* orbital. Unsaturated compounds with lone pair of electrons undergo such a transition. n π* transitions require lowest energy for absorption. It shows absorption at longer wavelength around 300 nm. Ex.: Aldehydes, Ketones, Carboxylic acids, Esters, Anhydrides, Amides. 1.9.6 Terms Involved in UV-Visible spectroscopy a) Chromophore: Chromophore is a functional group containing multiple bond capable of absorbing radiations above 200 nm due to π π*, n π* transitions. Example: NO2, N=O, C=O, HC N, C=C, C=S. b) Auxochrome: Auxochrome is a functional group with non-bonding electrons that does not absorb radiation in near UV region but when attached to a chromophore alters the wavelength and intensity of absorption. It causes shift of absorption to longer to wavelength since introduction of this groups extends the conjugation of a chromophore by sharing non-bonding electrons. Example: OH NH2 Benzene Phenol Aniline λmax = 255 nm λmax= 270 nm λmax = 280 nm. a) Bathochromic shift (Red Shift): when an absorption maximum (λmax) of a compound shifts to longer wavelength it is known as bathochromic shift or red shift. This effect is due to the presence of an auxochrome or by change of solvent. Example: auxochrome group like –OH, -OCH3, -NH2 causes absorption of a compound at longer wavelength. p-nitro phenol shows red shift in alkaline medium since negatively charged oxygen delocalizes electron more effectively than the non bonding electrons present on –OH group. O O O O N N OH- Alkaline Medium OH O λmax = 255 nm λmax = 265 nm b) Hypsochromic shift (Blue Shift): when an absorption maximum (λmax) of a compound shifts to shorter wavelength it is known as hypsochromic shift or blue shift. This shift is caused due to removal of conjugation or due to change in polarity of solvent. Example: Aniline shows blue shift in acidic medium due to loss of conjugation. NH2 NH3Cl Acidic Medium λmax = 280 nm λmax = 265 nm c) Hyperchromic shift: An increase in intensity of absorption maxima is called hyperchromic shift. Introduction of auxochrome usually increases the intensity of absorption. Example: N N CH3 Pyridine 2-Methyl pyridine λmax = 257 nm λmax = 260 nm ε= 2750 ε = 3560 d) Hypochromic shift: Decrease in intensity of absorption maxima is called hypochromic shift. It occurs due to introduction of groups that distorts the original geometry of the molecule. Example: CH3 Naphthalene 2-Methyl naphthalene ε= 1900 ε = 10250