CHEM3012 Enzymology Lecture 1 PDF
Document Details
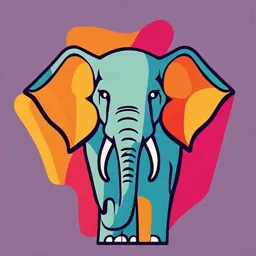
Uploaded by GloriousVerse5291
Ellis O'Neill
Tags
Related
- Areas Related to Biochemistry PDF
- TD Biologie - Examen - QCM PASS 2023-2024
- Enzymology In Medical Biotechnology PDF Lecture Notes 2022/2023
- Enzymology BCH3108 Amm Sulphate PDF
- CHEM271 Introductory Biochemistry I Fall 2024 Lecture Notes PDF
- CHEM 3310H Lecture 18 - Protein Chemistry and Enzymology PDF
Summary
This document provides lecture notes on enzymology, focusing on the fundamental concepts like evolution and protein structure. It details amino acids, their properties, and different types of protein structures. The lecture notes include a table of amino acids, their features, and abbreviations.
Full Transcript
[CHEM3012] [Enzymology] **Ellis O'Neill** Lecture 1 - Evolution is not "survival of the fittest" but death of the not fit enough - Evolution operates at a population level - Evolution changes at DNA level changing phenotype of organism (DNA-\>RNA-\>Protein) so evolution is change...
[CHEM3012] [Enzymology] **Ellis O'Neill** Lecture 1 - Evolution is not "survival of the fittest" but death of the not fit enough - Evolution operates at a population level - Evolution changes at DNA level changing phenotype of organism (DNA-\>RNA-\>Protein) so evolution is change of protein - Random mutation is single base change - Genetic drift is accumulation of these changes in a population - Selection is removal of unfavourable changes - With no selection there is just random genetic drift - Divergent evolution is 1 species diverging with no cross breeding - Convergent evolution is enzymes sharing result but not shape or mechanism - EC number of enzyme describes their function - Primary structure is assembly - Secondary structure is folding - Tertiary structure is packing - Quaternary structure is interaction - - All amino acids with R ≠proton are chiral - The α-carbon is sp^3^ hybridised and bonded to 4 different groups - 'CORN' rule: - H is lowest priority and goes to the back - Follow round from CO (carboxylate) -\> R group -\> N - If anticlockwise (left) it is L - If clockwise (right) it is D - All proteinogenic amino acids are L **Name** **Three letter code** **One letter code** **Features** --------------- ----------------------- --------------------- --------------------------------------------------------------------------------- Alanine Ala A Small (alkyl side chain) Arginine Arg R Basic (positively charged at physiological pH) (N on side chain) Asparagine Asn N Amide (carboxamide on side chain) (polar but non charged) Aspartic acid Asp D Acidic (negatively charged at physiological pH) (carboxylic acid on side chain) Cysteine Cys C Nucleophilic and contains sulphur (polar but non charged) Glutamic acid Glu E Acidic (negatively charged at physiological pH) (carboxylic acid on side chain) Glutamine Gln Q Amide (carboxamide on side chain) (polar but non charged) Glycine Gly G Small Histidine His H Basic (positively charged at physiological pH) (N on side chain) Isoleucine Ile I Hydrophobic (alkyl side chain) Leucine Leu L Hydrophobic (alkyl side chain) Lysine Lys K Basic (positively charged at physiological pH) (N on side chain) Methionine Met M Hydrophobic and contains sulphur (non-polar) (all proteins start with a Met) Phenylalanine Phe F Aromatic (non-polar and bulky) Proline Pro P Hydrophobic Serine Ser S Nucleophilic (hydroxyl on side chain) (polar but non charged) Threonine Thr T Nucleophilic (hydroxyl on side chain) (polar but non charged) Tryptophan Trp W Aromatic (non-polar and bulky) Tyrosine Tyr Y Aromatic (non-polar and bulky) Valine Val V Hydrophobic (alkyl side chain) -  - Bond rotations along main chain of polypeptide are restricted to C~α~-N and C~α~-C bonds - Amide/peptide bonds have partial double bond character, cannot rotate freely and trans more stable - Ramachandran plot describes rotation of these bonds - Range of phi and psi angles is wider for glycine and less wide for proline than that for most amino acids Lecture 2 - α-helix: - H bonds between main chain N and O atoms are important for helix stabilisation - 3.6 amino acids per complete turn of the helix - Every 7^th^ amino acid is next to each other giving 2 turns so the side chains are next to each other - Side chains stick out of the helix so can give different chemistry to each side - β-sheet: - H bonds between main chain N and O atoms determine the sheet stabilisation - Can be either parallel or anti-parallel ([⇉]{.math.inline} or [⇆]{.math.inline}) - Side chains stick out of the sheet so can give different chemistry to each side - α-helices and β-sheets are linked by loops and turns (random coils) - Hydrophobic side chains form hydrophobic cores to minimise interactions with water - Further side chain interactions with each other and the solvent stabilise the structure - Ionic interactions (salt bridges) between charged side chains will strongly stabilise different regions of the protein - Cys residues form disulphide bridges - A motif is a small, specific combination of secondary structure elements (e.g. β-α-β loop) - Motifs then combine to form domains (e.g. α/β barrel) - Proteins can have multiple domains and a domain can have a specific function - - Protein structure can involve clustering of several individual polypeptide chains known as quaternary structure - Variety of bonding interactions (H-bonding, salt bridges and disulphide bonds) hold various chains in place - If final protein structure is made of single peptide chain it is said to be monomeric - Proteins can be formed of multiple polypeptides: - Can be identical (they come from the same gene, homo- prefix) - Can be different (from different genes, hetero- prefix) - Dimers, tetramers, octamers etc. are very common protein structures - Each polypeptide unit is called a subunit - Post-translational modification is chemical change protein undergoes after translation - Such modifications are mostly catalysed by enzymes that recognise specific target sequences in specific proteins - Phosphorylation: - Amino acids containing OH group are often phosphorylated by kinase by addition of covalently bound phosphate group - Change chemistry of protein, its interactions or control its transfer across membranes - Reversible by phosphatases - Abnormal protein phosphorylation implicated in cancer and degenerative disorders - Glycosylation: - Carbohydrates attached to protein residue to form glycoprotein - N-Glycans linked through Asn - O-Glycans linked through Ser and Thr - Essential in protein folding - Major role in cell-cell recognition and signalling - Actin is the most abundant protein in most cells: - Main component of cytoskeleton - Forms fibrils throughout cell - Ferritin for mineral storage: - Oxidises and incorporates iron and keeps it in a non-toxic form - Large cavity for iron storage separated from solution by a protein shell - Ion channels: - Integral membrane protein (more typically assembly of several proteins) - Circular arrangement of proteins closely packed around water-filled pore through membrane or lipid bilayer - Enzymes are proteins that catalyse reactions - Upon binding of substrate to active site, the enzyme changes shape, generally closing around the substrate (induced fit) - Catalytic residues are often acids or bases: - - All enzymes within same family will have same catalytic residues. These residues are conserved and often a fingerprint for enzyme classification - Very specific control of stereochemistry by binding substrate in correct conformation - Proteins can change shape e.g. enzyme binding substrate - This can be more distant e.g. inhibitor binding - Allosterics is assemblies of functional units which undergo quaternary structural transitions in response to concentration changes of a specific ligand - Haemoglobin (Hb) is an allosteric protein: - The binding of oxygen to one subunit affects the binding of oxygen to other subunits - This is called cooperative binding and is reflected in haemoglobin's sigmoidal curve -  - Trivial enzyme name is adding -ase at the end of the reaction they catalyse - Enzyme commission (EC) numbers: - 4 digit number describing - 1\. Enzyme class - 2\. Bond acted on - 3\. Donor or acceptor - 4\. Specific reaction - E.g. 1.2.3.4. is oxalate oxidase Lecture 3 - Rate of reaction, [\$V\_{t} = - \\left( \\frac{d\\left\\lbrack S \\right\\rbrack}{\\text{dt}} \\right)\_{t} = \\left( \\frac{d\\left\\lbrack P \\right\\rbrack}{\\text{dt}} \\right)\_{t}\$]{.math.inline} - At time 0 (t~0~) we start with a known concentration of S, \[S\]~0~, and the initial rate (V~0~) in the absence of P is proportional to \[S\]~0~ so V~0~ = k\[S\]~0~ where k is the forward reaction rate constant - For the reverse reaction V~0~ = k'\[P\]~0~ - The rate at which S converts to P is given by k\[S\] and the rate at which P converts to S is given by k'\[P\] - Net conversion, V = k\[S\] -- k'\[P\] - Enzymes speed up reactions so initial reaction rates are difficult to measure - Assumptions: - Substrate concentration in vast excess relative to enzyme concentration - Rapid equilibrium is achieved and maintained at all times, k~1~ = k~-1~ when t\>0 - Enzyme is 'not used up' and can only exist as free E or as ES - \[ES\] steady state so formation = breakdown - [\$E + S\\begin{matrix} k\_{1} \\\\ \\rightleftarrows \\\\ k\_{- 1} \\\\ \\end{matrix}\\text{ES}\\begin{matrix} k\_{2} \\\\ \\rightarrow \\\\ \\ \\\\ \\end{matrix}E + P\$]{.math.inline} - Formation of ES = k~1~\[E\]\[S\] - Breakdown of ES = k~-1~\[ES\] + k~2~\[ES\] - [\$\\left\\lbrack \\text{ES} \\right\\rbrack = \\frac{\\left\\lbrack E \\right\\rbrack\_{t}\\left\\lbrack S \\right\\rbrack}{\\left\\lbrack S \\right\\rbrack + \\frac{\\left( k\_{- 1} + k\_{2} \\right)}{k\_{1}}}\$]{.math.inline} - V~0~ = k~2~\[ES\] - v~max~ is the maximum rate where all enzyme is involved and limited by k~2~ - v~max~ = k~2~\[E\]~t~ - K~M~ is Michaelis constant - K~M~ [\$= \\frac{\\left( k\_{- 1} + k\_{2} \\right)}{k\_{1}}\$]{.math.inline} - [\$V\_{0} = \\frac{V\_{\\max}\\left\\lbrack S \\right\\rbrack}{K\_{M} + \\left\\lbrack S \\right\\rbrack}\$]{.math.inline} - For high concentrations of substrate, V~0~ approaches V~max~ - In the presence of fixed \[E\], V~0~ is not linearly related to \[S\]~0~ - If \[S\] is kept constant, V~max~ is proportional to \[E\] - V~max~ = k~cat~\[E\]~t~ - When V~0~ is half V~max~, K~M~ = \[S\] so K~M~ can be experimentally found from \[S\] that gives half maximum V - The catalytic efficiency of an enzyme is defined by [\$\\frac{k\_{\\text{cat}}}{K\_{M}}\$]{.math.inline}: - High k~cat~ = rapid reaction - High K~M~ = doesn't bind substrate tightly -  **Reversible inhibitor** **Irreversible inhibitor** ------------------------------------------------------- ----------------------------------------- Removable Non-removable Remove inhibitor and enzyme recovers Enzyme cannot recover Non-covalent interaction between enzyme and inhibitor Usually a chemical reaction is involved Usually fast Usually slow - V~max~ changes and K~M~ unchanged for competitive and non-competitive inhibitors - Cofactors: - Chemical compound or ion that is required for an enzyme's role - Have features that help bind substrates - Take part in the reaction as part of transition states - Form reaction intermediates - Carry electrons in redox reactions - Coenzymes provide chemical functionalities that are not available in amino acids e.g. nicotinamide adenine dinucleotide (NAD) - Prosthetic groups mainly contribute to enzyme structural stability - An enzyme without its cofactor is known as apoenzyme - NAD: - NAD+ accepts the hydride from the alcohol during its oxidation to form NADH - NAD binds transiently to the enzyme - NAD+ makes the enzyme function because one of the end products of this reaction (hydrogen) is removed from the reaction site - Thiamine pyrophosphate (TPP): - Derived from vitamin B~1~ - Involved in forming and breaking C-C bonds - TPP+ loses hydrogen to form TPP Ylide Lecture 4 - Cobalamin: - Vitamin B~12~ - Can shuttle between Co^I^, Co^II^ and Co^III^ - R can be a range of groups including CN, HO and Me - E.g. methionine synthase - Biotin: - Vitamin B~7~ - Transfer of carboxylate - E.g. pyruvate carboxylase  - Pyridoxal phosphate (PLP): - Vitamin B~6~ - Forms Schiff base with amines including enzyme active site - Carries out amino transferases, eliminations and isomerisations - Proteases (peptidases) cleave the peptide bond between amino acids: - Exopeptidases cleave the terminal peptide bonds releasing an amino acid and reducing the chain length by one - Endopeptidases cleave internal peptide bonds releasing two peptides - The overall reaction is hydrolytic - Serine proteases: - The active site contains a catalytic triad of aspartate to align the histidine and act as a general acid, histidine to activate the serine and act as a base and serine to act as a nucleophile - The active site Asp in serine proteases maintains the imidazole group of the active site His in the tautomeric form - The cleaving reaction proceeds in two steps of formation of an acyl-enzyme intermediate involving the reactive Ser residue in the active site and hydrolysis of the acyl-enzyme intermediate - Most serine proteases exhibit no absolute substrate specificity - Polypeptides bind to the enzyme through H bonds between substrate main chain atoms in a short antiparallel β-sheet and enzyme loop main chain atoms - Many serine proteases show a preference for a particular side chain before the cleaved peptide bond - Selectivity pockets: - Negative charge, aspartate, trypsin prefers positively charged amino acids - Small, serine, chemotrypsin prefers positive, bulky amino acids - Bulky, valine/threonine, elastase prefers small, non-polar amino acids - Cysteine proteases: - E.g. papain, ficin, bromelain - pK~a~ of the Cys side chain (2) is a much stronger acid than the Ser side chain (13) so no need for Asp acid - His, Cys catalytic dyad - Papain: - Cys^25^ (nucleophilic attack) - His^159^ (general acid/base) - Oxyanion hole - Cys^25^ and Gln^19^ stabilise the oxyanion via H-bonding interactions - Binding site accommodates 7 amino acids - Aspartyl proteases: - E.g. pepsin, chymosin, HIV-protease - Cleaving reaction proceeds through general acid-base catalysis - Thiol proteases use a C, H catalytic dyad - Biotechnology: - Cleaning agents e.g. biological detergents - Meat tenderiser e.g. pineapple steak marinade - Cheese making e.g. rennet and vegetarian rennet - Protein engineering e.g. TEV protease cleaves at a specific sequence removing tags - Protein fingerprinting (proteomics) e.g. protease cleaves at specific positions based on specificity pocket **Luisa Ciano** Lecture 1 - Na, Mg, K and Ca are abundant metal ions (0.1% of human body weight) - V, Cr, Mn, Fe, Co, Ni, Cu, Zn, Mo and W are trace metals (μM concentration in body) (narrow safe concentration window) - B, C, N, O, F, P, S, Cl, Se, Br and I are main building blocks of living matter (make proteins, DNA, sugars, lipids) (95% of human body weight) - Group 1 and 2 metals (Na, K, Mg, Ca) have structural role in both body (e.g. bones) and protein folding, or aid in maintenance of charge, osmotic balance and nerve impulses - Transition metal ions can have structural role (Zn fingers), or be electron carriers, oxygen transporters (Fe, Cu), catalysts in redox and non-redox processes - Proteins bind to metal via side chains of coordinating residues, which can act as ligands for metal ions -  - Coordination modes found in metalloenzymes: - Tyr binding as a neutral ligand via lone pair of oxygen atom - His biding as a negative ligand via deprotonated imidazole moiety - Cys binding as a negative ligand via deprotonated thiol moiety - Porphyrins are an example of a chelating ligand -\> two or more donor atoms from same ligand. Chelation increases stability of complex - - Metals bind to ligand according to Hard Soft Acids Bases (HSAB) Principle - Hard acids: metals with small ionic radius and/or high charge (Na, Ca, Mg, K, Mn(II), Fe(III), Co(III)) - Soft acids: metals with large ionic radius, more polarisable (Pt(II/IV), Hg, Cd, Cu(I)) - Cys is soft - Ser, Thr, Asp and Glu are hard - Hard bases: ligands with high electronegativity (typically O-based) (-OH, -COO^-^) - Soft bases: ligands with low electronegativity and polarisable (sulphur or phosphorus based) - Intermediate metals: Zn(II), Cu(II), Fe(II), Co(II), Ni(II) - Intermediate ligands: N-based ligands - Binding of ligands to metal splits energy of d-orbitals (Ligand Field theory) Lecture 2 - Zinc is found in variety of enzymes, such as carbonic anhydrase, carboxypeptidase, superoxide dismutase (SOD), β-lactamase, alcohol dehydrogenase etc. - In some cases it's important to maintain protein structure and stability (e.g. SOD), in other cases it participates directly in catalysis (e.g. carbonic anhydrase, carboxypeptidase) - Zn acts as Lewis acid - Zn(II) d^10^ borderline hard soft, acid base interacts with O or N - Therefore, it's found in hydrolytic enzymes, does not catalyse redox reactions - Metalloproteases (also called metalloproteinases) are metal-based enzymes that cleave the peptide bond. They require Zinc (some can use Co) - Metalloproteases lose activity if metal is removed - Exopeptidases -- process from either end and cleave terminal or penultimate peptide bond, releasing amino acids or dipeptides - Endopeptidases -- break peptide bonds of non-terminal amino acids (i.e. in middle of chain). Usually specific for certain amino acids (Trypsin cuts after Arg or Lys) -  - Carboxypeptidase A: - Exopeptidase that cleaves C-terminal (Phe, Tyr, Trp or Leu favoured) - Produced by pancreas, crucial in processes such as digestion, blood clotting and post-translational modifications - Zn^2+^ ion coordinated by 2 x His, Glu and water/hydroxide molecule in distorted tetrahedral geometry - Zn can be removed at low pH or using chelating agent -\> loss of activity, but no effect on overall structure - Activity can be re-established if Zn is added to apoenzyme 1. Carbonyl of peptide backbone substitutes bound water molecule in coordinating Zn^2+^ 2. Zn acts as Lewis acid and polarises C=O group, making it more susceptible to nucleophilic attack by water molecule activated by nearby Glu residue 3. Resulting intermediate can evolve by addition of proton to amide from nearby Tyr residue, hence giving good leaving group and breaking peptide bond 4. Cleaved amino acid residue is released and new C-terminal is produced, with water binding to Zn^2+^ and reforming resting state 5. Protonations and deprotonations between residues and water molecules, followed by coordination of carbonyl from next residue, close cycle - Carboxypeptidase A catalyses cleavage of peptide bonds (very slow reaction without catalyst) from C-terminal of protein - Reaction catalysed by carboxypeptidase A is hydrolysis catalysed by Zn ion - Zn polarises C=O bond by acting as Lewis acid, while nearby residues act as acids/bases to create concerted mechanism - Carbonic anhydrases: - Family of Zn enzymes that catalyse interconversion of CO~2~ and water into carbonic acid - CO~2~ + H~2~O [⇌]{.math.inline} HCO~3~^-^ + H^+^ - Crucial in regulating pH and haemoglobin function - One of the fastest enzymes known, typically its rate is diffusion limited - Zn^2+^ ion coordinated by 3 x His and hydroxide in distorted tetrahedral geometry. Nearby Thr (His in some families) helps with formation of hydroxide - Zn can be removed using chelating agent -\> loss of activity, but no effect on overall structure - Activity can be re-established if Zn is added to apoenzyme 1. Zn acts as Lewis acid, lowering pK~a~ of bound water and forming hydroxide 2. CO~2~ is in pocket that brings it close to HO^-^ 3. HO^-^ does nucleophilic attack on CO~2~ 4. Bicarbonate formed is displaced by water, which is then deprotonated to close cycle - Carbonic anhydrase catalyses reversible hydration of CO~2~ to carbonic acid (very slow reaction without catalyst) - Zn lowers pK~a~ of bound water molecule, with formed hydroxide anion being effective nucleophile for reaction with CO~2~ Lecture 3 - Iron is the most abundant transition metal in the human body(\~4-6g in an average person) - Changes in Fe levels are usually associated with diseases, such as anaemia, haemochromatosis or siderosis - Fe-proteins and enzymes have a variety of roles (e.g. electron transport, oxygen transport, redox reactions) and structural motifs (haem, non-haem, Fe-S clusters) - Fe(III) -\> insoluble - Fe(II) -\> soluble - Fe(III) is insoluble in physiological conditions - Fe(II) is readily oxidised to Fe(III) in physiological conditions - Transferrin is used for the transport of Fe in the body - Transferrins bind Fe(III) in a pH dependent manner, with 2 Fe and 2 Carbonate (CO~3~^2-^) ions per protein in a distorted octahedral geometry - Once Fe has been transported, it is concentrated and stored within ferritins - Ferritins have been found in every living organism and are the only known proteins that can direct a phase transition between metal ions in solution and in a solid - In anaerobic environments Fe(II) (soluble) is prevalent, but in aerobic environments Fe(III) is found - Fe(III) is stored in a hydrated ferric oxide in the centre of the protein - Haemoglobin and myoglobin contain haem centres coordinating Fe(II) (deoxy) or Fe(III) (oxy) - Cytochrome c: - Fe-based protein with a crucial role in electron transfer processes - Mitochondrial cytochrome c transfers electrons between proteins involved in the respiratory chain reactions - Haem-Fe centre, but with His/Met coordination in the axial positions - Fe-S proteins: - Involved in a variety of processes including photosynthesis and cellular respiration - Fe is typically in a tetrahedral geometry, coordinated to thiolates from Cys residues or sulphide ions - Fe-S clusters allow for fast electron transfers across the full biological range of physiological redox potentials (-600mV to +460mV), tuned by the H-bonds around the cluster - Some Fe-S clusters are reported to have hydrolytic activity - - Haem and non-haem centres are found in Fe enzymes - Vast majority of Fe enzymes are involved in redox reactions, most notably O~2~ activation -  - Oxygen is an essential component for three main roles necessary for life: - Production of energy ([\$O\_{2}\\begin{matrix} 4e\^{-} \\\\ \\rightarrow \\\\ 4H\^{+} \\\\ \\end{matrix}2H\_{2}O\$]{.math.inline} coupled with ATP production) - Oxidative metabolism of compounds (synthesis, metabolism) - Defence of the organism against infections ([\$O\_{2}\\begin{matrix} e\^{-} \\\\ \\rightarrow \\\\ \\ \\\\ \\end{matrix}O\_{2}\^{\* -}\$]{.math.inline}) - Reactions of O~2~ with organic substrates are exothermic and thermodynamically favoured, but they are slow -\> catalyst is needed to activate and control the reaction - -  - Under normal conditions, these species are formed in all tissues and are controlled by enzymes to reduce their presence in the cells (e.g. SOD, catalase) - Lower activity of these protective enzymes leads to accumulation of O~2~-derived radicals -\> oxidative stress - Depending on the reaction mechanism and the product obtained, the enzymes involved in O~2~ activation are divided in: - Monooxygenases catalyse the addition of one oxygen atom from O~2~ to the substrate - Cytochrome P450 (CYP450): - CYP450 monooxygenases are widely found in living organisms. In humans, they are mostly found in the liver - They transfer electrons from NADPH or NADH to the active site, which activate O~2~ and insert one atom of oxygen into the substrate:  - First main role is specific oxidation steps during biosynthesis or biodegradation of endogenous compounds (e.g. steroids or fatty acids) -\> generally very specific for substrate with high regio- and stereoselective reactions - Second main role is oxidative metabolism and elimination of exogenous compounds from the body (e.g. drugs) -\> generally not very selective 1. Substrate binding near the active site causes a conformational change of the active site displacing a water molecule, and changing the Fe from low spin to high spin 2. This induces electron transfer from a reductase or a reductant, reducing the Fe(III) to Fe(II) 3. O~2~ binds to the Fe(II) centre, forming an adduct similar to what is found in oxymyoglobin 4. A second electron is transferred, giving a short lived peroxo intermediate 5. Rapid addition of two protons and elimination of water forms the very reactive Compound I - P450 are haem-based monooxygenases responsible for the 2e^-^, 2H^+^ hydroxylation of a variety of substrates, often breaking strong C-H bonds - They activate molecular oxygen in a controlled mechanism triggered by binding of substrate - The Fe cycles through different oxidation states (II, III and IV) aided by a non-innocent porphyrin ring ligand Lecture 4 - Cu(I) prefers softer donors such as sulphur or phosphorus and a lower coordination number like 2, 3 or 4 - Cu(II) prefers harder donors such as oxygen or nitrogen and a higher coordination number like 4, 5 or 6 - Hemocyanin is a Cu-based oxygen transport protein found in invertebrates - Cu-proteins have been divided in three classes: - Type I: mononuclear Cu sites characterised by intense blue colour and high reduction potential. The Cu is coordinated by 2 Histidines and a Cysteine, plus a fourth ligand (e.g. Met) - Type II: mononuclear Cu sites with N or N/O coordination and square planar/JT distorted octahedral geometry. Colour is typically not intense - Type III: dinuclear copper centres coordinated by His residues. EPR silent due to antiferromagnetic coupling between the ions - LPMOs: - Are Type II copper monooxygenase enzymes involved in the degradation of polysaccharides - LPMOs are monooxygenases - LPMOs cleave polysaccharides - LPMOs have the N-terminal amine in the active site - Are included in all commercial enzymatic cocktails used for the production of bioethanol - Have a unique active site which involves coordination from the amino terminus of the protein ("His brace"). The active site is located on the surface of the enzyme - Catalyse the selective oxidation (C1 or C4) of C-H bonds in polysaccharides - Responsible for the 2e^-^, 2H^+^ hydroxylation of polysaccharides, breaking strong C-H bonds - They activate molecular oxygen in a controlled mechanism triggered by binding of substrate - The Cu cycles through different oxidation states (I, II (and III?)) producing oxygen intermediates similar to those observed in P450 enzymes Lecture 5 - Galactose oxidases: - Are Cu-dependent oxidases - Are active on a variety of substrates (not just galactose), such as aliphatic and aryl alcohols, sometimes with high substrate selectivity - The reaction catalyses the oxidation of alcohols to aldehydes with concomitant O~2~ reduction: RCOH + O~2~ -\> RCHO + H~2~O~2~ - Role is not clear. Possibly antibacterial production of H~2~O~2~ - Are a type II copper protein. The metal is in a distorted square pyramidal geometry coordinated by 2 x His, 2 x Tyr and 1 H~2~O - The active site with the thioether link represents the mature form of the protein - The modification happens spontaneously in the presence of copper in aerobic conditions - The modification is thought to lower the redox potential of the Tyr, making it easier to oxidise to the tyrosyl radical -  1. The mechanism starts with the displacement of the coordinated water molecule in the active site by the alcohol 2. This is followed by a proton transfer from the alcohol to the tyrosinate 3. A proton coupled electron transfer reduces the tyrosyl radical, while another electron reduces the copper. At the same time, the alcohol is oxidised to an aldehyde 4. The aldehyde is released while O~2~ binds to the active site 5. The tyrosine is oxidised back, providing another electron and a proton to form the hydroperoxo intermediate 6. Another proton transfer from the tyrosine releases hydrogen peroxide and restarts the cycle - Their active site is characterised by a modified Tyr-Cys residue that aids in the electron transfer process during the mechanism - The 2e^-^ and 2H^+^ removed from the alcohol during the first part of the cycle are used to reduce O~2~ to H~2~O~2~ - The Cu cycles through different oxidation states (I, II) in a mechanism aided by the residues in the active site - Reactive oxygen species (ROS) are constantly produced in living organisms as part of O~2~ activation during metabolic processes - There are three main classes of antioxidant (ROS-protecting) enzymes: - Superoxide dismutase: catalyses the dismutation of superoxide into hydrogen peroxide and O~2~ (2O~2~^\*-^ + 2H^+^ -\> O~2~ + H~2~O~2~) - Catalase: Fe-haem enzyme, uses hydrogen peroxide as a substrate to produce O~2~ and water (2H~2~O~2~ -\> 2H~2~O + O~2~) - Glutathione Peroxidase: a selenoprotein, reduces hydrogen peroxide to water while oxidising glutathione (2GSH + H~2~O~2~ -\> GS--SG + 2H~2~O) - Superoxide dismutase (SOD): - - M can be Cu(I), Mn(II), Fe(II) or Ni(II) - Mn, Fe and Ni cycle between +2 and +3 - Cu(I) = M^n+^, Cu(II) = M^(n+1)+^ - FeSOD is found in both eukaryotes and prokaryotes, either as a homodimer or a homotetramer. The active site comprises three His and an Asp residue - MnSOD are found in all mitochondria and some bacteria - NiSOD are mostly found in prokaryotes as a homohexamer, with one Ni active site per subunit. The hydrophobic core drives the assembly of the quaternary structure - The Ni binding site is disordered in the absence of the metal and has a Pro that causes a turn in the structure, and hence is called the "Ni binding hook" - Cu/ZnSOD is found in all eukaryotes - It contains both Cu and Zn in the active site: Zn has mostly a structural role, while Cu is responsible for the catalysis. The metals are bridged by an imidazolate moiety [Chemical Biology] **Neil Thomas** Lecture 1 - Measuring protein concentrations using UV absorption: - Absorption near UV depends on W and Y content and to a small extent -S-S- bonds (the presence of C) - W = Trp, Y = Tyr, C = Cys - Simple and sample is recoverable - Interference from chromophores, nucleic acids - Far UV absorbance: - Peptide bonds absorb at 190nm - Difficulties with oxygen absorption, low output, buffer absorption - Better to use 205nm but absorbance is half that at 190nm - Must calibrate the spectrophotometer accurately as many buffers and their components, haem, pyridoxal groups etc also absorb strongly at 205nm - Near UV absorbance: - More reliable and easier to use 280nm - No problems with buffer absorption - A = ε c l - 5690 extinction coefficient for W - 1280 extinction coefficient for Y - 120 extinction coefficient for C - A~280~ (1 mg/ml) = (5690 W + 1280 Y + 120 C) / M where M is the molecular weight of the protein in Daltons - Correction for presence of nucleic acids: Protein (mg/ml) = 1.55 A~280~ -- 0.76 A~260~ - Absorbance at 260nm is dominated by nucleic acids - Lowry Method: - The first reaction is the formation of a copper ion complex with amide bonds, forming reduced copper in alkaline solutions. This is called a "Biuret" chromophore - The second reaction is the reduction of Folin-Ciocalteu reagent (phosphomolybdate and phosphotungstate reduced to heteropolymolybdenum blue) by tyrosine and tryptophan residues - The reduced Folin-Ciocalteu reagent is blue and thus detectable with a spectrophotometer in the range 500 -- 750nm - The Biuret reaction itself is not all that sensitive - Using the Folin-Ciocalteu reagent to detect reduced copper makes the assay nearly 100x more sensitive than the Biuret reaction alone - Results in a strong blue colour at 750nm (depends on W, Y content) - The absorbance can be read in the region of 500 -- 750nm - Most researchers use 660nm - Sensitive down to 0.01 mg/ml - Best used between 0.01 -- 1.0 mg/ml - Bicinchoninic Acid (BCA) Assay: - Cu(II) to Cu(I) conversion under alkaline conditions - Cu(I) detected by reaction with BCA - Strong purple colour - Absorbance at 562nm - Similar sensitivity to Lowry Method - Since BCA is stable under alkali conditions, this assay has the advantage that it can be carried out as a one-step process - The production of Cu+ in this assay is a function of protein concentration and incubation time - The protein content of unknown samples may be determined spectrophotometrically by comparison with known protein standards - Bradford Method: - Binding of Coomassie Blue G250 to protein - Free dye in 3 ionic forms - The more cationic orange-red form has absorbance maximum at 470nm - The more anionic blue form that binds to protein absorbs at 590nm - The absorption spectra of the two forms of the dye overlap causing the assay to respond non-linearly in the standard curve - Dye binds most readily to arginine and lysine residues which can lead to variation in the response for different proteins - Protein Electrophoresis: - Non-denaturing: Native protein (preserves activity) - Denaturing: Denatured protein (loss of activity) - Polyacrylamide Gels: Polymerisation of acrylamide in the presence of N,N'-methylene-*bis*acrylamide - CH~2~=CHCONH~2~: Acrylamide (bulk component) - CH~2~(NHCOCH=CH~2~)~2~: *bis*-acrylamide (cross linking agent) - If you increase the percentage of *bis*-acrylamide you get a closer, tighter structure and large proteins will not move as far within the gel - Free radical catalysis-addition of ammonium persulfate and N,N,N',N'-tetramethylenediamine (TEMED) that catalyses the decomposition of the persulfate ion to give a free radical e.g. S~2~O~8~^2-^ + e^-^ SO~4~^2-^ + SO~4~^-\*^ - Slight charge element in movement of proteins through polyacrylamide gels but it's mainly dominated by size - The more negative the protein the faster it runs - Separation gel at the bottom - Percentage of acrylamide and *bis*-acrylamide is selected based on known molecular weights of your gel - If you have very large proteins you use a lower percentage of *bis*-acrylamide - If you have very low molecular weight proteins you need a much higher concentration to get good resolution - 3 -- 10% acrylamide at top end if protein fragments are very small - Stacking gels: - Short gel on top of the main separating gel - Concentrates the sample into a sharp band before entering the main separating gel - Low concentration of acrylamide - Low ionic strength - pH near neutrality - Very large pore size -- Proteins move freely and quickly 'stack up' onto the interface with the main gel - pH 6.9 but resolving gel pH 8 -- 9 - Tris glycine pH 8 -- 9: - NH~3~^+^-CH~2~-COO^-^ Zwitterion - NH~2~-CH~2~-COO^-^ + H^+^ Anion - Buffered using glycine system - Cl^-^ ions carry the charge in the stacking gel, glycinate ions in the resolving gel - Na ions present when you generate the stacking gel are what are used as the main ion transporters in the top of the structure - Denaturing electrophoresis (SDS-PAGE) (PAGE = poly acrylamide gel electrophoresis): - Sodium dodecyl sulphate (SDS): CH~3~-(CH~2~)~10~-CH~2~OSO~3~^-^ Na^+^ - Anionic detergent binds to and denatures proteins - β-mercaptoethanol reduces disulphide bridges - Denaturing -- Migration is size-dependent - Non-denaturing -- Migration is size, shape and charge-dependent - Dithiothreitol and 2-mercaptoethanol are cheap, common denaturants - Dithiothreitol is actually quite difficult to separate from protein mixtures - Nowadays if you need to reisolate the protein you use a different compound called TCEP which is much more expensive - Native PAGE: - Apoferritin - 24 subunits - Self-assemble into a protein nanocage - Cages composed of different ratios of modified and unmodified apoferritins - Proteins are not denatured and retain tertiary and quaternary structure - Gradient SDS-PAGE: - Gradient: Increasing acrylamide concentration (decreasing pore size) - Proteins migrate until the pore size impedes further progress - Upon reaching the 'pore limit' migration does not change appreciably with time (does not cease completely) - Wider range of protein molecular weights can be separated - Better separation of similar size proteins - Complicated and difficult to setup - Isoelectric focusing (IEF): - Separation -- According to isoelectric point (pI) through a pH gradient - pI of a protein is the pH where it has no overall charge - Low % acrylamide gel in the presence of ampholytes (low MW synthetic polyamino-polycarboxylic acids) - Low % gel allows free movement through large pores - Ampholytes arrange themselves in order of increasing pI from the anode to the cathode under an electric field - Each ampholyte maintains a local pH corresponding to its pI, creating a uniform pH gradient across the gel - A protein will migrate through the gel until it reaches the region where its pI corresponds to the local pH - At this point the protein will have no net charge and will cease to move (trapped or focused) - High pI proteins are ones with lots of arginines, histidines and lysines in them - Low pI proteins are ones with lots of aspartates and glutamates relative to the number of asparagines and glutamines - 2-D electrophoresis: - Electrophoresis through 2 dimensions - 1^st^ dimension IEF (separation according to pI) - 2^nd^ dimension SDS-PAGE (separation according to size) Lecture 2 - Plasmids: - Those used in molecular biology are called vectors - They typically comprise a multiple cloning site (MCS) which is a short region with several restriction sites allowing easy insertion of DNA fragments (genes) and an antibiotic resistance gene for selection which can be introduced into bacteria through a process called transformation - Cell transformation: - Chemical transformation using a 0.1M \[Ca^2+^\] solution on ice to make competent cells followed by heat shock in presence of plasmid - Electroporation -- purchase electrocompetent cells and use an electroporator to disrupt the membrane (\>90% cells die) in presence of plasmid - Alternatives: Sonication, microwaves - The gene of interest (GOI) is cloned into a plasmid, the recombinant plasmid is introduced into bacteria and the bacteria are induced to produce large amounts of the corresponding protein - Only the successfully transformed cells containing the plasmid (with its antibiotic gene) grow -- only those that are white have GOI Restriction Enzyme Cut Site -------------------- ----------- BamH1 G↓GATCC EcoR1 G↓AATTC Hind3 A↓AGCTT Not1 GC↓GGCCGC - DNA ligases stick DNA back together by reforming phosphate backbone - DNA ligase uses energy from hydrolysis of 2 phosphate bonds from ATP to make new phosphodiester bond - Polymerase chain reaction: - Amplifying/copying the GOI - Reaction cycles: - Step 1 -- DNA melting - Step 2 -- Primer annealing - Step 3 -- Extension - Taq polymerase (Thermus aquaticus) -- High processivity, lower fidelity (no 3'-5' exonuclease activity), adds an extra A base at the 3'-end, 50% activity lost after 30 minutes at 95^o^C - Vent polymerase (Thermococcus litoralis) -- grows at 98^o^C in submarine thermal vents, less processive than Taq but higher fidelity (has 3'-5' exonuclease activity) and forms blunt-ended products, 10% activity lost after 60 minutes at 98^o^C **Neil Oldham** Lecture 1 - - Electrospray Ionisation (ESI) and Matrix Assisted Laser Desorption Ionisation (MALDI) allow the ionisation and transfer of large molecules (proteins etc.) into the gas-phase for mass measurement - MALDI: - Uses laser energy to desorb and ionise sample embedded in a crystalline matrix - The matrix: - Absorbs energy from the laser (usually UV) and transfers it to the sample causing desorption - Reacts with the sample in the gas phase, leading to proton exchange and ionisation - Singly charged ions are seen in the spectra -- even with large proteins such as bovine serum albumin (BSA), M.W. 66.5 kDa - ESI: - - Proteins show multiple charge states -- contrast with MALDI - Measuring the mass of a protein by MALDI or ESI is a good way of checking that the protein has the expected mass (e.g. in characterising a recombinant protein product expressed in E. coli) but it doesn't provide sequence information of unknown proteins - Protein sequencing: - The protein is digested by a protease to generate peptides - Trypsin is commonly used as the protease - Trypsin cleaves specifically at C-terminal side of lysine (K) and arginine (R) residues - LDFPQRILLWKAGGT would give LDFPQR, ILLWK and AGGT as peptide products - Even a relatively small protein like myoglobin can give a complex mixture of peptides - The peptide mixture can be analysed by liquid chromatography-ESI-MS (LC-ESI-MS) - Peptides are separated by chromatography and then the mass of each peptide is measured by ESI-MS - Sequence information can be obtained by fragmenting the peptide ions in the mass spectrometer (MS/MS) - A quadruple-time of flight MS (Q-TOF) is commonly used for this - Q1 is used to select the desired m/z peptide ion, which is fragmented in collision cell q2 - The product ions are then analysed in the TOF mass analyser - Collision induced dissociation (CID) of peptide ions (usually ^2+^ or ^3+^) results in cleavage about the peptide bond to produce N-terminal (a, b, c) and C-terminal (x, y, z) fragments - The subscript number shows how many AA residues the ion contains - B and y ions usually dominate - The difference between a pair of adjacent ions gives the mass and ID of the amino acid - Given a reasonable series of y or b ions, the peptide can be (partially) sequenced - Note it is not possible to distinguish Leu (113.08) and Ile (113.08); and Lys (128.10) and Gln (128.06) require high resolution accurate mass measurement (condensed mass) - As few as 6 or 7 contiguous amino acids from a single peptide can uniquely identify a protein (remember: this corresponds to 18 -- 21 DNA bases) - Can be used to identify and locate protein modification due to them causing a mass change to the modified amino acid Lecture 2 - Sedimentation velocity experiments are dependent of the protein shape and size - Analytical Ultracentrifugation (AUC): - Uses a high-speed centrifuge to cause sedimentation of biomolecules (proteins) - Two main sedimentation experiments: equilibrium and non-equilibrium - Provides information on molecular mass and interactions, either self-association or association with other molecules - - Absorbance -- protein solute absorbs light (e.g. UV), but solvent doesn't, so sedimentation may be monitored. Can be specific if a given protein absorbs at particular wavelengths due to e.g. aromatic AA residues, bound metal or cofactor (e.g. NAD^+^/H, FAD/H~2~ etc.) - Interference -- uses relative refractive index between sample and reference solution. Universal detection (does not need absorbing protein), but not specific - Fluorescence -- relatively recent introduction to AUC. Very sensitive and can be very specific (defined excitation and emission wavelengths) - Non-equilibrium sedimentation: - - Uses very fast rotor speeds (60,000 rpm) to measure sedimentation velocity - Means there is no back-diffusion of protein solute - Whilst undergoing sedimentation in AUC, solute molecules experience a combination of three forces: - Centrifugal force -- due to the spin of the centrifuge - Buoyancy force -- due to the solute displacing solvent molecules - Frictional force -- due to restriction of solute passing through solvent -   - Equilibrium sedimentation: - With slower rotor speeds (10,000 rpm) protein sedimentation is balanced by diffusion back up the cell - After a period of centrifugation, equilibrium is reached - This can take many hours - The technique can be used to estimate molecular weight M - This is now mainly superseded by mass spectrometry - In the example on the right, a 50 kDa protein is in a monomer-dimer equilibrium - At low concentration (absorbance) the protein is monomeric, whilst at high concentration (absorbance) it is dimeric - Surface Plasmon Resonance (SPR): - Light passing through a prism can undergo total internal reflection - If the upper surface of the prism is coated with a thin conductor layer (e.g. gold) electrons can oscillate through the conductor layer - These oscillations are called surface plasmons - At a certain angle (θ) of incident light, resonance between the light and the surface plasmons is achieved and the intensity of reflected light dips to a minimum (θ~SPR~) - θ~SPR~ is very sensitive to refractive index, so changes on the gold surface affect its value (change from 1 to 2) - In an SPR experiment the resonance angle theta depends on the refractive index of the sample - The upper gold surface is usually modified with reactive groups to allow proteins to be attached: usually exploiting nucleophilic AA sidechains on the protein such as Lys and Cys - By immobilising a protein on the upper surface of the gold and passing interacting molecules over the surface, binding can be detected and studied (as θ~SPR~ changes) - This is done in a flow cell - One example would be to attach antibodies to the gold surface and pass over potential target proteins (analytes) - Their binding to the antibody changes the refractive index of the system and hence θ~SPR~ - Pure analyte samples are required as the technique does not discriminate well between components in a mixture - Following injection of the analyte, association between the analyte and the immobilised protein occurs - Injection of pure buffer (with no analyte) induces dissociation of the complex - At the end of the experiment residual bound analyte is removed by injecting a regeneration buffer to strip the complex - Returning to the normal buffer resets the system for another cycle - Analysis of the sensorgram can provide kinetic and thermodynamic data about binding - The rate of association of analyte A and immobilised protein P is = k~1~\[A\]\[P\] where k~1~ is the association rate constant - The rate of dissociation of analyte A and immobilised protein P is = k~-1~\[AP\] where k~-1~ is the dissociation rate constant - The dissociation constant K~D~ = \[A\]\[P\] / \[AP\] = k~-1~ / k~1~ - Accurate in the nM to μM range Lecture 3 - Principle of fluorescence: - Absorption at one wavelength and emission at another (longer) wavelength caused by thermal loss of energy and accompanying change in vibrational energy state - The change between excitation and emission wavelengths caused by rapid thermal decay is known as the Stokes shift - Fraction of excited molecules that emit fluorescence known as quantum yield - Measurement of fluorescence: - Requires polarised chromophore (fluorophore) - Intrinsic protein fluorophores include aromatic AAs, especially tryptophan (indole ring) and e.g. flavin, NADH cofactors - Extrinsic fluorophores can be attached or bound to the protein - Extended exposure to intense excitation light can cause photobleaching (photochemical destruction of the fluorophore) - Use of fluorescence: - Fluorescence is very sensitive to the environment of the fluorophore: both in terms of λ (emission) and quantum yield (signal) - In a protein, a surface tryptophan residue exposed to water will have different fluorescence from one buried inside the protein core - This can be used to monitor protein folding - If no suitable tryptophan is present, an extrinsic fluorophore can be introduced chemically - If a fluorophore binds to a protein non-covalently, its λ (emission) and quantum yield (signal) can change significantly - The protein bovine serum albumin (BSA) binds many lipids as part of its role in transport through blood - The extrinsic fluorescent compound ANS binds to BSA causing fluorescence to change dramatically as it moves from the polar environment of water to the non-polar environment of the protein - Fluorescence anisotropy: - Anisotropy = different in different directions - Uses plane polarised light to selectively excite the fluorophore - Only when their fluorescence dipole is oriented with the plane of polarisation do they absorb - If the molecules are large, they tumble slowly in solution and emit light which is still highly polarised - If the molecules are small, they tumble rapidly between absorption and emission causing their emitted light to be depolarised (reduced anisotropy) - If a small fluorescent ligand binds to a large protein, the rate of tumbling will decrease significantly - This increases anisotropy (A) of the fluorescence relative to the ligand alone (A~0~) - Forster resonance energy transfer (FRET): - If two different fluorophores are present and one (the 'donor') is selectively excited by irradiation, its fluorescence can be quenched by the second ('acceptor') fluorophore if: - The emission spectrum of the donor overlaps with the excitation (absorption) spectrum of the acceptor - The donor and acceptor are sufficiently close in space - This phenomenon is called FRET - If donor and acceptor fluorophores are placed on different domains of a protein, FRET can be used to measure their relative motion and hence the conformational flexibility of a protein - In the example, the calcium binding protein undergoes a large conformational change on binding a helical peptide - The contraction can be measured by a substantial increase in FRET efficiency between the fluorophores - FRET can also be used to monitor dissociation of two linked fluorescent proteins - For example, a green fluorescent protein (GFP) is linked to a blue fluorescent protein (BFP) by a 25 AA peptide linker - The linker contains a trypsin cleavage site (Arg residue) - As the linker is cleaved, the two proteins diffuse apart and the FRET between them reduces Lecture 4 - Protein molecules are large, containing thousands of atoms making their NMR spectra very complex and difficult to interpret - Even 'small' proteins, such as lysozyme (14 kDa) give many overlapping signals in 1D ^1^H NMR - Protein NMR relies on 2D (and increasingly 3D) methods - NMR signals are correlated with each other to give a 2D plot - There are different types of 2D experiment, depending on: - Whether the coupled nuclei are the same or different (homo- or heteronuclear) - Whether the coupling is through bonds (scalar, J) or through space (dipolar, d) - Common homonuclear experiments include: - ^1^H-^1^H COSY (through bond) - ^1^H-^1^H NOESY (through space) - NOESY experiments exploit the nuclear Overhauser effect (nOe) between protons held rigidly in close proximity due to secondary structure - Patterns in ^1^H-^1^H NOESY between contiguous AAs can reveal the presence of an alpha helix or beta sheet - Heteronuclear experiments: - Provide more information about protein structure - Particularly important for larger proteins (\>100 AAs), where slow tumbling rates cause line broadening in ^1^H-^1^H experiments - Coupling between ^13^C, ^15^N and ^1^H is commonly examined, which usually requires ^13^C- and ^15^N-labelled proteins - Large coupling constants are often observed - The process of assigning signals in 2D NMR to specific AAs, then determining their spatial relationships is a complex process (even with knowledge of the sequence) - It requires considerable skill and time, but can result in a 3D structure - The process is performed in combination with molecular modelling - Once the signals in a 2D NMR spectrum are assigned it is possible to monitor how they change upon addition of a binding partner - By titrating in a 'binder' (small molecule ligand or another protein) changes in the chemical shifts can be seen, called chemical shift perturbation (CSP) - AA residues that show the greatest CSP are where the binder interacts and hence the binding site is mapped - If the protein is electrosprayed from a purely aqueous solvent containing ammonium acetate as a volatile buffer, the spectrum changes to give lower charge states (and fewer charge states) - This is because the protein is still folded when it is protonated and there is a smaller surface area to accept protons - The spectrum gives a measured mass of 17,566 and not 16,951 Da. Why? - Myoglobin has a non-covalently bound haem ligand (Fe binds oxygen) - The mass of the haem is 615 Da, the mass difference seen between the native and denatured spectra - Thus, the ligand is maintained in the binding pocket of the protein under native conditions - Native MS can be used to study protein-ligand complexes - Very large protein complexes can be studied by native MS - Using example of GroEL, an 800 kDa 14mer complex - The charge states appear high, they are, actually, relatively low for such a large mass - MS/MS can be used to break up the complex and examine individual subunits