Food Biotechnology (1110427) LECTURE NOTES PDF
Document Details
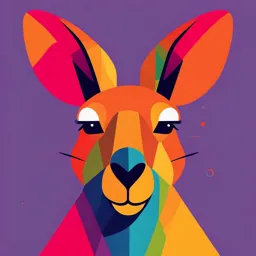
Uploaded by SatisfactoryHonor
Philadelphia University
Dr. Ghadder Othman
Tags
Summary
These lecture notes cover various aspects of food biotechnology, including concepts of macromolecules, the central dogma of molecular biology, transcription processes, gene expression, and recombinant DNA technology. The material is presented in a structured format suitable for an undergraduate-level course or further study.
Full Transcript
Food Biotechnology (1110427) Instructor: Dr. Ghadder Othman Department of clinical nutrition and dietetics Faculty of Allied Medical Sciences Chapter 2: Concepts of macromolecules: function and synthesis 1. The Central Dogma Of Molecular Biology Two essential fea...
Food Biotechnology (1110427) Instructor: Dr. Ghadder Othman Department of clinical nutrition and dietetics Faculty of Allied Medical Sciences Chapter 2: Concepts of macromolecules: function and synthesis 1. The Central Dogma Of Molecular Biology Two essential features of living creatures are the ability to reproduce their own genome and manufacture their own energy. In order to accomplish these feats, an organism must be able to make proteins using information encoded in its DNA. Proteins are essential for cellular architecture, giving the cell a particular shape and structure. 1. The Central Dogma Of Molecular Biology Proteins include enzymes that catalyze reactions used to make energy. Proteins control cellular processes like replication. Proteins provide channels in the membrane for cells to communicate with each other or share metabolites. Making proteins is a key operation for all living organisms. 1. The Central Dogma Of Molecular Biology The central dogma of molecular biology states that information flows from DNA to RNA to protein. First, RNA is made from DNA in a process called transcription. Next, messenger RNA are used to make protein in a process called translation. 2. Transcription Expresses Genes Gene expression involves making an RNA copy of information present on the DNA, that is, transcribing the DNA. Making RNA involves: a) Uncoiling the DNA, b) Melting the strands at the start of the gene, c) Making an RNA molecule that is complementary in sequence to the template strand of the DNA with an enzyme called RNA polymerase, d) Stopping at the end of the gene. e) The newly made RNA is released from the DNA, which then returns to its supercoiled form. 2. Transcription Expresses Genes An important issue in transcription is identifying the right gene. Which gene needs to be decoded to make protein? There are different types of genes. Some are housekeeping genes that encode proteins that are used all the time. Other genes are activated only under certain circumstances. For instance, in E. coli, genes that encode proteins involved with the utilization of lactose are expressed only when lactose is present. The same principle applies to the genes for using other nutrients. 2. Transcription Expresses Genes The final product encoded by a gene is often a protein but may be RNA. Genes that encode proteins are transcribed to give messenger RNA, which is then translated to give the protein. Other RNA molecules, such as tRNA, rRNA, and snRNA, are used directly (i.e., they are not translated to make proteins). Some RNA molecules, such as large-subunit rRNA, are called ribozymes and can catalyze enzymatic reactions 2. Transcription Expresses Genes The coding region of a gene is sometimes called a cistron or a structural gene and may encode a protein or a non-translated RNA. In contrast, an open reading frame (ORF) is a stretch of DNA (or the corresponding RNA) that encodes a protein and is not interrupted by any stop codons for protein translation. 2. Transcription Expresses Genes Every gene has a region upstream of the coding sequence called a promoter. RNA polymerase recognizes this region and starts transcription here. When a gene is transcribed all the time or constitutively, then the promoter sequence closely matches the consensus sequence. If the gene is expressed only under special conditions, activator proteins or transcription factors are needed to bind to the promoter region before RNA polymerase will recognize it. 2. Transcription Expresses Genes Just after the promoter region is the transcription start site. This is where RNA polymerase starts adding nucleotides. Between the transcription start site and the ORF is a region that is not made into protein called the 5 untranslated region (5 UTR). This region contains translation regulatory elements like the ribosome binding site. Next is the ORF, where no translational stop codons are found. Then there is another untranslated region after the ORF, known as the 3 untranslated region (3 UTR). Finally comes the termination sequence where transcription stops. 3. Making The RNA In bacteria, once the sigma subunit of RNA polymerase recognizes the promotor, the core enzyme forms a transcription bubble where the two DNA strands are separated from each other. The strand used by RNA polymerase is called the template strand (DNA template) and is complementary to the resulting mRNA. The core enzyme adds RNA nucleotides in the 5’ to 3’ direction, based on the sequence of the template strand of DNA. 3. Making The RNA The newly made RNA anneals to the template strand of the DNA via hydrogen bonds between base pairs. The opposite strand of DNA is called the coding strand. Because this is complementary to the template strand, its sequence is identical to the RNA. 4. Transcription Stop Signals RNA polymerase continues transcribing DNA until it reaches a termination signal. In bacteria, the Rho-independent terminator is a region of DNA with two inverted repeats separated by about six bases, followed by a stretch of A’s. As RNA polymerase makes these sequences, the two inverted repeats form a hairpin structure. The secondary structure causes RNA polymerase to pause. As the stretch of A’s is transcribed into U’s, the DNA/RNA hybrid molecule becomes unstable (A/U base pairs only have two hydrogen bonds). RNA polymerase “stutters” and then falls off the template strand of DNA in the middle of the A’s. 5. The Number Of Genes On An mRNA Varies In prokaryotes, the distance between genes is much smaller, and genes associated with one metabolic pathway are often found next to each other. For example, the lactose operon contains several clustered genes for lactose metabolism. Operons are clusters of genes that share the same promoter and are transcribed as a single large mRNA that contains multiple structural genes or cistrons. These transcripts are called polycistronic mRNA. 5. The Number Of Genes On An mRNA Varies In eukaryotes, genes are often separated by large stretches of DNA that do not encode any protein. In eukaryotes, each mRNA only has one cistron and is therefore called monocistronic mRNA. 6. Eukaryotic Transcription Is More Complex Eukaryotes have three different RNA polymerases that each transcribe different types of genes. 1. RNA polymerase I transcribes the eukaryotic genes for large ribosomal RNA. 2. RNA polymerase III transcribes the genes for tRNA, 5S rRNA, and other small RNA molecules. 3. RNA polymerase II transcribes the genes that encode proteins and has been studied the most. 6. Eukaryotic Transcription Is More Complex The layout of the eukaryotic promoter is much different. RNA polymerase II needs three different regions, the initiator box, the TATA box, and various upstream elements that bind proteins known as transcription factors. The initiator box is the site where transcription starts and is separated by about 25 base pairs from the TATA box. The upstream elements vary from gene to gene and aid in controlling what proteins are expressed at what time. 6. Eukaryotic Transcription Is More Complex 7. Eukaryotic mRNA Is Processed Before Making Protein Bacterial mRNA may be translated without any processing. Bacteria often start translating their mRNA while it is still being transcribed (known as coupled transcription/ translation). Eukaryotic RNA is processed in a variety of ways before it can leave the nucleus and be translated into protein. 7. Eukaryotic mRNA Is Processed Before Making Protein First, eukaryotic mRNA must have a cap added to the 5’ end of the message. The cap is a GTP added in reverse orientation and which is methylated on position 7 of the guanine base. Methyl groups may also be added to the first one or two nucleotides of the mRNA. 7. Eukaryotic mRNA Is Processed Before Making Protein The second modification of eukaryotic mRNA is adding a long stretch of adenines to the 3’ end—the poly(A) tail. A third modification made to eukaryotic mRNA is the removal of introns. Eukaryotic DNA contains many stretches of intervening sequence (introns) between regions that will ultimately code for a protein (exons). The mRNA is then transported from the nucleus to the endoplasmic reticulum for translation by ribosomes. 8. Translating The Genetic Code Into Proteins Messenger RNA provides the information a ribosome needs to make proteins. This process is known as translation because it involves translating information carried by nucleic acids to give the sequences of amino acids that make up proteins. Messenger RNA is therefore read in groups of three bases, known as triplets or codons. Each triplet of bases codes for one amino acid. Because there are more than 20 triplets, many are redundant so that multiple codons will be translated into the same amino acid. For example, valine is encoded by GUU, GUC, GUA, or GU 8. Translating The Genetic Code Into Proteins Small RNA molecules known as transfer RNA (tRNA) recognize the individual codons on mRNA and carry the corresponding amino acids. The anticodon consists of three bases complementary to those of the corresponding codon and it recognizes the codon by base pairing. The acceptor stem is where the amino acid is added to the free 3’ end of the tRNA 9. Protein Synthesis Occurs At The Ribosome The ribosome is a molecular machine that unites the mRNA with the appropriate tRNAs and then links the amino acids together into a chain. A ribosome consists of several RNA molecules (ribosomal RNA or rRNA) and many proteins. The larger subunit has three binding sites for tRNA, called A for acceptor, P for peptide, and E for exit, referring to the action occurring at each site. mRNA Translation (Advanced) - YouTube 9. Protein Synthesis Occurs At The Ribosome The ribosome cycle The process of translating mRNA consists of: a. Initiation, b. Elongation c. Termination. The ribosomal cycle, is essentially identical in all cases, despite differences in the sizes of ribosomal subunits between prokaryotes and eukaryotes 9. Protein Synthesis Occurs At The Ribosome I. Initiation Initiation requires a specific interaction between the 16 S rRNA of the small ribosomal subunit and the ribosomal binding site (RBS) or a Shine– Delgarno (SD) sequence of the message. In the initiation phase, prokaryotes and eukaryotes differ in the ways in which mRNA is bound and the initiation codons selected. In bacteria the initiation factors are known as IFs (IF-1, IF-2, IF-3, etc.); in eukaryotes they are termed eIF-1 and so on 9. Protein Synthesis Occurs At The Ribosome II. Elongation Elongation is the stage of translation in which the mRNA is decoded in the 5’-to-3’ direction and the polypeptide chain is synthesized starting at the amino terminus. This process is essentially similar in prokaryotic and eukaryotic cells. Elongation is a cyclic process, each turn of the cycle corresponding to the decoding of one triplet codon and to the addition to the growing polypeptide chain of one amino acid (AA) residue. Elongation requires proteins called elongation factors (EFs). 9. Protein Synthesis Occurs At The Ribosome The peptide bond forms between the terminal carboxyl group of the peptide and the α-amino group of the amino acid in the A site. The peptidyl transfer reaction requires neither accessory protein factors nor the expenditure of energy. Peptidyl-transfer is followed by a complex and incompletely understood series of reactions called translocation. The free tRNA molecule in the P site is released and the ribosome moves to the next codon of the mRNA, thereby moving peptidyl-tRNA from the A site to the P site and putting in register in the empty A site the next codon to be read. 9. Protein Synthesis Occurs At The Ribosome III. Posttranslational control After release from the ribosome, polypeptides may undergo a number of types of covalent modification. These modifications, which are necessary to form a fully functional protein, include limited proteolysis and proteolytic modification involved in zymogen activation and removal of signal peptides. In addition, polypeptides undergo chemical modification of amino acids by the addition of acetyl, methyl, phosphate, hydroxy, and carboxyl groups, as well as glycosylation and acylation. In fact, more than 140 modified amino acid residues have been isolated from proteins. 10. Roles of RNA Three major classes of cellular RNA: a. messenger RNA (mRNA), b. rRNA, c. and tRNA all involved in translation, but only mRNA carries the information that specifies the primary structure of proteins. 10. Roles of RNA: rRNA Ribosomes are the most abundant constituent of cells actively engaged in protein synthesis. Since about half the mass of ribosomes is RNA, ribosomal RNA (rRNA) is the most abundant type of cellular RNA, accounting for 75% of total RNA. Ribosomes possess a multitude of activities necessary for protein synthesis including peptidyltransferase activity, codon-directed binding of aminoacyl-tRNAs, binding mRNA, initiation, elongation and termination factors, and GTPase activity 10. Roles of RNA: tRNA Transfer RNAs (tRNAs), the smallest type (4 S), are formed in the cytoplasm and serve for the translation of the genetic code at the ribosome. tRNAs have several features in common. All are single chains containing between 73 and 93 ribonucleotides. Nearly all tRNAs have a cloverleaf structure. This Photo by Unknown Author is licensed under CC BY 10. Roles of RNA: mRNA The structure of a typical mRNA is essential for understanding the way in which ribosomes interact with mRNA. Part of the sequence of nucleotide bases consists of the coding region of the mRNA, which contains the codons corresponding to the amino acid sequence of the protein. The beginning of the sequence is an initiator codon (AUG), and the mRNA molecule also terminates in a noncoding sequence at the 5’ end, which is termed the leader sequence. 10. Roles of All cytoplasmic eukaryotic cellular mRNA molecules RNA: mRNA possess at the 5′ end a catabolite activator protein (CAP) structure that is involved in the interaction of mRNA with the ribosome. 11. Concepts of recombinant DNA technology Basic to all biotechnology research is the ability to manipulate DNA. First for recombinant DNA work, researchers need a method to isolate DNA from different organisms. recombinant DNA technology Through recombinant DNA technology it became possible to understand the organization, the expression, and the structure of genes, as well as to diagnose many inherited diseases. This technology is also creating unique industrial microorganisms able to synthesize valuable proteins and is finding a wide spectrum of uses in other manufacturing processes and agriculture. recombinant DNA technology rDNA technology was an outgrowth of basic research on three major scientific developments that occurred roughly at the same time. i. The first was the discovery and subsequent commercial supply of restriction endonuclease enzymes, which can be used to recognize and cut specific nucleotide sequences within the DNA molecule. ii. The second was the creation and the purification in high yield of artificial cloning vectors (i.e., plasmid DNA in which selectable marker and restriction sites are introduced artificially), iii. The third was the ability to achieve transformation – the introduction of DNA into E. coli, and subsequently to identify the transformants containing recombinant clones. recombinant DNA technology Cloning means to obtain, from among many millions of different DNA fragments, a colony of genetically identical cells that contain the DNA fragment of interest. The principle of the gene cloning technique is that genetic information carrying DNA is isolated from a donor organism and is cleaved by restriction endonuclease enzymes into individual pieces that create cohesive or sticky ends. The fragments of DNA are then joined to a suitable vector DNA using another enzyme, DNA ligase. The cohesive ends have complementary sequences and can thus base pair. recombinant DNA technology There are three possible routes in cloning a foreign DNA fragment: a) the protein route (shotgun cloning), b) the complimentary DNA (cDNA) route, c) the synthetic DNA route 11.1. DNA Isolation And Purification Isolating DNA from bacteria is the easiest procedure because bacterial cells have little structure beyond the cell wall and cell membrane. Bacteria such as E. coli are the preferred organisms for manipulating any type of gene because of the ease at which DNA can be isolated. E. coli maintain both genomic and plasmid DNA within the cell. Genomic DNA is much larger than plasmid DNA, allowing the two different forms to be separated by size. 11.1. DNA Isolation And Purification 1. To release the DNA from a cell, the cell membrane must be destroyed. 2. The intracellular components are separated from the remains of the outer structures by either centrifugation or chemical extraction. 3. Once the proteins are removed, the sample still contains RNA along with the DNA. The enzyme ribonuclease (RNase) digests RNA into ribonucleotides. Ribonuclease treatment leaves a sample of DNA in a solution containing short pieces of RNA and ribonucleotides. 4. When an equal volume of alcohol is added, the extremely large DNA falls out of the aqueous phase and is isolated by centrifugation. Extracting DNA from fruit - YouTube 11.2. Electrophoresis Separates DNA Fragments By Size Gel electrophoresis uses electric current to separate DNA molecules by size. Agarose Gel Electrophoresis - YouTube 11.3. Restriction Enzymes Cut DNA; Ligase Joins DNA Naturally occurring restriction enzymes or restriction endonucleases are the key to making DNA fragments. These bacterial enzymes bind to specific recognition sites on DNA and cut the backbone of both strands. The enzymes do not cut their own cell’s DNA because they are methylation sensitive, that is, if the recognition sequence is methylated, then the restriction enzyme cannot bind. 11.3. Restriction Enzymes Cut DNA; Ligase Joins DNA Bacteria produce modification enzymes that recognize the same sequence as the corresponding restriction enzyme. These methylate each recognition site in the bacterial genome. Therefore, the bacteria can make the restriction enzyme without endangering their own DNA. 11.3. Restriction Enzymes Cut DNA; Ligase Joins DNA Fragments are linked or ligated using DNA ligase. The most common ligase used is actually from T4 bacteriophage. Ligase catalyzes linkage between the 3’-OH of one strand and the 5’-PO of 4 the other DNA strand. Ligase is much more efficient with overhanging sticky ends, but can also link blunt ends much more slowly. 11.4. Plasmid vectors Plasmids are extrachromosomal, self-replicating, double-stranded DNA molecules that are often found in bacteria and some lower eukaryotes. Most are circular, but there are some linear ones. They are not essential for growth, but they confer some unusual properties on the cells that harbor them. These molecules are transmissible; that is, they have the ability to transfer themselves during conjugation. 11.4. Plasmid vectors An essential prerequisite for a successful practical application of gene manipulation is the availability of a suitable vector, which ensures replication and maintenance of both the vector DNA and the integrated foreign DNA. Cloning vectors serve primarily to amplify foreign DNA in the host cell, that is, to induce a large increase in the ratio of plasmid to chromosomal DNA. 11.5. Purpose of gene cloning the general strategy of gene cloning essentially comprises four stages. 1. Preparation of genome fragments To do this, the entire genome of the first organism is digested with a restriction endonuclease to produce a random mixture of fragments (≈4–10 kb). 2. Insertion into vector The vector is normally cut with the same restriction enzyme used to generate the chromosomal DNA fragments. Linearized vectors are incubated with DNA ligase, which covalently joins the DNA molecules. 11.5. Purpose of gene cloning 3. Transformation of host cell The resulting recombinants are introduced into the host cell by the process of transformation. In this process, the ligated plasmid mixture is introduced into a suspension of bacteria treated with a cold CaCl solution. After this treatment the 2 cells are mixed with the plasmid DNA that is to be introduced, given a brief heat shock, and allowed to recover. 11.5. Purpose of gene cloning 4. Selection of recombinant clones Only a few bacteria will take up the recombinant plasmid, and the problem then becomes how to select the bacteria that contain the insert (i.e., foreign) DNA from the vast majority of the bacteria that do not contain insert. In shotgun cloning, the insertional inactivation technique can be used. Suppose that DNA fragments were inserted at the BamHI site in plasmid pBR322. Since inserts at this site interrupt the gene (insertional inactivation) from expressing tetracycline resistance, cells containing recombinant plasmids will be ampicillin resistant and tetracycline sensitive, while cells without inserts will be resistant to both ampicillin and tetracycline. 12. DNA sequencing DNA Sequencing - 3D (youtube.com) There are two methods for sequencing DNA: a) chemical b) enzymatic 12. DNA sequencing Two different methods for rapid and efficient sequencing of DNA are the Maxam–Gilbert and Sanger techniques, which were invented in the 1970s. Both have the same basic principle: the generation of a series of DNA fragments having a common starting point but variable termini. 12. DNA sequencing The Sanger DNA sequencing method is usually used for sequencing extensive domains of DNA. Sequencing of a purified single-stranded fragment obtained from vector M13 is considered to be easy and is accomplished by generating a series of complementary fragments of DNA by incubating the fragment with DNA polymerase (Klenow), a primer (a short piece of DNA), templates, the four normal deoxynucleotide triphosphates (one of which is radioactive), and one of the four dideoxynucleotide triphosphates (e.g., dideoxythymidine triphosphate). 13. Polymerase chain reaction (PCR) The polymerase chain reaction (PCR) is a new, powerful (in vitro) method for amplifying a small amount of DNA (a specific DNA fragment as small as 50–100 bp) several millionfold in a few hours. This technique in a way mimics the in vivo DNA replication in that the number of DNA molecules generated by the PCR doubles after each cycle. 13. Polymerase chain reaction (PCR) This method repeats a set of three steps: 1. Denaturing the DNA, 2. Annealing the primers to their complementary sequences, 3. Extending the annealed primers with a DNA polymerase 13. Polymerase chain reaction (PCR) 13. Polymerase chain reaction (PCR) I. In denaturation: a double-stranded DNA sample (the template) is heated at nearly 100 ∘C, the two strands separate and remain in solution until the temperature is cooled (annealed) in the presence of two primers. II. In primer annealing the separated strands are cooled in the presence of two short primers (≈20 bases each). III. Next DNA polymerase mediates the 5’ -to-3’ extension of the ′ ′ primer–template complex. (i.e., the pairs of synthetic oligonucleotides that anneal to sites flanking the region to be amplified). 13. Polymerase chain reaction (PCR) Thermostable Taq DNA polymerase is isolated from the thermophilic bacteria Thermus aquaticus. Taq polymerase is stable enough to survive up to 30 cycles, which give about a billionfold amplification. Running more than 30 cycles often leads to accumulation of unspecified product and does not increase the yield of specific amplifications. Thus, the technique does amplify the target DNA faster than the bacteria can multiply themselves. This development has led to the automation of the PCR by a variety of simple temperature-cycling devices. 13. Polymerase chain reaction (PCR) Real-time polymerase chain reaction (RT PCR) has emerged as a method of choice for food analysis due to its rapid and sensitive species identification capabilities, especially since RT-PCR can be performed when target concentrations are very low. 13. Polymerase chain reaction (PCR) Multiplex PCR: Whereas standard PCR usually uses one pair of primers to amplify a specific sequence, multiplex PCR allows the simultaneous amplification of more than one target sequence in a single reaction by using a set of primers This saves considerable time and effort, and decreases the number of reactions that need to be performed to detect the desired targets in the sample. For multiplex PCR, primers should be chosen with similar annealing temperatures. 13. Cloning, expression, and production of bovine chymosin (rennet) in yeast K. lactis Rennet (chymosin; EC 3.4.23.4) is a complex of enzymes produced in any mammalian stomach and is often used in the production of cheese. Rennet contains many enzymes, including a proteolytic enzyme (protease) that coagulates the milk, causing it to separate into solids (curds) and liquid (whey). They are also very important in the stomach of young mammals as they digest their mothers’ milk. 13. Cloning, expression, and production of bovine chymosin (rennet) in yeast K. lactis Rennet (chymosin) enzyme secreted by cells lining the stomach in mammals is responsible for clotting milk. It acts on a soluble milk protein (kappa-casein), which it converts to the insoluble form casein. This ensures that milk remains in the stomach long enough to be acted on by protein-digesting enzymes. 13. Cloning, expression, and production of bovine chymosin (rennet) in yeast K. lactis Expensive production costs of microbial and animal rennet made many producers sought further replacements of rennet by fermentation produced chymosin (FPC). With the development of genetic engineering, it became possible to insert animal genes into certain bacteria, fungi or yeasts to make them produce chymosin during fermentation. The genetically modified yeasts or fungi are killed after fermentation and chymosin isolated from the fermentation broth, so that the FPC used by cheese producers does not contain any genetically modified component or ingredient 13. Cloning, expression, and production of bovine chymosin (rennet) in yeast K. lactis FPC contains the identical chymosin as the animal source, but produced in a more efficient way. FPC products have been on the market since 1990 and have been considered in the past 20 years the ideal milk-clotting enzyme. By 2008, approximately 80–90% of commercially made cheeses in the United States and Britain were made using FPC. 13. Cloning, expression, and production of bovine chymosin (rennet) in yeast K. lactis Today, the most widely used FPC is produced either by the fungus A. niger and commercialized under the trademark CHY-MAX® by the Danish company Chr. Hansen, or by Kluyveromyces lactis and commercialized under the trademark MAXIREN® by the Dutch company DSM. The chymosin gene was inserted into the K. lactis chromosome and the yeast is grown by fed-batch fermentation. 13. Cloning, expression, and production of bovine chymosin (rennet) in yeast K. lactis After fermentation, the yeast is killed by addition of benzoic acid and the chymosin is isolated by filtration. Cheeses produced with FPC can be certified Koshen and Halal, and are suitable for vegetarians if there was no animal-based alimentation used during the chymosin production in the fermenter.