Chapter 9 - Muscle PDF
Document Details
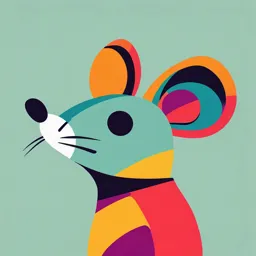
Uploaded by ProdigiousDobro
University of Toronto
Tags
Summary
This document provides an overview of muscle structure and function. The document details the different types of muscle, their components, and the process of muscle contraction and relaxation.
Full Transcript
Chapter 9: Skeletal Muscle Objectives To understand : Muscle structure and function- gross and micro levels Key muscles proteins of the sarcomere excitation and contraction, relaxation Cross bridge formation Force generation, recruitment, Length tension, force veloc...
Chapter 9: Skeletal Muscle Objectives To understand : Muscle structure and function- gross and micro levels Key muscles proteins of the sarcomere excitation and contraction, relaxation Cross bridge formation Force generation, recruitment, Length tension, force velocity Fibre types- characteristics Sensory aspects, reflexes Muscle Contraction CNS:Brain and Spinal Cord Peripheral Nervous System 1. Afferent 2. Efferent a) Somatic b) autonomic 1) sympathetic 2) parasympathetic Figure 9.39a Cardiac Muscle (a) Light micrograph of cardiac muscle tissue 4 Figure 12.1 The three types of muscles MultiNucleated Intercalated Figure 9.1 Appearance of Skeletal Muscle As Seen With Light Microscopy (Top) and In Schematic Form (Bottom) 6 Structure of Skeletal Muscles Connective tissue components a. Skeletal muscles are surrounded by a fibrous epimysium. b. Connective tissue called perimysium subdivides the muscle into fascicles. c. Each fascicle is subdivided into muscle fibers (myofibers) surrounded by endomysium. Epimysium Endomysium Perimysium Fibres digested away Figure 9.2 Structure of a Skeletal Muscle, a Single Muscle Fiber, and Its Component Myofibrils Muscle Bundles Fibres Myofibrils Sarcomeres Thick/thin Filaments MHC/actin 8 Figure 9.3 Structure of Thick and Thin Filaments 9 Figure 9.4 High Magnification of a Sarcomere and a Diagrammatic View of the Thick and Thin Filaments Aligned with the Sarcomere 2.6 u 1.6 u (a) Marion L. Greaser, University of Wisconsin (a) Electron micrograph of a sarcomere (b) Detailed illustration of sarcomere structure 10 Figure 9.5 Hexagonal Arrangements of the Thick and Thin Filaments in the Overlap Region in a Single Myofibril 3D 6:1 11 Figure 9.6 Transverse Tubules and Sarcoplasmic Reticulum in a Single Skeletal Muscle Fiber 12 Figure 9.7 Motor Units and the Muscle Fibers They Innervate (a) Single motor unit (b) Two motor units 13 Figure 9.8a Scanning Electron Micrograph Showing Branching of Motor Neuron Axons, With Axon Terminals Embedded in Grooves in the Muscle Fiber’s Surface (a) SEM of neuromuscular junction 14 Figure 9.8b Structure of a Neuromuscular Junction Showing the Junctional Folds of the Motor End Plate (b) Detailed illustration of section through a neuromuscular junction 15 Figure 9.9 Events at the Neuromuscular Junction That Lead to an Action Potential in the Muscle Fiber Plasma Membrane 16 Figure 9.12 Release and Uptake of Ca2+ by the Sarcoplasmic Reticulum During Contraction and Relaxation of a Skeletal Muscle Fiber 17 Figure 9.12 Release and Uptake of Ca2+ by the Sarcoplasmic Reticulum During Contraction and Relaxation of a Skeletal Muscle Fiber 18 Figure 9.12 Release and Uptake of Ca2+ by the Sarcoplasmic Reticulum During Contraction and Relaxation of a Skeletal Muscle Fiber 19 Figure 9.11 Activation of Cross-bridge Cycling by Ca2+ a) Low cytosolic Ca2+, relaxed muscle b) High cytosolic Ca2+, activated muscle 20 Figure 9.13 Cross-Bridges in the Thick Filaments Bind to Actin in the Thin Filaments and Undergo a Conformational Change that Propels the Thin Filaments Toward the Center of a Sarcomere 21 Figure 9.14 Sliding of Thick Filaments Past Overlapping Thin Filaments Shortens the Sarcomere With No Change in Thick or Thin Filament Length 22 Figure 9.15 Chemical and Mechanical Representations of the Four Stages of a Cross-Bridge Cycle 23 Figure 9.10 Time Relationship Between a Skeletal Muscle Fiber Action Potential and the Resulting Contraction and Relaxation of the Muscle Fiber From neuron at muscle fibre 24 Figure 9.18 Velocity of Skeletal Muscle Shortening and Lengthening as a Function of Load Switch axes 25 Force Velocity Curve At any given velocity the Trained can apply more force Against any given load the Trained can move (an object) faster Trained Untrained Force (load) Trained Untrained Velocity Figure 9.19 Summation of Isometric Contractions Produced by Shortening the Time Between Stimuli 27 Figure 9.20 Isometric Contractions Produced by Multiple Stimuli (S) at 10 Stimuli Per Second (Unfused Tetanus) and 100 Stimuli Per Second (Fused Tetanus), as Compared With a Single Twitch 28 Figure 9.21b Measurement of Passive (Elastic) and Active Tension in a Skeletal Muscle Fiber length-tension relationship 29 Figure 9.24 Immunohistological Staining of a Cross-Sectional Area of a Skeletal Muscle Showing the Three Fiber Types Found in Humans Blue Cells : Type 1 fibers Green Cells : Type 2A fibers Black Cells : Type 2X fibers 30 Identifying Proteins in Muscle Fluorescent tag Epitope (specific shape) 2°Antibody (Binds 1°Antibody to 1°) (Binds to epitope) Figure 9.22 The Three Sources of ATP Production During Muscle Contraction 32 Figure 9.23 Muscle Fatigue During a Maintained Isometric Tetanus and Recovery Following a Short Period of Rest 33 Figure 9.25 The Rate of Fatigue Development in the Three Fiber Types 34 Type I Tension 0 10 20 30 40 50 60 Time (min) Type Ila Tension 0 10 20 30 40 50 60 Tension Time (min) 0 10 20 30 40 50 60 Type Ilx Time (min) Table 9.3 Characteristics of the Three Types of Skeletal Muscle Fibers Slow-Oxidative Fibers Fast-Oxidative Glycolytic Fast-Glycolytic Fibers (Type 1) Fibers (Type 2A) (Type 2X)* Primary source of ATP Oxidative phosphorylation Oxidative phosphorylation Glycolysis production Mitochondria Many Intermediate Few Capillaries Many Many Few Myoglobin content High (red muscle) High (red muscle) Low (white muscle) Glycolytic enzyme activity Low Intermediate High Glycogen content Low Intermediate High Rate of fatigue Slow Intermediate Fast Myosin-ATPase activity Low Intermediate High Contraction velocity Slow Fast Fastest Fiber diameter Small Large Large Size of motor neuron Small Intermediate Large innervating fiber OX Intermediate GLY * Some muscle fibers found in the head and neck do not fit neatly into these categories, including some that control movements of the eye, middle ear bones, larynx, and jaw. 36 Figure 12.17 Motor units Figure 9.26 (a) Diagram of a Cross Section Through a Muscle Composed of Three Types of Motor Units; (b) Tetanic Muscle Tension Resulting From the Successive Recruitment of the Three Types of Motor Units (a) Illustration of a cross section of a muscle showing motor (b) Successive recruitment of motor unit types units 38 Muscle Fibre Type Recruitment Endurance (Jog) At Rest Resistance (Sprinting/Weights) Schematics of Motor Units in Skeletal Muscle Figure 12.14 Fast-twitch and slow-twitch muscles What about diffusion into or from Each Fibre type? Major Types of Muscle Fibers Classified based on differences Three major types – Slow-oxidative (type I) fibres – Fast-oxidative (type IIa) fibres – Fast-glycolytic (type IIx) fibres Biochemical Myosin Heavy Chain protein Metabolic (ATPase) Energy System Twitch Metabolic Provides ATP Speed of Force Generation Chemical Energy to Mechanical Energy Physiological FROM THE NEURON PERSPECTIVE- MOTOR Anatomy Motor Unit Size FROM THE FIBRE PERSPECTIVE Immuno- Biochem (Histochem) x FROM THE CONTRACTILE PERSPECTIVE Physiology Table 12.2 Characteristics of Muscle Fiber Types Blank Slow-Twitch Oxidative; Red Fast-Twitch Oxidative-Glycolytic; Fast-Twitch Glycolytic; White Muscle (Type I) Red Muscle (Type IIA) Muscle (Type IIB/IIX) Speed of Development of Slowest Intermediate Fastest Maximum Tension Myosin ATPase Activity Slow Fast Fast Diameter Small Medium Large Contraction Duration Longest Short Short -ATPase 2+ Activity in SR Moderate High High Ca Endurance Fatigue resistant Fatigue resistant Easily fatigued Use Most used: posture Standing, walking Least used: jumping; quick, fine movements Metabolism Oxidative; aerobic Glycolytic but becomes more Glycolytic; more anaerobic than oxidative with endurance training fast-twitch oxidative-glycolytic type Capillary Density High Medium Low Mitochondria Numerous Moderate Few Color Dark red (myoglobin) Red Pale (White) (always in the middle) Comparison of Rates to Reach Maximum Tension a = Type Ilx b = Type Ila c = Type I Peak Tension/Fiber Area Sizes Specific/normalized Time to Type II Type I (fast) Peak Tension (slow) (TPT) vs In vivo /reality 0 30 75 Time (msec) Muscle Disorders Have Multiple Causes Muscle cramp – Sustained painful contraction Overuse – Fatigue or soreness (DOMS, Rhabdomyolysis) Disuse leads to atrophy Acquired disorders Inherited disorders – Duchenne muscular dystrophy Absence of dystrophin – McArdle’s disease Myophosphorylase deficiency Glycogen not converted to glucose 6-phosphate Figure 9.16 Contractions SHORTENING Isometric LENGTHENING Winner Tie Loser WORK, Movement, Resistance, Damage 47 CONTRACTION TYPES Isometric -tetanus Square wave L- above > S- below < Muscle Damage and Repair 1. Skeletal muscles have stem cells called satellite cells (SCs) located near muscle fibers. 2. SCs divide, and fuses to: a) damaged muscle cells …..repair b) fuse to each other to form new muscle fibers. Satellite Cells Activation and WHEN A FIBRE IS INJURED/DAMAGED. Proliferation of Resting Myofibre Satellite Cells Quiescent Satellite Cell Myotrauma myonuclei Self-renewal Fusion to damaged Chemotaxis Regenerated myofibres to injured fibre myofibre with (hypertrophy) central nuclei Fusion to Produce new myofibres (hyperplasia) From Hawke and Garry, 2001 Creatine Kinase 1. Creatine kinase (CK), also called creatine phosphokinase (CPK), is an enzyme measured in blood samples to aid the diagnosis of such conditions as myocardial infarction (heart attack), muscular dystrophy, rhabdomyolysis, and others. 2. Rhabdomyolysis, which can produce a greatly increased concentration of CK in the blood, refers to skeletal muscle damage producing muscle weakness and pain. Different drugs, various forms of trauma, and certain toxins may cause rhabdomyolysis. 3. If necessary for diagnosis, laboratories can test for different isoenzymatic forms of CK. For example, damaged skeletal muscles release the CK-MM isoenzyme, whereas damaged heart muscle releases the CK-MB isoenzyme. Muscle Disorders Have Multiple Causes Muscle cramp – Sustained painful contraction Overuse – Fatigue or soreness (DOMS, Rhabdomyolysis) Disuse leads to atrophy Acquired disorders Inherited disorders – Duchenne muscular dystrophy Absence of dystrophin – McArdle’s disease Myophosphorylase deficiency Glycogen not converted to glucose 6-phosphate Muscular Dystrophy (DMD) Duchenne Muscular Dystrophy a. Duchenne muscular dystrophy (DMD) accounts for half of the more than 30 genetically different muscular dystrophies and is the most severe form. This disease is caused by mutations in a recessive gene located on the X chromosome. b. The gene codes for a protein called dystrophin. The dystrophin protein is located just under the sarcolemma where it provides support by bridging the cytoskeleton and myofibrils in the muscle fiber with the extracellular matrix. c. Mutations result in activity-induced damage to the sarcolemma that cannot be repaired by the muscle satellite cells and this causes muscle fiber necrosis and replacement by fibrous connective and fatty tissue. Figure 9.31 (a) Schematic Diagram Showing Costamere Proteins That Link Z Disks with Membrane and Extracellular Matrix Proteins, and (b) Boy with Duchenne Muscular Dystrophy (a) Location of costamere proteins (b) Standing in a body with Duchenne muscular dystrophy 56 Duchenne Muscular Dystrophy X-chromosome linked genetic disorder Mutation or deletion on gene which encodes for dystrophin Leads to progressive muscular weakness Typically by 12 years of age the patient is wheelchair dependent 22 + X 22 + X 22 + Y 44 + XY Other Muscle Diseases Myasthenia Gravis a. Myasthenia gravis an autoimmune disease caused by antibodies that block the nicotinic ACh receptors, particularly in the motor end plates (postsynaptic membranes) of skeletal muscle cells. b. This produces muscle weakness, especially in the eyes, eyelids, and face. c. Neostigmine and related drugs, which block the enzyme that degrades ACh (discussed shortly) in the synaptic cleft, can help treat symptoms. = antibody (size not to scale ) EM – Ultra structure NADH STAIN Add NADH and a substrate - wait 30 min- cell enzymes use it produce a change in colour. Explain why some are darker than others? (Hint: amount enzyme) Trends? Summary Muscle structure and function Components/proteins of the sarcomere Excitation and contraction, relaxation Cross bridge formation Force generation, recruitment Twitch, summation, tetanus, NMJ Motor Units, damage, satellite cells Length tension, force velocity Characteristics of the various fibre types- Diseases: DMD, MG Clinical Case Study A 17-year-old boy was undergoing a procedure to repair a fractured jaw. He received lidocaine (a local anesthetic) and sevoflurane (an inhaled general anesthetic). An hour into the procedure the anesthesiologist noticed that the patient’s face was red and beads of sweat were forming on his forehead. The patient’s heart rate had almost doubled, and his body temperature and exhaled CO2 concentration had increased. The oral surgeon reported that the patient’s jaw muscles had gone rigid. The patient was exhibiting all of the signs of malignant hyperthermia, a rare but potentially deadly condition. Malignant hyperthermia can lead to depletion of ATP, increased CO2 production, acidosis, elevated body temperature, flushing of the skin, and rapid breakdown of muscle tissue (rhabdomyolysis). Most patients who develop malignant hyperthermia have inherited a mutation of a gene that encodes the ryanodine receptors in skeletal muscle fibers, causing them to malfunction under certain circumstances, such as general anesthesia. What is the role of ryanodine receptors in skeletal muscle contraction? What are the consequences of a massive release of Ca2+ from the sarcoplasmic reticulum into cytoplasm in skeletal muscle fibers? 65 Types of Muscle Tissue There are 3 types of muscle ion the human body, each with it own specific characteristics and functions: Skeletal muscle: Attached to bone; contraction supports and moves skeleton; contractions are initiated by action potentials in motor neurons; voluntary Smooth muscle: Found in walls of hollow organs and tubes; contraction supports internal movements; controlled by autonomic nervous system, hormones, other signals; involuntary Cardiac muscle: Found in the heart; contraction propels blood through circulatory system; regulated by autonomic nervous system, hormones, other signals; involuntary 66 Characteristics of a Skeletal Muscle Fiber Characteristics of a skeletal muscle cell (muscle fiber): – Striated: striped appearance due to light and dark bands, originating in banding pattern of microscopic myofilaments Multi-nucleated Contains many mitochondria Consists of myofibrils, which are composed of sarcomeres Contains transverse (T) tubules to relay electrical messages into sarcoplasmic reticulum, which stores calcium for contraction Sarcolemma = plasma membrane Sarcoplasm = cytoplasm 67 Connective Tissue Structure A muscle contains a number of skeletal muscle fibers bound together by connective tissue. Skeletal muscles are attached to bones by bundles of connective tissue consisting of collagen fibers called tendons. 68 Myofibrils – Thin and thick filaments are arranged in cylindrical bundles called myofibrils, which are approximately 1 to 2 micrometers in diameter and extend from one end of the muscle fiber to the other. – The arrangement of thin and thick filaments along myofibrils gives skeletal and cardiac muscle fibers their characteristic striated appearance. 69 Composition of Thick Filaments – Thick filaments are composed mainly of the protein myosin. – A myosin molecule is composed of two large polypeptide heavy chains, which are intertwined to form a tail that lies along the axis of the thick filament. – At the end of the heavy chains, two globular heads extend out to the sides, forming cross-bridges, which make contact with the thin filament and exert force during muscle contraction. – Each globular head contains two light chains and two binding sites, one for attaching to the thin filament and one for ATP – The ATP binding site functions as an enzyme called myosin-ATPase 70 Composition of Thin Filaments – Thin filaments are composed mainly of the protein actin, as well as a protein called nebulin that is thought to play a role in thin filament assembly, and two other proteins – troponin and tropomyosin– that have important functions in regulating contraction. – An actin molecule is a globular protein composed of a polypeptide that polymerizes with other actin monomers to form a polymer made up of two intertwined, helical chains. – These chains make up the core of a thin filament. – Each actin molecule contains a binding site for myosin. 71 Other Myofibril Structures The sarcoplasmic reticulum (SR) in a muscle fiber is homologous to the endoplasmic reticulum found in most cells. At the end of each SR segment are two enlarged regions, the terminal cisternae, that are connected to each other by a series of smaller tubular elements. The presence of the Ca2+ binding protein calsequestrin in the terminal cisternae allows the storage of a large quantity of Ca2+ without having to transport it against a large concentration gradient. A transverse (T-) tubule lies directly between the terminal cisternae of adjacent segments of the SR. T-tubules are continuous with the sarcolemma, and the lumen of the T- tubule is continuous with the extracellular fluid surrounding the muscle fiber. Action potentials propagating along the plasma membrane travel to the interior of the muscle fiber via the T-tubules. 72 Molecular Mechanisms of Skeletal Muscle Contraction The term contraction does not necessarily mean “shortening” in muscle physiology. It refers to activation of cross-bridges, the force- generating sites within muscle fibers. For example, holding a dumbbell steady with your elbow bent requires muscle contraction, but not muscle shortening. Following contraction, the mechanisms that generate force are turned off and tension declines, allowing relaxation of muscle fibers. 73 Motor Units – A motor unit is defined as a motor neuron and all of the skeletal muscle fibers it innervates. – A single motor neuron innervates many muscle fibers, but each muscle fiber is controlled by a branch from only one motor neuron. – When an action potential occurs in a motor neuron, all the muscle fibers in its motor unit are stimulated to contract. – A whole muscle contains many motor units. 74 The Neuromuscular Junction 1 Stimulation of the neurons to a skeletal muscle is the only mechanism by which action potentials are initiated in this type of muscle. The neurons whose axons innervate skeletal muscle fibers are known as alpha motor neurons (or simply as motor neurons). Their cell bodies are located in the brainstem and the spinal cord. The axons of motor neurons are myelinated and are the largest- diameter axons in the body. They propagate action potentials at high velocities, allowing signals from the central nervous system to travel to skeletal muscle fibers very quickly. 75 The Neuromuscular Junction 2 The axon terminals of a motor neuron contain vesicles similar to the vesicles found at synaptic junctions between two neurons. The vesicles contain the neurotransmitter acetylcholine (ACh). The region of the muscle fiber plasma membrane that lies directly under the terminal portion of the axon is known as the motor end plate. The junction of an axon terminal with the motor end plate is known as a neuromuscular junction. 76 The Neuromuscular Junction 3 All neuromuscular junctions are excitatory. In addition to receptors for ACh, the synaptic junction contains the enzyme acetylcholinesterase, which breaks down ACh, just as it does at ACh-mediated synapses in the nervous system. 77 Disruption of Neuromuscular Signaling 1 Curare is a deadly arrowhead poison used by some indigenous peoples of South America, which binds strongly to nicotinic ACh receptors. It does not open their ion channels, and is not decomposed by acetylcholinesterase. When a receptor is occupied by curare, ACh cannot bind to the receptor. Although the motor neurons still conduct normal action potentials and release ACh, there is no resulting end plate potential (EPP) in the motor end plate and no contraction. Because the skeletal muscles responsible for breathing, like all skeletal muscles, depend upon neuromuscular transmission to initiate their contraction, curare poisoning can cause death by asphyxiation. 78 Disruption of Neuromuscular Signaling 2 Neuromuscular transmission can also be blocked by inhibiting acetylcholinesterase. Some organophosphates, which are the main ingredients in certain pesticides and “nerve gases” (the latter developed for chemical warfare), inhibit this enzyme. The result is skeletal muscle paralysis and death from asphyxiation. Nerve gases also cause ACh to build up at muscarinic synapses, where parasympathetic neurons inhibit cardiac pacemaker cells. The antidote for organophosphate and nerve gas exposure includes both pralidoxime, which reactivates acetylcholinesterase, and the muscarinic receptor antagonist atropine. 79 Disruption of Neuromuscular Signaling 3 Drugs that block neuromuscular transmission are sometimes used in small amounts to prevent muscular contractions during certain types of surgical procedures. For example, Succinylcholine acts as an agonist to the ACh receptors and produces a depolarizing/desensitizing block similar to acetylcholinesterase inhibitors. Nondepolarizing neuromuscular junction blocking drugs that act more like curare and last longer are also used, such as rocuronium and vecuronium. The use of such paralytic agents in surgery reduces the required dose of general anesthetic, allowing patients to recover faster and with fewer complications. Patients must be artificially ventilated, however, to maintain respiration until the drug has been cleared from their bodies. 80 Disruption of Neuromuscular Signaling 4 The toxin produced by the bacterium Clostridium botulinum blocks the release of acetylcholine from axon terminals. Botulinum toxin is an enzyme that breaks down proteins of the SNARE complex that are required for the binding and fusion of ACh vesicles with the plasma membrane of the axon terminal. This toxin, which produces the food poisoning called botulism, is one of the most potent poisons known. Application of botulinum toxin is increasingly being used for clinical and cosmetic procedures, including the inhibition of overactive extraocular muscles, prevention of excessive sweat gland activity, treatment of migraine headaches, and reduction of aging-related skin wrinkles. 81 Excitation-Contraction Coupling Excitation-Contraction Coupling: Sequence of events in which an action potential in the motor end plate of a muscle fiber causes actin-myosin crossbridge formation and contraction of the muscle Action potentials in the muscle fibers conduct their messages into the muscle fibers through the Transverse (T) Tubules. These, in turn, initiate Ca2+ release from its storage site in the lateral sacs of the Sarcoplasmic Reticulum. Ca 2+ ions are then available for binding to Troponin and initiating the events of the Sliding Filament Mechanism. Action potentials cause Ca2+ release from SR, which leads to increased 2+ concentration in the vicinity of the actin & myosin, and finally, to Ca muscle contraction. At the end of an action potential, Ca -ATPase pumps return 2+ Ca2+ ions to the SR by Active Transport; this leads the to decreased Ca2+ concentration around actin & myosin, which results in muscle relaxation. 82 The Thin Filament Proteins: Troponin and Tropomyosin 1 Thin filaments are mainly composed of the protein actin. Tropomyosin is a rod-shaped molecule composed of two intertwined polypeptides. Chains of tropomyosin molecules are arranged end to end along the actin thin filament. These molecules partially cover the myosin-binding site on each actin monomer, thereby preventing the cross-bridges from making contact with actin. Each tropomyosin molecule is held in this blocking position by the smaller globular protein, troponin. 83 The Thin Filament Proteins: Troponin and Tropomyosin 2 Troponin, which interacts with both actin and tropomyosin, is composed of three subunits designated by the letters I (inhibitory), T (tropomyosin- binding) and (Ca2+ -binding). One molecule of troponin binds to each molecule of tropomyosin and regulates the access to myosin-binding sites on the seven actin monomers in contact with that tropomyosin. In a resting muscle fiber; troponin and tropomyosin cooperatively block the interaction of myosin cross-bridges with the thin filament. binding of Ca2+ causes a change in the shape of troponin, which The relaxes its inhibitory grip and allows tropomyosin to move away from the myosin-binding site on each actin molecule. Conversely, the removal of Ca2+ from troponin reverses the process, turning off contractile activity. 84 Mechanism of Cytosolic Increase in Ca2+ The T-tubules are in intimate contact with the terminal cisternae of the sarcoplasmic reticulum. This junction involves two integral membrane proteins, one in the T-tubule membrane, and the other in the membrane of the sarcoplasmic reticulum. The T-tubule protein is a modified voltage-sensitive Ca2+ channel known as the dihydropyridine (DHP) receptor, which acts as a voltage sensor. The protein embedded in the sarcoplasmic reticulum membrane is known as the ryanodine receptor, which forms a Ca2+ channel. During a T-tubule action potential, charged amino acid residues within the D HP receptor protein induce a conformational change, which pulls open the ryanodine receptor channel. Ca2+ is then released from the terminal cisternae of the sarcoplasmic reticulum into the cytosol. 85 Sliding-Filament Mechanism When force generation produces shortening of a skeletal muscle fiber, the overlapping thick and thin filaments in each sarcomere move past each other, propelled by movements of the cross-bridges. During this shortening of the sarcomeres, there is no change in the lengths of either the thick or thin filaments, only in their amount of overlap. This is known as the sliding-filament mechanism of muscle contraction. 86 Table 9.1 Functions of ATP in Skeletal Muscle Contraction Hydrolysis of ATP by the Na+K+-ATPase in the plasma membrane maintains Na+ and K+ gradients, which allows the membrane to produce and propagate action potentials (review Figure 6.13). Hydrolysis of ATP by the Ca2+-ATPase in the sarcoplasmic reticulum provides the energy for the active transport of calcium ions into the reticulum, lowering cytosolic Ca2+ to prerelease concentrations, ending the contraction, and allowing the muscle fiber to relax. Hydrolysis of ATP by myosin-ATPase energizes the cross-bridges, providing the energy for force generation. Binding of ATP to myosin dissociates cross-bridges bound to actin, allowing the bridges to repeat their cycle of activity. 87 Table 9.2 Sequence of Events Between a Motor Neuron Action Potential and Skeletal Muscle Fiber Contraction 1 1. Action potential is initiated and propagates to motor neuron axon terminals. enters axon terminals through voltage-gated Ca2+ channels. entry triggers release of ACh from axon terminals. 4. ACh diffuses from axon terminals to motor end plate in muscle fiber. 5. ACh binds to nicotinic receptors on motor end plate, increasing their permeability to Na+ and K+. 6. More Na+ moves into the fiber at the motor end plate than K+ moves out, depolarizing the membrane and producing the end-plate potential (EPP). 7. Local currents depolarize the adjacent muscle cell plasma membrane to its threshold potential, generating an action potential that propagates over the muscle fiber surface and into the fiber along the T-tubules. 8. Action potential in T-tubules induces DHP receptors to pull open ryanodine receptor channels, allowing release of Ca2+ from terminal cisternae of sarcoplasmic reticulum. 9. Ca2+ binds to troponin on the thin filaments, causing tropomyosin to move away from its blocking position, thereby uncovering cross-bridge binding sites on actin. 88 Table 9.2 Sequence of Events Between a Motor Neuron Action Potential and Skeletal Muscle Fiber Contraction 2 10. Energized myosin cross-bridges on the thick filaments bind to actin: A + M ∙ ADP ∙ Pi → A ∙ M ∙ ADP ∙ Pi 11. Cross-bridge binding triggers release of ATP hydrolysis products from myosin, producing an angular movement of each cross-bridge: A ∙ M ∙ ADP ∙ Pi → A ∙ M + ADP + Pi 12. ATP binds to myosin, breaking linkage between actin and myosin and thereby allowing cross- bridges to dissociate from actin: A ∙ M + ATP ∙→ A + M ∙ ATP 13. ATP bound to myosin is split, energizing the myosin cross-bridge: M ∙ ATP → M ∙ ADP ∙ Pi 14. Cross-bridges repeat steps 10 to 13, producing movement (sliding) of thin filaments past thick filaments. Cycles of cross-bridge movement continue as long as Ca2+ remains bound to troponin. 15. Cytosolic Ca2+ concentration decreases as Ca2+ -ATPase actively transports Ca2+ into sarcoplasmic reticulum. 16. Removal of Ca2+ from troponin restores blocking action of tropomyosin, the cross-bridge cycle ceases, and the muscle fiber relaxes. 89 Mechanics of Single-Fiber Contraction The force exerted on an object by a contracting muscle is known as muscle tension, and the force exerted on the muscle by an object (usually its weight) is the load. Muscle tension and load are opposing forces. The mechanical response of a muscle fiber to a single action potential is known as a twitch. 90 Main Features of an Isotonic Twitch There are 3 main features of an isotonic twitch: – Latent Period This is the period of time from the action potential to the onset of contraction. The time delay is due to processes associated with excitation-contraction coupling. – Contraction Time This is the time interval from the beginning of tension development at the end of the latent period to the peak tension. – Relaxation Time This is the time interval from peak tension until the tension declines back to zero. 91 Isometric and Isotonic Contractions When a muscle develops tension but does not shorten or lengthen, the contraction is said to be an isometric (constant length) contraction. Such contractions occur when the muscle supports a load in a constant position or attempts to move an otherwise supported load that is greater than the tension developed by the muscle. A contraction in which the muscle changes length while the load on the muscle remains constant is an isotonic (constant tension) contraction. Two types of isotonic contractions: When tension exceeds load, shortening occurs, and the contraction is called a concentric contraction. When an unsupported load is greater than the tension generated by cross-bridges, the load pulls the muscle to a longer length in spite of cross-bridges; this is an eccentric contraction. 92 Frequency-Tension Relation Because a single action potential in a skeletal muscle fiber lasts only 1 to 2 milliseconds but the twitch may last for 100 milliseconds or more, it is possible for a second action potential to be initiated during the period of mechanical activity. When a stimulus is applied before a fiber has completely relaxed from a twitch, it induces a contractile response with a peak tension greater than that produced in a single twitch. The increase in muscle tension from successive action potentials occurring during the phase of mechanical activity is known as summation. A maintained contraction in response to repetitive stimulation is known as a tetanus (tetanic contraction). 93 Length-Tension Relation The spring-like characteristic of the protein titin is responsible for most of the passive elastic properties of relaxed muscle fibers. With increased stretch, the passive tension in a relaxed fiber increases, not from active cross-bridge movements but from elongation of the titin filaments. If the stretched fiber is released, it will return to an equilibrium length. By a different mechanism, the amount of active tension a muscle fiber develops during contraction can also be altered by changing the length of the fiber. If you stretch a muscle fiber to various lengths and tetanically stimulate it at each length, the magnitude of the active tension will vary with length. The length at which the fiber develops the greatest isometric active tension is termed the optimal length, (L0). 94 Skeletal Muscle Energy Metabolism ATP performs four functions directly related to muscle fiber contraction and relaxation. There are three ways a muscle fiber can form ATP: Phosphorylation of ADP by creatine phosphate Oxidative phosphorylation of ADP in the mitochondria Phosphorylation of ADP by the glycolytic pathway in the cytosol 95 Muscle Fatigue When a skeletal muscle fiber is repeatedly stimulated, the tension the fiber develops eventually decreases even though the stimulation continues. This decline in muscle tension as a result of previous contractile activity is known as muscle fatigue. Fatigued muscles also exhibit a decreased shortening velocity and a slower rate of relaxation. The onset of fatigue and its rate of development depend on the type of skeletal muscle fiber that is active, the intensity and duration of contractile activity, and the degree of an individual’s fitness. 96 Causes of Muscle Fatigue 1 Many factors can contribute to skeletal muscle fatigue. Acute fatigue from high-intensity, short-duration exercise is thought to involve: – decrease in ATP concentration + – increase in concentrations of ADP,P,i Mg ,H , 2+ and oxygen free radicals These metabolic changes have been shown to: – decrease the rate of Ca2+ release, reuptake, and storage by the endoplasmic reticulum – decrease the sensitivity of the thin filament proteins to activation by Ca2+ directly inhibit the binding and power-stroke motion of the myosin cross-bridges Each of these has been shown to be important under experimental conditions, but muscle fatigue is still not well understood. 97 Causes of Muscle Fatigue 2 Central Command Fatigue: – Another type of fatigue quite different from muscle fatigue occurs when the appropriate regions of the cerebral cortex fail to send excitatory signals to the motor neurons. – This may cause a person to stop exercising even though the muscles are not fatigued. – An athlete’s performance depends not only on the physical state of the appropriate muscles but also upon the mental ability to initiate central commands to muscles during a period of increasingly distressful sensations. 98 Types of Skeletal Muscle Fibers 1 Skeletal muscle fibers do not all have the same mechanical and metabolic characteristics. Fibers are classified on the basis of: – their maximal velocities of shortening (fast or slow-twitch) – the major pathway they use to form ATP (oxidative or glycolytic) Fast and slow-twitch fibers contain forms of myosin that differ in the maximal rates at which they use ATP, and corresponding differences in proteins that affect the speed of membrane excitation, excitation- contraction coupling, and ATP-production mechanisms. The myosin subtype in each fiber determines the maximal rate of cross-bridge cycling and thus the maximal shortening velocity. 99 Types of Skeletal Muscle Fibers 2 The second means of classifying skeletal muscle fibers is according to the abundance of the different types of enzymatic machinery available for synthesizing ATP. Some fibers contain numerous mitochondria and thus have a high capacity for oxidative phosphorylation. These fibers are classified as oxidative fibers. Most of the ATP such fibers produce is dependent upon blood flow to deliver oxygen and fuel molecules to the muscle. They also contain myoglobin, making these fibers appear darker, so they are sometimes called red muscle fibers. Muscles containing many of these fibers are recruited for long-term contractions (like maintaining posture). In contrast, glycolytic fibers have few mitochondria but possess a high concentration of glycolytic enzymes and a large store of glycogen. This allows for quick bursts of activity when these fibers are recruited. These fibers lack myoglobin and are thus referred to as white muscle fibers. 100 Types of Skeletal Muscle Fibers On the basis of these two characteristics, three main types of skeletal muscle fibers can be distinguished: – Slow-oxidative fibers (type 1) combine low myosin- ATPase activity with high oxidative capacity. – Fast-oxidative-glycolytic fibers (type 2A) combine high myosin-ATPase activity with high oxidative capacity and intermediate glycolytic capacity. – Fast-glycolytic fibers (type 2X) combine high myosin-ATPase activity with high glycolytic capacity. 101 Whole-Muscle Contraction Depending on the proportions of the fiber types present, muscles can differ considerably in their maximal contraction speed, strength, and fatigability. For example, the muscles of the back, which must maintain activity for long periods of time without fatigue while supporting an upright posture, contain large numbers of slow-oxidative fibers. In contrast, muscles in the arms that are used to produce large amounts of tension over a short time period, as when a boxer throws a punch, have a greater proportion of fast-glycolytic fibers. Leg muscles used for fast running over intermediate distances typically have a high proportion of fast-oxidative-glycolytic fibers. 102 Control of Muscle Tension The total tension a muscle can develop depends upon two factors: – the amount of tension developed by each fiber – the number of fibers contracting at any time By controlling these two factors, the nervous system controls whole-muscle tension as well as shortening velocity. 103 Table 9.4 Factors Determining Muscle Tension Tension Developed by Each Fiber Action potential frequency (frequency–tension relation) Fiber length (length–tension relation) Fiber diameter Fiber type Fatigue Number of Active Fibers Number of fibers per motor unit Number of active motor units 104 Motor Unit Size Varies from Muscle to Muscle The muscles in the hand and eye, which produce delicate movements, contain small motor units. For example, one motor neuron innervates only about 13 fibers in an eye muscle. The more coarsely controlled muscles, for example, in the legs, contain large motor units, containing hundreds or thousands of fibers. When a muscle is composed of small motor units, the total tension the muscle produces can be increased in small steps by activating additional motor units. This is called recruitment. If the motor units are large, large increases in tension will occur as each additional motor unit is activated. Thus, finer control of muscle tension is possible in muscles with small motor units. 105 Control of Shortening Velocity Shortening velocity of a whole muscle depends on: The load on the muscle The types of motor units in the muscle The number of motor units recruited to work against the load 106 Muscle Adaptation to Exercise Increased amounts of contractile activity, such as regular exercise, can produce an increase in the size, hypertrophy, of muscle fibers as well as changes in their capacity for ATP production and the subtype of myosin they express. “Use it or lose it.” Muscles that are not used will atrophy. There are 2 types of atrophy: – Disuse atrophy (like an arm in a cast) – Denervation atrophy (nerve damage = loss of function) 107 Skeletal Muscle Disorders A number of conditions and diseases can affect the contraction of skeletal muscle. Many of them are caused by defects in the parts of the nervous system that control contraction of the muscle fibers rather than by defects in the muscle fibers. For example, poliomyelitis is a viral disease that can destroy motor neurons, leading to the paralysis of skeletal muscle, which may result in death due to respiratory failure. 108 Muscle Cramps Involuntary tetanic contraction of skeletal muscles produces muscle cramps. During cramping, action potentials fire at abnormally high rates, a much greater rate than occurs during maximal voluntary contraction. The specific cause is uncertain, but it may be partly related to electrolyte imbalances in the extracellular fluid surrounding both the muscle and nerve fibers. Imbalances may arise from overexercise or persistent dehydration, and they can directly induce action potentials in motor neurons and muscle fibers. Another possibility is that chemical imbalances within the muscle stimulate sensory receptors in the muscle, and the motor neurons to the area are activated by reflex when those signals reach the spinal cord. 109 Hypocalcemic Tetany Hypocalcemic tetany is the involuntary tetanic contraction of skeletal muscles that occurs when the extracellular Ca2+ concentration falls to about 40% of its normal value. This may seem surprising, because we have seen that Ca2+ is required for excitation-contraction coupling. However, recall that this Ca2+ is sarcoplasmic reticulum Ca2+ , not Ca2+. extracellular effect of changes in extracellular The Ca2+ is exerted not on the sarcoplasmic reticulum Ca2+ , but directly on the plasma membrane. Low extracellular Ca2+ (hypocalcemia) increases the opening of Na+ channels in excitable membranes, leading to membrane depolarization and the spontaneous firing of action potentials. 110 Muscular Dystrophy Muscular dystrophy is a relatively common genetic disease, affecting an estimated one in every 3,500 males (but many fewer females). It is associated with the progressive degeneration of skeletal and cardiac muscle fibers, weakening the muscles, and ultimately death due to respiratory or cardiac failure. Muscular dystrophy is caused by the absence or defect of one or more proteins that make up the costameres in striated muscle. Costameres are clusters of structural and regulatory proteins that link the Z disks of the outermost myofibrils to the sarcolemma and extracellular matrix. Duchenne muscular dystrophy is a sex-linked recessive disorder caused by a defect in a gene on the X chromosome that codes for the protein, dystrophin. Dystrophin was the first costamere protein discovered to be related to a muscular dystrophy, which is how it earned its name. 111 Myasthenia Gravis Myasthenia gravis is a neuromuscular disorder characterized by muscle fatigue and weakness that progressively gets worse as the muscle is used. It affects about one 7,500 Americans, occurring more often in women than men. The most common cause is the destruction of nicotinic Ach-receptor proteins of the motor end plate, mediated by antibodies of a person’s own immune system. A number of approaches are currently used to treat the disease. One is to administer acetylcholinesterase inhibitors. This can partially compensate for the reduction in available ACh receptors by prolonging the time that acetylcholine is available at the synapse. Other therapies aim at blunting the immune response. Treatment with glucocorticoids is one way that immune function is suppressed. Plasmapheresis is a treatment that involves replacing the liquid fraction of blood (plasma) that contains the offending antibodies. 112