Chapter 8 Membrane Trafficking PDF
Document Details
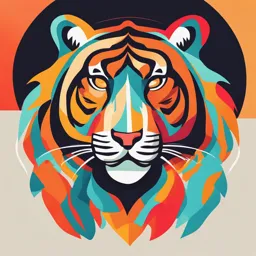
Uploaded by DazzlingLarch
Tags
Summary
This document is about membrane trafficking, focusing on the structure and function of the endomembrane system in cells. It details the different organelles involved in this process: endoplasmic reticulum, Golgi complex, endosomes, lysosomes, and vacuoles. It describes the secretory pathway and how materials are shuttled between organelles.
Full Transcript
270 8 Cytoplasmic Membrane Systems: Structure, Function, and Membrane Trafficking 8.1 An Overview of the Endomembrane System Under the light microscope, the cytoplasm of living cells 8.2 A Few Approaches to the Study of a...
270 8 Cytoplasmic Membrane Systems: Structure, Function, and Membrane Trafficking 8.1 An Overview of the Endomembrane System Under the light microscope, the cytoplasm of living cells 8.2 A Few Approaches to the Study of appears relatively devoid of structure. Yet, even before the begin- Endomembranes ning of the twentieth century, examination of stained sections of animal tissues hinted at the existence of an extensive membrane 8.3 The Endoplasmic Reticulum network within the cytoplasm. It wasn’t until the development of 8.4 The Golgi Complex the electron microscope in the 1940s, however, that biologists 8.5 Types of Vesicle Transport and Their Functions began to appreciate the diverse array of membrane-bound struc- 8.6 Lysosomes tures present in the cytoplasm of most eukaryotic cells. These early electron microscopists saw membrane-bound vesicles of 8.7 Plant Cell Vacuoles varying diameter containing material of different electron density; 8.8 The Endocytic Pathway: Moving Membrane long channels bounded by membranes that radiate through the and Materials into the Cell Interior cytoplasm to form an interconnected network of canals; and 8.9 Posttranslational Uptake of Proteins by stacks of flattened membrane-bound sacs, called cisternae. Peroxisomes, Mitochondria, and Chloroplasts It became evident from these early electron microscopic studies and the biochemical investigations that followed that the THE HUMAN PERSPECTIVE: Disorders Resulting from cytoplasm of eukaryotic cells was subdivided into a variety of Defects in Lysosomal Function distinct compartments bounded by membrane barriers. As more EXPERIMENTAL PATHWAYS: Receptor-Mediated types of cells were examined, it became apparent that these Endocytosis membranous compartments in the cytoplasm formed different organelles that could be identified in diverse cells from yeast to multicellular plants and animals. The extent to which the Colorized scanning electron micrograph of a human neutrophil, a type of white blood cell, ingesting a number of bacteria by the process of phagocytosis. Neutrophils are essential components of our innate immune response against pathogens. (FROM SCOTT D. KOBAYASHI ET AL., COVER OF PNAS, VOL. 100, #19, 2003, © 2003, NATIONAL ACADEMY OF SCIENCES, U.S.A., COURTESY OF FRANK R. DELEO.) 271 cytoplasm of a eukaryotic cell is occupied by membranous struc- tures is illustrated in the electron micrograph of a maize root cell shown in Figure 8.1. As we will see in the following pages, each of these organelles contains a particular complement of proteins and is specialized for particular types of activities. Thus, just as a house or restaurant is divided into specialized rooms where dif- ferent activities can take place independent of one another, the cytoplasm of a cell is divided into specialized membranous com- partments for analogous reasons. Keep in mind, as you examine the micrographs in this chapter, that these cytoplasmic organelles may appear as stable structures, like the rooms of a house or Golgi restaurant, but in fact they are dynamic compartments that are in complex continual flux. In the present chapter, we will examine the structure and ER functions of the endoplasmic reticulum, Golgi complex, Secretory endosomes, lysosomes, and vacuoles. Taken together, these vesicle organelles form an endomembrane system in which the individual components function as part of a coordinated unit. (Mitochondria and chloroplasts are not part of this interconnected Figure 8.1 Membrane-bound compartments of the cytoplasm. The system and were the subjects of Chapters 5 and 6. Current cytoplasm of this root cap cell of a maize plant contains an array of evidence suggests that peroxisomes, which were also discussed membrane-bound organelles whose structure and function will be in Chapter 6, have a dual origin. The basic elements of the examined in this chapter. As is evident in this micrograph, the boundary membrane are thought to arise from the endoplasmic combined surface area of the cytoplasmic membranes is many times reticulum, but many of the membrane proteins and the soluble greater than that of the surrounding plasma membrane. (COURTESY OF HILTON H. MOLLENHAUER.) internal proteins are taken up from the cytosol, as described in Section 8.9.) 8.1 | An Overview which proteins are synthesized in the endoplasmic reticulum, modified during passage through the Golgi complex, and of the Endomembrane System transported from the Golgi complex to various destinations, The organelles of the endomembrane system are part of a dy- such as the plasma membrane, a lysosome, or the large vacuole namic, integrated network in which materials are shuttled of a plant cell. This route is also referred to as the secretory back and forth from one part of the cell to another. For the pathway, as many of the proteins synthesized in the endoplas- most part, materials are shuttled between organelles—from mic reticulum (as well as complex polysaccharides synthesized the Golgi complex to the plasma membrane, for example—in in the Golgi complex, Figure 7.37c) are destined to be dis- small, membrane-bounded transport vesicles that bud from a charged (secreted) from the cell. Secretory activities of cells donor membrane compartment (Figure 8.2a).1 Transport can be divided into two types: constitutive and regulated vesicles move through the cytoplasm in a directed manner, of- (Figure 8.2b). During constitutive secretion, materials are 8.1 An Overview of the Endomembrane System ten pulled by motor proteins that operate on tracks formed by transported in secretory vesicles from their sites of synthesis microtubules and microfilaments of the cytoskeleton (see Fig- and discharged into the extracellular space in a continual ure 9.1a). When they reach their destination, the vesicles fuse manner. Most cells engage in constitutive secretion, a process with the membrane of the acceptor compartment, which re- that contributes not only to the formation of the extracellular ceives the vesicle’s soluble cargo as well as its membranous matrix (Section 7.1), but to the formation of the plasma mem- wrapper (Figure 8.2a). Repeated cycles of budding and fusion brane itself. During regulated secretion, materials are stored shuttle a diverse array of materials along numerous pathways as membrane-bound packages and discharged only in re- that traverse the cell. sponse to an appropriate stimulus. Regulated secretion occurs, Several distinct pathways through the cytoplasm have for example, in endocrine cells that release hormones, in pan- been identified and are illustrated in the overview shown in creatic acinar cells that release digestive enzymes, and in nerve Figure 8.2b. A biosynthetic pathway can be discerned in cells that release neurotransmitters. In some of these cells, materials to be secreted are stored in large, densely packed, 1 membrane-bound secretory granules (see Figure 8.3). The term vesicle implies a spherical-shaped carrier. Cargo may also be trans- ported in irregular or tubular-shaped membrane-bound carriers. For the sake of Proteins, lipids, and complex polysaccharides are trans- simplicity, we will generally refer to carriers as “vesicles,” while keeping in mind ported through the cell along the biosynthetic or secretory they are not always spherical. pathway. We will focus in the first part of the chapter on the 272 Figure 8.2 An overview of the biosynthetic/secretory and endocytic pathways that unite endomembranes into a dynamic, interconnected network. (a) Schematic diagram illustrating the process of vesicle transport by which materials are transported from a donor compartment to a recipient compartment. Vesicles form by membrane budding, dur- ing which specific membrane proteins (green spheres) of the donor membrane are incorporated into the vesicle membrane and specific sol- uble proteins (purple spheres) in the donor compartment are bound to specific receptors. When the transport vesicle subsequently fuses to an- Chapter 8 Cytoplasmic Membrane Systems: Structure, Function, and Membrane Trafficking other membrane, the proteins of the vesicle membrane become part of the recipient membrane, and the soluble proteins become sequestered within the lumen of the recipient compartment. (b) Materials follow the biosynthetic (or secretory) pathway from the endoplasmic reticulum, through the Golgi complex, and out to various locations including lysosomes, endosomes, secretory vesicles, secretory granules, vacuoles, and the plasma membrane. Materials follow the endocytic pathway from the cell surface to the interior by way of endosomes and lysosomes, where they are generally degraded by lysosomal enzymes. synthesis and transport of proteins, as summarized in Figure 8.2b. During the discussion, we will consider several distinct classes of proteins. These include soluble proteins that are dis- charged from the cell, integral proteins of the various mem- lysosomal enzymes, which are also manufactured in the endo- branes depicted in Figure 8.2b, and soluble proteins that plasmic reticulum, are specifically targeted to a lysosome. reside within the various compartments enclosed by the en- Different organelles also contain different integral membrane domembranes (e.g., lysosomal enzymes). Whereas materials proteins. Consequently, membrane proteins must also be move out of the cell by the secretory pathway, the endocytic targeted to particular organelles, such as a lysosome or Golgi pathway operates in the opposite direction. By following the cisterna. These various types of cargo—secreted proteins, endocytic pathway, materials move from the outer surface of lysosomal enzymes, and membrane proteins—are routed to the cell to compartments, such as endosomes and lysosomes, their appropriate cellular destinations by virtue of specific located within the cytoplasm (Figure 8.2b). “addresses” or sorting signals that are encoded in the amino The movement of vesicles and their contents along the acid sequence of the proteins or in the attached oligosaccha- various pathways of a cell is analogous to the movement of rides. The sorting signals are recognized by specific receptors trucks carrying different types of cargo along the various that reside in the membranes or surface coats of budding vesi- highways of a city. Both types of transport require defined cles, ensuring that the protein is transported to the appropri- traffic patterns to ensure that materials are accurately delivered ate destination. For the most part, the machinery responsible to the appropriate sites. For example, protein trafficking for driving this complex distribution system consists of solu- within a salivary gland cell requires that the proteins of sali- ble proteins that are recruited to specific membrane surfaces. vary mucus, which are synthesized in the endoplasmic reticu- During the course of this chapter we will try to understand lum, are specifically targeted to secretory granules, while why one protein is recruited, for example, to the endoplasmic 273 reticulum whereas another protein might be recruited to a covered with a thin layer of photographic emulsion, which is particular region of the Golgi complex. exposed by radiation emanating from radioisotopes within the Great advances have been made over the past three tissue. Sites in the cells containing radioactivity are revealed decades in mapping the traffic patterns that exist in eukaryotic under the microscope by silver grains in the overlying emul- cells, identifying the specific addresses and receptors that gov- sion (Figure 8.3). ern the flow of traffic, and dissecting the machinery that en- To determine the sites where secretory proteins are syn- sures that materials are delivered to the appropriate sites in the thesized, Palade and Jamieson incubated slices of pancreatic cell. These subjects will be discussed in detail in the following tissue in a solution containing radioactive amino acids for a pages. Motor proteins and cytoskeletal elements, which play brief period of time. During this period, labeled amino acids key roles in the movements of transport vesicles and other en- were taken up by the living cells and incorporated into the di- domembranes, will be described in the following chapter. We gestive enzymes as they were being synthesized on ribosomes. will begin the study of endomembranes by discussing a few of The tissues were quickly fixed, and the locations of proteins the most important experimental approaches that have led to that had been synthesized during the brief incubation with la- our current understanding of the subject. beled amino acids were determined autoradiographically. Us- ing this approach, the endoplasmic reticulum was discovered REVIEW to be the site of synthesis of secretory proteins (Figure 8.3a). 1. Compare and contrast the biosynthetic pathway with To determine the intracellular path followed by secretory the endocytic pathway. proteins from their site of synthesis to their site of discharge, Palade and Jamieson carried out an additional experiment. 2. How are particular proteins targeted to particular sub- After incubating the tissue for a brief period in radioactive cellular compartments? amino acids, they washed the tissue free of excess isotope and transferred the tissue to a medium containing only unlabeled amino acids. An experiment of this type is called a “pulse- chase.” The pulse refers to the brief incubation with radioactiv- 8.2 | A Few Approaches ity during which labeled amino acids are incorporated into protein. The chase refers to the period when the tissue is ex- to the Study of Endomembranes posed to the unlabeled medium, a period during which addi- Early studies with the electron microscope provided biolo- tional proteins are synthesized using nonradioactive amino gists with a detailed portrait of the structure of cells but gave acids. The longer the chase, the farther the radioactive pro- them little insight into the functions of the components they teins manufactured during the pulse will have traveled from were observing. Determining the functions of cytoplasmic their site of synthesis within the cell. Using this approach, one organelles required the development of new techniques and can ideally follow the movements of newly synthesized mole- the execution of innovative experiments. The experimental cules by observing a wave of radioactive material moving approaches described in the following sections have proven through the cytoplasmic organelles of cells from one location particularly useful in providing the foundation of knowledge to the next until the process is complete. The results of these on which current research on cytoplasmic organelles is based. experiments—which first defined the biosynthetic (or secre- tory) pathway and tied a number of seemingly separate mem- branous compartments into an integrated functional unit— Insights Gained from Autoradiography 8.2 A Few Approaches to the Study of Endomembranes are summarized in Figure 8.3b–d. Among the many cells in the body, the acinar cells of the pan- creas have a particularly extensive endomembrane system. Insights Gained from the Use These cells function primarily in the synthesis and secretion of of the Green Fluorescent Protein digestive enzymes. After secretion from the pancreas, these enzymes are shipped through ducts to the small intestine, The autoradiographic experiments described in the previous where they degrade ingested food matter. Where within the section require investigators to examine thin sections of dif- pancreatic acinar cells are the secretory proteins synthesized, ferent cells that have been fixed at various times after intro- and how do they reach the surface of the cells where they are duction of a radioactive label. Techniques involving the use of discharged? These questions are inherently difficult to answer radioactive isotopes have largely been abandoned by modern because all of the steps in the process of secretion occur simul- cell biologists. An alternative technology allows researchers to taneously within the cell. To follow the steps of a single cycle follow the dynamic movements of specific proteins with their from start to finish, that is, from the synthesis of a secretory own eyes as they occur within a single living cell. This tech- protein to its discharge from the cell, James Jamieson and nology utilizes a gene isolated from a jellyfish that encodes a George Palade of Rockefeller University utilized the tech- small protein, called the green fluorescent protein (GFP), nique of autoradiography (Section 18.4). which emits a green fluorescent light. In this approach, DNA Autoradiography provides a means to visualize biochem- encoding GFP is fused to DNA encoding the protein to be ical processes by allowing an investigator to determine the studied, and the resulting chimeric (i.e., composite) DNA is location of radioactively labeled materials within a cell. In this introduced into cells that can be observed under the micro- technique, tissue sections containing radioactive isotopes are scope. Once inside a cell, the chimeric DNA expresses a 274 Silver grains Silver grains Rough Rough ER ER Secretory Secretory granule granule Figure 8.3 Autoradiography reveals the sites of synthesis and subse- quent transport of secretory proteins. (a) Electron micrograph of a section of a pancreatic acinar cell that had been incubated for 3 minutes in radioactive amino acids and then immediately fixed and prepared for autoradiography. The black silver grains that appear in the emulsion following development are localized over the endoplasmic reticulum. (b–d) Diagrams of a sequence of autoradiographs showing the movement of labeled secretory proteins (represented by the silver grains in red) through a pancreatic acinar cell. When the cell is pulse-labeled for 3 minutes and immediately fixed (as shown in a), radioactivity is localized in the endoplasmic reticulum (b). After a 3-minute pulse and 17-minute chase, radioactive label is concentrated in the Golgi complex and adjacent vesicles (c). After a 3-minute pulse and 117-minute chase, radioactivity is concentrated in the secretory granules and is beginning Chapter 8 Cytoplasmic Membrane Systems: Structure, Function, and Membrane Trafficking to be released into the pancreatic ducts (d ). (A: COURTESY OF JAMES D. JAMIESON AND GEORGE PALADE.) (a) Lumen Secretory granules Silver grains Golgi complex Rough endoplasmic reticulum Nucleus Mitochondria 3 min 20 min 120 min (b) (c) (d) chimeric protein consisting of GFP fused to the end of the with VSV, massive amounts of the VSVG protein are protein to be studied. In most cases, the presence of GFP produced in the endoplasmic reticulum (ER). The VSVG joined to the end of a protein has little or no effect on the molecules then traffic through the Golgi complex and are movement or function of that protein. transported to the plasma membrane of the infected cell Figure 8.4 shows a pair of micrographs depicting cells where they are incorporated into viral envelopes. As in a that contain a GFP fusion protein. In this case, the cells were radioactive pulse-chase experiment, the use of a virus allows infected with a strain of the vesicular stomatitis virus (VSV) investigators to follow a relatively synchronous wave of pro- in which one of the viral genes (VSVG) is fused to the GFP tein movement, in this case represented by a wave of green gene. Viruses are useful in these types of studies because they fluorescence that begins soon after infection. Synchrony can turn infected cells into factories for the production of viral be enhanced, as was done in the experiment depicted in Fig- proteins, which are carried like any other protein cargo ure 8.4, by use of a virus with a mutant VSVG protein that is through the biosynthetic pathway. When a cell is infected unable to leave the ER of infected cells that are grown at an 275 Viral genome contains temperature- VSV sensitive VSVG gene fused to GFP gene + (a) (b) Golgi complex 40º C 32º C 60' 10' Nucleus ER (c) Figure 8.4 The use of green fluorescent protein (GFP) live infected cell that was held at 40⬚C to allow the VSVG protein to reveals the movement of proteins within a living cell. accumulate in the ER and then incubated at 32⬚C for 10 minutes. (a) Fluorescence micrograph of a live cultured mammalian cell that had The fluorescent VSVG protein has moved on to the Golgi complex. been infected with the VSV virus at 40⬚C. This particular strain of the (c) Schematic drawing showing the retention of the mutant VSVG VSV virus contained a VSVG gene that (1) was fused to a gene encod- protein in the ER at 40⬚C and its synchronous movement to the Golgi ing the fluorescent protein GFP and (2) contained a temperature- complex within 10 minutes of incubation at the lower temperature. sensitive mutation that prevented the newly synthesized VSVG protein (A,B: FROM DANIEL S. CHAO ET AL., COURTESY OF RICHARD H. from leaving the ER when kept at 40⬚C. The green fluorescence in this SCHELLER, J. CELL BIOL. 144:873, 1999; REPRODUCED WITH micrograph is restricted to the ER. (b) Fluorescence micrograph of a PERMISSION OF THE ROCKEFELLER UNIVERSITY PRESS.) elevated temperature (e.g., 40⬚C). When the temperature is cytoplasmic membranes become fragmented and the fractured 8.2 A Few Approaches to the Study of Endomembranes lowered to 32⬚C, the fluorescent GFP-VSVG protein that edges of the membrane fragments fuse to form spherical vesi- had accumulated in the ER (Figure 8.4a,c) moves synchro- cles less than 100 nm in diameter. Vesicles derived from differ- nously to the Golgi complex (Figure 8.4b,c), where various ent organelles (nucleus, mitochondrion, plasma membrane, processing events occur, and then on to the plasma membrane. endoplasmic reticulum, and so forth) have different properties, Mutants of this type that function normally at a reduced which allow them to be separated from one another, an (permissive) temperature, but not at an elevated (restrictive) approach that is called subcellular fractionation. temperature, are described as temperature-sensitive mutants. Membranous vesicles derived from the endomembrane An experiment utilizing two different fluorescent probes is system (primarily the endoplasmic reticulum and Golgi com- described on page 321. plex) form a heterogeneous collection of similar sized vesicles referred to as microsomes. A rapid (and crude) preparation of the microsomal fraction of a cell is depicted in Figure 8.5a. The Insights Gained from the Biochemical microsomal fraction can be further fractionated into smooth Analysis of Subcellular Fractions and rough membrane fractions (Figure 8.5b,c) by the gradient Electron microscopy, autoradiography, and the use of GFP techniques discussed in Section 18.6. Once isolated, the bio- provide information on the structure and function of cellular chemical composition of various fractions can be determined. organelles but fail to provide much insight into the molecular In recent years, the identification of the proteins present in composition of these structures. Techniques to break up cell fractions has been carried out using sophisticated pro- (homogenize) cells and isolate particular types of organelles were teomic technology. Once a particular organelle has been iso- pioneered in the 1950s and 1960s by Albert Claude and Chris- lated, the proteins can be extracted, separated, and identified tian De Duve. When a cell is ruptured by homogenization, the by mass spectrometry as discussed on page 72. Hundreds of 276 Figure 8.5 Isolation of a microsomal fraction by differential centrifugation. (a) When a cell is broken by mechanical homogenization (step 1), the various membranous organelles become fragmented and form spherical membranous vesicles. Vesicles derived from different organelles can be separated by various techniques of centrifugation. In the procedure depicted here, the cell homogenate is first subjected to low-speed centrifugation to pellet Homogenate the larger particles, such as nuclei and mitochondria, leaving the smaller vesicles (microsomes) 1 in the supernatant (step 2). The microsomes can be removed from the supernatant by cen- Homogenize trifugation at higher speeds for longer periods of time (step 3). A crude microsomal fraction of this type can be fractionated into different vesicle types in subsequent steps. (b) Electron micrograph of a smooth microsomal fraction in which the membranous vesicles Whole cells lack ribosomes. (c) Electron micrograph of a rough microsomal fraction containing ribosome-studded membranes. (B,C: COURTESY OF J. A. HIGGINS AND R. J. BARNETT.) Postnuclear supernatant 2 proteins can be identified simultaneously, providing a compre- hensive molecular portrait of any organelle that can be Centrifuge prepared in a relatively pure state. In one example of this tech- 20,000g for 20 min. nology, it was found that a simple phagosome (page 315) con- taining an ingested latex bead comprised more than 160 different proteins, many of which had never previously been identified or were not known to be involved in phagocytosis. Homogenate Whole cells, nuclei, Insights Gained from the Use mitochondria of Cell-Free Systems Transfer postnuclear Chapter 8 Cytoplasmic Membrane Systems: Structure, Function, and Membrane Trafficking Once techniques to fractionate membranous organelles were supernatant developed, researchers began to probe the capabilities of these crude subcellular preparations. They found that the isolated parts of a cell were capable of remarkable activities. These 3 early cell-free systems—so called because they did not con- Centrifuge tain whole cells—provided a wealth of information about bio- 50,000g logical processes that were impossible to study within the for 2 hrs. complex environment of intact cells. During the 1960s, for example, George Palade, Philip Siekevitz, and their colleagues at Rockefeller University set out to learn more about the Postmicrosomal properties of the rough microsomal fraction (shown in Fig- supernatant ure 8.5c), whose membrane vesicles are derived from the rough ER (page 280). They found that they could strip a Microsomes rough microsomal preparation of its attached particles, and (a) the isolated particles (i.e., ribosomes) were capable of synthe- sizing proteins when provided with required ingredients from the cytosol. Under these conditions, the newly synthesized proteins were simply released by the ribosomes into the aque- ous fluid of the test tube. When the same experiment was car- ried out using intact rough microsomes, the newly synthesized proteins were no longer released into the incubation medium but were trapped within the lumen of the membranous vesi- cles. It was concluded from these studies that the microsomal (b) (c) membrane was not required for the incorporation of amino acids into proteins but for sequestering newly synthesized secretory proteins within the ER cisternal space. Over the past few decades, researchers have used cell-free with purified proteins that normally comprise coats on the cy- systems to identify the roles of many of the proteins involved tosolic surface of transport vesicles within the cell. Without in membrane trafficking. Figure 8.6 shows a liposome with the added coat proteins, vesicle budding could not occur. Us- vesicles budding from its surface (arrows). As discussed on ing this strategy in which cellular processes are reconstituted in page 128, liposomes are vesicles whose surface consists of an vitro from purified components, researchers have been able to artificial bilayer that is created in the laboratory from purified study the proteins that bind to the membrane to initiate vesi- phospholipids. The buds and vesicles seen in Figure 8.6 were cle formation, the proteins responsible for cargo selection, and produced after the preparation of liposomes was incubated the proteins that sever the vesicle from the donor membrane. 277 Insights Gained from the Study of Mutant Phenotypes A mutant is an organism (or cultured cell) whose chromo- somes contain one or more genes that encode abnormal pro- teins. When a protein encoded by a mutant gene is unable to carry out its normal function, the cell carrying the mutation exhibits a characteristic deficiency. Determining the precise nature of the deficiency provides information on the function of the normal protein. Study of the genetic basis of secretion has been carried out largely on yeast cells, most notably by Randy Schekman and colleagues at the University of California, Berkeley. Yeasts are particularly amenable to genetic studies because they have a small number of genes compared to other types of eukaryotes; they are small, single-celled organisms easily grown in culture; and they can be grown as haploids for the majority of their life cycle. A mutation in a single gene in a haploid cell produces an observable effect because the cells lack a second copy of the gene that would mask the presence Figure 8.6 Formation of coated vesicles in a cell-free system. of the abnormal one. Electron micrograph of a liposome preparation that had been In yeast, as in all eukaryotic cells, vesicles bud from the ER incubated with the components required to promote vesicle budding and travel to the Golgi complex, where they fuse with the Golgi within the cell. The proteins in the medium have become attached to cisternae (Figure 8.7a). To identify genes whose encoded pro- the surface of the liposomes and have induced the formation of tein is involved in this portion of the secretory pathway (i.e., protein-coated buds (arrows). (COURTESY OF LELIO ORCI AND SEC genes), researchers screen for mutant cells that exhibit an RANDY SCHEKMAN.) abnormal distribution of cytoplasmic membranes. An electron micrograph of a wild-type yeast cell is shown in Figure 8.7b. The cell depicted in Figure 8.7c has a mutation in a gene that Figure 8.7 The use of genetic mutants in the study of secretion. (a) The first leg of the biosynthetic secretory pathway in budding yeast. The steps are described below. (b) Electron micrograph of a section through a wild-type yeast cell. (c) A yeast cell bearing a mutation in the Vesicle sec12 gene whose product is involved in the formation of vesicles at the Vesicle targeting ER membrane (step 1, part a). Because vesicles cannot form, expanded budding and fusion ER cisternae accumulate in the cell. (d ) A yeast cell bearing a mutation 1 2 in the sec17 gene, whose product is involved in vesicle fusion (step 2, part a). Because they cannot fuse with Golgi membranes, the vesicles (indicated by the arrowheads) accumulate in the cell. [The mutants depicted in c and d are temperature-sensitive mutants. When kept at 8.2 A Few Approaches to the Study of Endomembranes the lower (permissive) temperature, they are capable of normal growth Rough and division.] (FROM CHRIS A. KAISER AND RANDY SCHEKMAN, endoplasmic Golgi CELL 61:724, 1990; © 1990, REPRINTED WITH PERMISSION FROM reticulum complex ELSEVIER.) (a) (b) 1 (c) (d) 278 encodes a protein involved in the formation of vesicles at the ER membrane (step 1, Figure 8.7a). In the absence of vesicle formation, mutant cells accumulate an expanded endoplasmic reticulum. In contrast, the cell depicted in Figure 8.7d carries a mutation in a gene that encodes a protein involved in vesicle fu- sion (step 2, Figure 8.7a). When this gene product is defective, the mutant cells accumulate an excess number of unfused vesi- cles. Researchers have isolated dozens of different mutants which, taken as a group, exhibit disruptions in virtually every step of the secretory pathway. The genes responsible for these defects have been cloned and sequenced, and the proteins they (a) (b) encode have been isolated. Isolation of proteins from yeast has launched successful searches for homologous proteins (i.e., pro- Figure 8.8 Inhibition of gene expression with RNA interference. teins with related sequence) in mammals. (a) A control Drosophila S2 cultured cell expressing GFP-labeled man- nosidase II. The fluorescent enzyme becomes localized in the Golgi One of the most important lessons learned from the use complex after its synthesis in the ER. (b) A cell that has been genetically of all of these techniques is that the dynamic activities of the engineered to express a specific siRNA, which binds to a complemen- endomembrane system are highly conserved. Not only do tary mRNA and inhibits translation of the encoded protein. In this yeast, plant, insect, and human cells carry out similar case, the siRNA has caused the fluorescent enzyme to remain in the processes, but they do so with remarkably similar proteins. It ER, which has fused with the Golgi membranes. This phenotype sug- is evident that the structural diversity of cells belies their un- gests that the mRNA being affected by the siRNA encodes a protein derlying molecular similarities. In many cases, proteins from involved in an early step of the secretory pathway during which the widely divergent species are interchangeable. For example, enzyme is synthesized in the ER and traffics to the Golgi complex. cell-free systems derived from mammalian cells can often uti- Among the genes that exhibit this phenotype when targeted by siRNAs lize yeast proteins to facilitate vesicle transport. Conversely, are those encoding proteins of the COPI coat, Sar1, and Sec23. The yeast cells with genetic deficiencies that disrupt some phase of functions of these proteins are discussed later in the chapter. (FROM Chapter 8 Cytoplasmic Membrane Systems: Structure, Function, and Membrane Trafficking FREDERIC BARD ET AL., COURTESY OF VIVEK MALHOTRA, NATURE the biosynthetic pathway can often be “cured” by genetically 439:604, 2006; © 2006, REPRINTED BY PERMISSION OF MACMILLAN engineering them to carry mammalian genes. PUBLISHERS LTD.) Over the past decade or so, researchers interested in searching for genes that affect a particular cellular process in plant or animal cells have taken advantage of a cellular phe- nomenon called RNA interference (RNAi). RNAi is a process enzyme from the ER to the Golgi complex. Of the 130 differ- in which cells produce small RNAs (called siRNAs) that ent siRNAs that were found to interfere in some way with the bind to specific mRNAs and inhibit the translation of these secretory pathway in this study, 31 of them generated a phe- mRNAs into proteins. This phenomenon and its use are dis- notype similar to that shown in Figure 8.8b. Included among cussed in detail in Sections 11.5 and 18.17. For the present these 31 siRNAs were numerous species that inhibited the ex- purpose we will simply note that researchers can synthesize a pression of genes that were already known to be involved in collection (library) of siRNAs that are capable of inhibiting the secretory pathway. In addition, the study identified other the translation of virtually any mRNA that is produced by a genes whose function had been unknown and are now pre- genome. Each mRNA represents the expression of a specific sumed to be involved in these processes as well. Because it is gene; therefore, one can find which genes are involved in a easier to synthesize a small RNA than to generate an organ- particular process by determining which siRNAs interfere ism with a mutant gene, RNAi has become a common strat- with that process. In the experiment depicted in Figure 8.8, egy to investigate the effect of a missing protein. researchers set out to identify genes that were involved in var- ious steps of the secretory pathway, a goal similar to that of the REVIEW investigators studying the yeast mutants shown in Figure 8.7. In this case, researchers used a strain of cultured Drosophila 1. Describe the differences between an autoradiograph cells and attempted to identify genes that affected the local- of a pancreas cell that had been incubated in labeled ization of mannosidase II, an enzyme that is synthesized in amino acids for 3 minutes and immediately fixed and the endoplasmic reticulum and moves via transport vesicles to one of a cell that had been labeled for 3 minutes, the Golgi complex, where it takes up residence. Figure 8.8a chased for 40 minutes, and then fixed. shows a control cell that is synthesizing a GFP-labeled ver- 2. What techniques or approaches might you use to learn sion of mannosidase II; the fluorescence becomes localized in which proteins are normally present in the endoplasmic the numerous Golgi complexes of the cell as would be ex- reticulum? pected. Figure 8.8b shows a cell that contains siRNA mole- 3. How does the isolation of a mutant yeast that accumu- cules that have caused a relocation of the GFP-mannosidase lates vesicles provide information on the process of into the ER, which is seen to be fused with the Golgi com- protein trafficking? plexes. This type of phenotype is typically caused by the ab- 4. How can GFP be used to study membrane dynamics? sence of one of the proteins involved in the transport of the 279 8.3 | The Endoplasmic Reticulum surface, whereas the smooth ER lacks associated ribosomes. The RER is typically composed of a network of flattened sacs The endoplasmic reticulum (ER) comprises a network of (cisternae), as shown in Figure 8.9a. The RER is continuous membranes that penetrates much of the cytoplasm. The ER with the outer membrane of the nuclear envelope, which also probably evolved from invaginations of the plasma membrane bears ribosomes on its cytosolic surface (Figure 8.2b). In con- as depicted in Figure 1, page 27. Enclosed within the ER is an trast, the membranes of the SER are highly curved and tubu- extensive space, or lumen, that is separated from the sur- lar, forming an interconnecting system of pipelines traversing rounding cytosol by the ER membrane. As will be evident in the cytoplasm. When cells are homogenized, the SER tubules the following discussion, the composition of the luminal (or fragment into smooth-surfaced vesicles, whereas the RER cisternal) space inside the ER membranes is quite different sheets fragment into rough-surfaced vesicles (Figure 8.5b,c). from that of the surrounding cytosolic space. Like other sub- Fluorescently labeled proteins and lipids are capable of cellular organelles, the ER is a highly dynamic structure diffusing from one type of ER into the other, indicating that undergoing continual turnover and reorganization. their membranes are continuous. In fact, the two types of ER The endoplasmic reticulum is divided into two subcom- share many of the same proteins and engage in certain com- partments, the rough endoplasmic reticulum (RER) and the mon activities, such as the synthesis of certain lipids and cho- smooth endoplasmic reticulum (SER). The rough ER is lesterol. At the same time, numerous proteins are found only defined by the presence of ribosomes bound to its cytosolic in one or the other type of ER. For example, the high degree Cytosol Ribosomes Cisternal space Cytosolic space Cisternae of rough endoplasmic reticulum (a) (b) ER ER ERER Nucleus Nucleus Nucleus Nucleus 8.3 The Endoplasmic Reticulum (c) (d) Figure 8.9 The rough endoplasmic reticulum (RER). (a) Schematic pancreatic acinar cell. (d ) Visualization of the rough ER in a whole diagram showing the stacks of flattened cisternae that make up the cultured cell as revealed by immunofluorescence staining for the rough ER. The cytosolic surface of the ER membrane contains bound enzyme protein disulfide isomerase (PDI), an ER resident protein. ribosomes, which gives the cisternae their rough appearance. (B: MEDIMAGE/PHOTO RESEARCHERS, INC.; C: FROM K. TANAKA, (b) Colorized transmission electron micrograph of a portion of the INT. REV. CYTOL. 68:101, COURTESY OF K. TANAKA; 1980; D: FROM rough ER of a pancreatic acinar cell. The division of the rough ER into BRIAN STORRIE, RAINER PEPPERKOK, AND TOMMY NILSSON, a cisternal space (which is devoid of ribosomes) and a cytosolic space is TRENDS CELL BIOL. 10:388, © 2000, WITH PERMISSION FROM evident. (c) Scanning electron micrograph of the rough ER in a ELSEVIER SCIENCE.) 280 of curvature of the SER tubules is induced and maintained by Sequestering calcium ions within the cytoplasm of cells. the presence of membrane-bending proteins, called reticulons, The regulated release of Ca2⫹ from the SER of skeletal which are largely absent from the flattened RER sheets. The and cardiac muscle cells (known as the sarcoplasmic reticu- RER, in contrast, contains proteins involved in the movement lum in muscle cells) triggers contraction. of nascent proteins into the ER lumen. Different types of cells contain markedly different ratios of the two types of ER, depending on the activities of the cell. Functions of the Rough Endoplasmic Reticulum For example, cells that secrete large amounts of proteins, such Early investigations into the functions of the RER were car- as the cells of the pancreas or salivary glands, have extensive ried out on cells that secrete large quantities of proteins, such regions of RER (Figure 8.9b–d). We will return to the func- as the acinar cells of the pancreas (Figure 8.3) or the mucus- tion of the RER shortly, but first we will describe the activities secreting cells of the lining of the digestive tract (Figure 8.11). of the SER. It is evident from the drawing and micrograph of Figure 8.11 that the organelles of these epithelial secretory cells are posi- The Smooth Endoplasmic Reticulum tioned in the cell in such a way as to produce a distinct polar- ity from one end to the other. The nucleus and an extensive The SER is extensively developed in a number of cell types, array of RER cisternae are located near the basal surface of the including those of skeletal muscle, kidney tubules, and cell, which faces the blood supply. The Golgi complex is lo- steroid-producing endocrine glands (Figure 8.10). SER func- cated in the central region of the cell. The apical surface of the tions include: cell faces a duct that carries the secreted proteins out of the or- Synthesis of steroid hormones in the endocrine cells of the gan. The cytoplasm at the apical end of the cell is filled with gonad and adrenal cortex. secretory granules whose contents are ready to be released into the duct upon arrival of the appropriate signal. The polarity of Detoxification in the liver of a wide variety of organic these glandular epithelial cells reflects the movement of secre- compounds, including barbiturates and ethanol, whose tory proteins through the cell from their site of synthesis to chronic use can lead to proliferation of the SER in liver their site of discharge. The rough ER is the starting point of Chapter 8 Cytoplasmic Membrane Systems: Structure, Function, and Membrane Trafficking cells. Detoxification is carried out by a collection of oxygen- the biosynthetic pathway: it is the site of synthesis of the pro- transferring enzymes (oxygenases), including the cytochrome teins, carbohydrate chains, and phospholipids that journey P450 family. These enzymes are noteworthy for their lack through the membranous compartments of the cell. of substrate specificity, being able to oxidize thousands of different hydrophobic compounds and convert them into Synthesis of Proteins on Membrane-Bound versus Free more hydrophilic, more readily excreted derivatives. The Ribosomes The discovery of the rough endoplasmic retic- results are not always positive. For example, the relatively ulum as the site of synthesis of secretory proteins in pancreatic harmless compound benzo[a]pyrene formed when meat is acinar cells was described earlier (Figure 8.3). Similar results charred on a grill is converted into a potent carcinogen by were found for other types of secretory cells, including intes- the “detoxifying” enzymes of the SER. Cytochrome P450s tinal goblet cells that secrete mucoproteins, endocrine cells that metabolize many prescribed medications, and genetic vari- secrete polypeptide hormones, plasma cells that secrete anti- ation in these enzymes among humans may explain differ- bodies, and liver cells that secrete blood serum proteins. ences from one person to the next in the effectiveness and Further experiments revealed that polypeptides are syn- side effects of many drugs. thesized at two distinct locales within the cell. 1. Approximately one-third of the proteins encoded by a mammalian genome are synthesized on ribosomes at- tached to the cytosolic surface of the RER membranes. These include (a) secreted proteins, (b) integral mem- brane proteins, and (c) soluble proteins that reside within compartments of the endomembrane system, including the ER, Golgi complex, lysosomes, endosomes, vesicles, and plant vacuoles. 2. Other polypeptides are synthesized on “free” ribosomes, that is, on ribosomes that are not attached to the RER, and are subsequently released into the cytosol. This class includes (a) proteins destined to remain in the cytosol (such as the enzymes of glycolysis and the proteins of the cytoskeleton), (b) peripheral proteins of the cytosolic sur- Figure 8.10 The smooth ER (SER). Electron micrograph of a face of membranes (such as spectrins and ankyrins that Leydig cell from the testis showing the extensive smooth ER where are only weakly associated with the plasma membrane’s steroid hormones are synthesized. DON FAWCETT/PHOTO RESEARCHERS, INC. cytosolic surface), (c) proteins that are transported to the nucleus (Section 12.2), and (d) proteins to be incorporated 281 Duct Secretory granules Mucigen granule Golgi complex Lysosome Rough ER Golgi complex (b) Nucleus Figure 8.11 Polarized structure of a secretory cell. (a) Drawing of a mucus-secreting goblet cell from the rat colon. (b) Low-power electron micrograph of a mucus- Mitochondrion secreting cell from Brunner’s gland of the mouse small intestine. Both types of cells display a distinctly polarized arrangement of organelles, reflecting their role in secreting large quantities of mucoproteins. The basal ends of the cells contain the nucleus and rough ER. Proteins synthesized in the rough ER move into the closely associated Golgi complex and from there into membrane-bound carriers in which the final secretory product is concentrated. The apical regions of the cells are filled RER with secretory granules containing the mucoproteins ready for release into a duct. (A: FROM MARIAN NEUTRA AND C. P. LEBLOND, COPYRIGHT 1966, ROCKEFELLER UNIVERSITY PRESS. ORIGINALLY PUBLISHED IN THE JOURNAL OF CELL BIOLOGY VOLUME 30:119. B: FROM ALAIN RAMBOURG AND YVES CLERMONT, EUR. J. CELL (a) BIOL. 51:196, 1990; © 1990, REPRINTED WITH PERMISSION OF ELSEVIER.) into peroxisomes, chloroplasts, and mitochondria. Proteins 2. The polypeptide moves into the cisternal space of the ER in the latter two groups are synthesized to completion in through a protein-lined, aqueous channel in the ER the cytosol and then imported posttranslationally into the membrane. It was proposed that the polypeptide moves appropriate organelle across its boundary membrane(s) through the membrane as it is being synthesized, that is, (page 316). cotranslationally.2 What determines the location in a cell where a protein is 2 It should be noted that protein transport across the ER membrane can also synthesized? In the early 1970s, Günter Blobel, in collabora- occur posttranslationally (i.e., after synthesis). In this process, the polypeptide is 8.3 The Endoplasmic Reticulum tion with David Sabatini and Bernhard Dobberstein of Rock- synthesized totally in the cytosol and then imported into the ER lumen through efeller University, first proposed, and then demonstrated, that the same protein-conducting channels used in the cotranslational pathway. The posttranslational pathway is utilized much more heavily in yeast than in mam- the site of synthesis of a protein was determined by the se- malian cells for import into the ER. In fact, yeast cells that are unable to engage quence of amino acids in the N-terminal portion of the in cotranslational transport into the ER are still able to survive, even though polypeptide, which is the first part to emerge from the ribo- they grow more slowly than normal cells. It can also be noted that a substantial some during protein synthesis. They suggested the following: number of integral membrane proteins (including the SNAREs disscussed on page 302) have a single transmembrane domain located near the C-terminus of the polypeptide chain. Because of its position within the polypeptide, the trans- 1. Secretory proteins contain a signal sequence at their membrane domain is the last segment to leave the ribosome and cannot be rec- N-terminus that directs the emerging polypeptide and ognized by the SRP. These tail-anchored proteins must also be inserted into the ribosome to the ER membrane. membrane posttranslationally (see Nature Revs. Mol. Cell Biol. 12:787, 2011). 282 This proposal, known as the signal hypothesis, has been thesis of the polypeptide begins after a messenger RNA binds substantiated by a large body of experimental evidence. Even to a free ribosome, that is, one that is not attached to a cyto- more importantly, Blobel’s original concept that proteins con- plasmic membrane. In fact, all ribosomes are thought to be tain built-in “address codes” has been shown to apply in prin- identical; those employed in the synthesis of secretory, lysoso- ciple to virtually all types of protein trafficking pathways mal, or plant vacuolar proteins are taken from the same popu- throughout the cell. lation (pool ) as those used for production of proteins that remain in the cytosol. Polypeptides synthesized on mem- Synthesis of Secretory, Lysosomal, or Plant Vacuolar brane-bound ribosomes contain a signal sequence—which Proteins on Membrane-Bound Ribosomes The steps typically includes a stretch of 6–15 hydrophobic amino acid that take place during the synthesis of a secretory, lysosomal, residues—that targets the nascent polypeptide to the ER or plant vacuolar protein are shown in Figure 8.12a. The syn- membrane and leads to the compartmentalization of the Figure 8.12 A schematic model of the synthesis are accompanied by the reciprocal hydrolyis of GTP molecules (not of a secretory protein (or a lysosomal enzyme) on a shown) bound to both the SRP and its receptor. In the model membrane-bound ribosome of the RER. (a) Syn- depicted here, the signal peptide then binds to the interior of the thesis of the polypeptide begins on a free ribosome. translocon, displacing the plug from the channel and allowing the As the signal sequence (shown in red) emerges from the ribosome, it remainder of the polypeptide to translocate through the membrane