Chapter 7-Photosynthesis: Using Light to Make Food PDF
Document Details
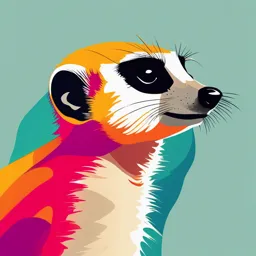
Uploaded by PeacefulBlueLaceAgate
Tags
Summary
This document provides a detailed overview of photosynthesis, discussing its fundamental principles, and describing the processes involved, including the light-dependent reactions and the Calvin cycle.
Full Transcript
**Chapter 7-Photosynthesis: Using Light to Make Food** **7.1 Photosynthesis powers most life on Earth** Life on Earth thrives on solar energy. Chloroplasts in plant cells capture sunlight, which travels 150 million kilometers from the sun. Through photosynthesis, plants convert carbon dioxide (CO~...
**Chapter 7-Photosynthesis: Using Light to Make Food** **7.1 Photosynthesis powers most life on Earth** Life on Earth thrives on solar energy. Chloroplasts in plant cells capture sunlight, which travels 150 million kilometers from the sun. Through photosynthesis, plants convert carbon dioxide (CO~2~) and water (H~2~O) into sugars and other organic molecules, releasing oxygen (O~2~) as a by-product. Plants, being autotrophs (self-feeders), produce their own food and serve as the primary source of organic molecules for nearly all other organisms. Specifically, plants and other photosynthesizers are known as photoautotrophs because they harness light energy. Photoautotrophs, often called the producers of the biosphere, create a food supply that other organisms rely on. They feed the consumers of the biosphere, known as heterotrophs, which cannot make their own food and must consume plants, animals, or decompose organic material. Heterotrophs, including humans, depend entirely on photoautotrophs for essential raw materials, organic fuel, and the oxygen needed for cellular respiration. Photoautotrophs provide us with food, clothing (like cotton), housing (like wood), and energy for warmth, light, transport, and manufacturing. Fossil fuels are ancient stores of the sun\'s energy captured by photoautotrophs long ago. The photographs highlight the diversity of today\'s photoautotrophs. On land, plants in tropical forests are the producers. In aquatic environments, photoautotrophs include algae, some protists, and photosynthetic prokaryotes. Examples include kelp, which forms underwater forests off California\'s coast, and cyanobacteria, important producers in freshwater and marine ecosystems. This chapter delves into photosynthesis in plants, occurring in chloroplasts. The ability of chloroplasts to harness light energy and synthesize organic compounds stems from the structural organization and interactions of their components. Photosynthetic pigments and enzymes are grouped in membranes and compartments, enabling the complex chemical reactions of photosynthesis. Some bacteria also perform photosynthesis, but they lack chloroplasts and use infolded plasma membrane regions containing photosynthetic molecules. What do "self-feeding" photoautotrophs require from the environment to make their own food? Light, CO~2~, and H~2~O. **7.2 Photosynthesis occurs in chloroplasts in plant cells** All green parts of a plant contain chloroplasts, but leaves are the primary sites of photosynthesis. A 1 mm² section of a leaf has about half a million chloroplasts. The green color of leaves comes from chlorophyll, a pigment in chloroplasts that converts solar energy into chemical energy. Figure 7.2 takes you on a journey inside a leaf, into a cell, and finally into a chloroplast, the site of photosynthesis. Chloroplasts are concentrated in the mesophyll cells, the green tissue inside the leaf. CO~2~ enters and O~2~ exits through tiny pores called stomata. Water absorbed by the roots is delivered to the leaves through veins, which also transport manufactured sugar to other parts of the plant. A single mesophyll cell contains numerous chloroplasts, typically around 30 to 40. The structures within a chloroplast are shown in the bottom drawing and electron micrograph. Chloroplast membranes form the framework for many photosynthesis reactions, like how mitochondrial membranes support energy-harvesting in cellular respiration. In the chloroplast, two membranes enclose an inner compartment filled with stroma, a thick fluid. Suspended in the stroma are interconnected membranous sacs called thylakoids, which enclose the thylakoid space. Thylakoids are often stacked in grana (singular: granum). The thylakoid membranes contain chlorophyll molecules that capture light energy and house the machinery to convert light energy to chemical energy, which is then used in the stroma to make sugar. The precise arrangement of membranes and compartments is crucial for photosynthesis, exemplifying the theme of structure and function. This chapter will explore these structures and their functions in detail, starting with an overview of the general process of photosynthesis. How do the reactant molecules of photosynthesis reach the chloroplasts in leaves? CO~2~ enters leaves through stomata, and H~2~O enters the roots and is carried to leaves through veins. **7.4 Photosynthesis is a redox process** Photosynthesis and cellular respiration are indeed complementary processes. Photosynthesis converts carbon dioxide and water into glucose and oxygen using sunlight, while cellular respiration breaks down glucose and oxygen to produce carbon dioxide, water, and energy (ATP). Both processes involve redox reactions, where electrons are transferred between molecules, and they illustrate the transformation of energy. In cellular respiration, glucose is oxidized to carbon dioxide, and oxygen is reduced to water, releasing energy that is used to synthesize ATP in the mitochondria. In photosynthesis, electrons move from water (H~2~O) to carbon dioxide (CO~2~). Water is split, and its electrons are transferred along with hydrogen ions (H+) to CO~2~, reducing it to sugar. The potential energy of electrons increases as they move from H~2~O to CO~2~, with light energy captured by chlorophyll molecules in the chloroplast providing this energy boost. This process involves energy-requiring redox reactions, where CO~2~ is reduced to sugar and water molecules are oxidized to O~2~. **7.5 Photosynthesis occurs in two stages, which are linked by ATP and NADPH** Photosynthesis is a complex process that can be summarized by a simple equation. It consists of two linked processes, each with multiple steps: the light reactions and the Calvin cycle. The light reactions capture energy from sunlight and convert it into chemical energy, while the Calvin cycle uses this energy to convert carbon dioxide into glucose. A diagram of a chloroplast shows the inputs and outputs of these two stages and how they are related. The light reactions occur on and inside the thylakoids, converting light energy to chemical energy and releasing oxygen (O~2~). Water is split, providing electrons and releasing O~2~ as a by-product. Light energy absorbed by chlorophyll molecules drives the transfer of electrons and hydrogen ions (H+) from water to NADP+, reducing it to NADPH. NADPH stores electrons and provides reducing power to the Calvin cycle. The light reactions also generate ATP from ADP and a phosphate group. The light reactions absorb solar energy and convert it to chemical energy stored in ATP and NADPH. These reactions do not produce sugar; sugar is made during the Calvin cycle, which is the second stage of photosynthesis. The Calvin cycle takes place in the stroma of the chloroplast. It is a series of cyclic reactions that use carbon dioxide (CO2) and the energy-rich products of the light reactions to assemble sugar molecules. The process begins with carbon fixation, where CO~2~ is incorporated into organic compounds. These carbon compounds are then reduced to sugars. NADPH produced by the light reactions provides electrons for reducing carbon compounds in the Calvin cycle. ATP from the light reactions supplies the chemical energy needed for several steps in the Calvin cycle. Although the Calvin cycle is sometimes called the dark reactions or light-independent reactions because it doesn\'t require light directly, it usually occurs during daylight when the light reactions provide NADPH and ATP. The word \"photosynthesis\" encapsulates its two stages. \"Photo,\" from the Greek word for \"light,\" refers to the light reactions, while \"synthesis,\" meaning \"putting together,\" refers to sugar construction by the Calvin cycle. The next sections will delve into these stages in more detail, starting with the properties of light, the energy source that powers photosynthesis. **7.6 Visible radiation absorbed by pigments drives the light reactions** **The Nature of Sunlight** Sunlight is a type of electromagnetic energy or radiation that travels in space as rhythmic waves, like ripples in water. The distance between the crests of these waves is called a wavelength. The electromagnetic spectrum includes a wide range of wavelengths, from very short gamma rays to very long radio waves. Visible light, which ranges from about 380 nm to 750 nm, is only a small part of this spectrum. A prism can separate visible light into its component colors by bending different wavelengths at different angles, like how water droplets in the atmosphere create rainbows. Light behaves both as waves and as discrete packets of energy called photons. A photon has a fixed amount of energy, and shorter wavelengths of light have photons with greater energy. Photons with wavelengths shorter than visible light can damage molecules like proteins and nucleic acids, which is why ultraviolet (UV) radiation can cause sunburns and skin cancer. **Photosynthetic Pigments** In a chloroplast, pigments in the thylakoid membranes absorb certain wavelengths of light and reflect or transmit others. The absorbed wavelengths\' energy is taken in by the pigment molecules, so we don\'t see those colors. Instead, we see the green wavelengths that are not absorbed but are reflected or transmitted by the pigments. This is why leaves appear green to us. Different pigments in chloroplasts absorb light of different wavelengths. Chlorophyll a, which participates directly in light reactions, absorbs mainly blue-violet and red light. Chlorophyll b, a similar molecule, absorbs mainly blue and orange light. Chlorophyll b broadens the range of light a plant can use by conveying absorbed energy to chlorophyll a, which then uses the energy in light reactions. Chloroplasts contain pigments called carotenoids, which are yellow and orange. These pigments contribute to the fall foliage colors when chlorophyll breaks down. Carotenoids broaden the spectrum of light for photosynthesis and provide photoprotection by absorbing and dissipating excessive light energy, preventing damage to chlorophyll and cell molecules. Similar carotenoids in vegetables and fruits protect our eyes. Each type of pigment absorbs certain wavelengths of light because it can absorb the specific amounts of energy in those photons. **Terms to Know** **Mesophyll**-leaf cells specialized for photosynthesis, a leaf's ground tissue system. **Grana***-*A stack of membrane-bounded thylakoids in a chloroplast. Grana are the sites where light energy is trapped by chlorophyll and converted to chemical energy during the light reactions of photosynthesis. **light reactions**-the first of two stages in photosynthesis; the steps in which solar energy is absorbed and converted to chemical energy of ATP and NADPH, releasing oxygen in the process. ------------------------------------------------------------------------------------------------------------------------------------------------------------------------------------------------------------------------- ---------------------------------------------------------------------------------------------------------------------------------------------------------------------------------------------------------------------------------------------- ---------------------------------------------------------------------------------------------------------------------------------------------------------------------------------------------------------------------------------------------------------------------------------------- **Stomata***-*a microscopic pore surrounded by guard cells in the epidermis of a leaf. When stomata are open, CO~2~ enters a leaf, and H~2~O and O~2~ exit. A plant conserves water when its stomata are closed. **Chloroplast***-*An organelle found in plants and algae that absorbs sunlight and uses it to drive the synthesis of organic compounds (sugars) from carbon dioxide and water*.* **Calvin cycle***-*the second of two stages of photosynthesis; a cyclic series of chemical reactions that occur in the stroma of a chloroplast, using the carbon in CO~2~ and ATP and NADPH produced by the light reactions to make energy-rich sugar molecule G3P. **Stroma**-the dense fluid within the chloroplast that surrounds the thylakoid membranes. Sugars are made in the stroma by enzymes of the Calvin cycle. **Chlorophyll**-a green pigment located within the chloroplasts of plants and algae and in the membranes of certain prokaryotes. Chlorophyll-*a* participates directly in the light reactions, which convert solar energy to chemical energy **carbon fixation***-*the incorporation of carbon from atmospheric CO~2~ into an organic compound. During photosynthesis in a C3 plant, carbon is fixed into a three-carbon sugar as it enters the Calvin cycle. In C4 and CAM plants, carbon is first fixed into a four-carbon sugar. **Thylakoids**-a flattened membranous sac inside a chloroplast. Thylakoid membranes contain chlorophyll and the molecular complexes of the light reactions of photosynthesis. A stack of thylakoids is called a granum. **Photosynthesis**-the process by which plants, algae, and some protists and prokaryotes convert light energy to chemical energy that is stored in sugars made from carbon dioxide and water. **7.7 Photosystems capture solar energy** Energy cannot be created or destroyed, only transferred or transformed. When a pigment molecule absorbs a photon of light, one of its electrons jumps to a higher energy level, becoming excited. This excited state is unstable, and the electron quickly returns to its ground state, releasing excess energy as heat. This conversion of light energy to heat explains why black cars get hot on sunny days, as black pigments absorb all wavelengths of light. Some isolated pigments, including chlorophyll, emit light and heat after absorbing photons. When chlorophyll is illuminated, it produces a reddish afterglow called fluorescence. This occurs when an absorbed photon boosts an electron to an excited state, which then drops back to the ground state, emitting energy as heat and light. The key to harvesting light energy is capturing these excited electrons before they return to the ground state, which happens when chlorophyll molecules are embedded in intact chloroplasts. In the thylakoid membrane, chlorophyll molecules are organized into clusters called photosystems. Each photosystem has a reaction-center complex surrounded by light-harvesting complexes. These light-harvesting complexes, made up of various pigment molecules bound to proteins, function as a light-gathering antenna. When a pigment molecule absorbs a photon, the energy is transferred from molecule to molecule until it reaches the reaction-center complex. Here, a pair of special chlorophyll *a* molecules and a primary electron acceptor capture the energy, transforming light energy into chemical energy in the light reactions. Two types of photosystems, photosystem I and photosystem II, cooperate in the light reactions. Despite their names, photosystem II functions first in the sequence. Each photosystem has a unique reaction-center complex. Together, they work to generate ATP and NADPH in light reactions. Compared with a solution of isolated chlorophyll, why do intact chloroplasts not release heat and light when illuminated? In the chloroplasts, a light-excited electron from the reaction-center chlorophyll molecules is passed to a primary electron acceptor before it can fall back to the ground state. **Terms to Know** **Photon**-a fixed quantity of light energy. The shorter the wavelength of light, the greater the energy of a photon. **Wavelength**-the distance between crests of adjacent waves, such as the electromagnetic spectrum. **electromagnetic energy**-the entire spectrum of electromagnetic radiation ranging in wavelength from less than a nanometer to more than a kilometer. **photosystem reaction centre**-a light capturing unit of a chloroplast's thylakoid membrane, consisting of a reaction-center complex surrounded by numerous light-harvesting complexes. Compared with a solution of isolated chlorophyll, why do intact chloroplasts not release heat and light when illuminated? In the chloroplasts, a light-excited electron from the reaction-center chlorophyll molecules is passed to a primary electron acceptor before it can fall back to the ground state. **7.8 Two photosystems connected by an electron transport chain convert light energy to the chemical energy of ATP and NADPH** Light energy boosts an electron of chlorophyll a in the reaction center of a photosystem to an excited state, where it is captured by a primary electron acceptor. The production of ATP and NADPH involves the arrangement of photosystems II and I in the thylakoid membrane, connected by an electron transport chain. Electrons removed from H2O flow through these components to NADPH. The synthesis of ATP is linked to an electron transport chain pumping H+ into a membrane compartment, where the ions flow through an ATP synthase embedded in the membrane. To simplify this system, imagine a mechanical analogy. A large yellow photon mallet boosts an electron from photosystem II to a higher energy level, where it is caught by the primary electron acceptor. The electron then moves down an electron transport chain \"ramp\" to photosystem I, releasing energy to produce ATP. Another photon mallet boosts the electron to a higher energy level in photosystem I, where it is caught by another primary electron acceptor. The photoexcited electrons are then used to produce NADPH. This analogy illustrates how the two photosystems and an electron transport chain transform light energy into the chemical energy of ATP and NADPH. The simple analogy in Figure 7.8 leaves some important questions unanswered: What is the source of the electrons moving through the photosystems to NADPH? How do the light reactions produce O2, and where does that occur? How does the flow of electrons down the ramp produce ATP? In photosynthesis, the electrons that reduce NADP+ to NADPH originate from water. An enzyme in the thylakoid space splits water (H₂O) into two electrons, two hydrogen ions (H⁺), and one oxygen atom (O). The oxygen atom quickly pairs with another oxygen atom to form O₂, which diffuses out of the leaf through the stomata. The electrons from water are transferred to the reaction center chlorophyll *a* molecules in photosystem II, replacing the photoexcited electrons captured by the primary electron acceptor. These electrons then travel through an electron transport chain to photosystem I, where they replace photoexcited electrons captured by its primary electron acceptor. Finally, the electrons are passed through a short electron transport chain to NADP+, reducing it to NADPH. In the light reactions of photosynthesis, ATP is produced through an electron transport chain and chemiosmosis, like the process in cellular respiration. Chemiosmosis involves the potential energy of a concentration gradient of hydrogen ions (H⁺) across a membrane, which powers ATP synthesis. This gradient is created when the electron transport chain uses the energy released from passing electrons to pump H⁺ across the membrane. The energy of the concentration gradient then drives H⁺ back across the membrane through ATP synthase, spinning this rotary motor and phosphorylating ADP to produce ATP. In sum**:** 1. **Light Energy Boosts Electrons**: Light energy excites an electron in chlorophyll a within Photosystem II, raising it to a higher energy level. This excited electron is captured by a primary electron acceptor. 2. **Electron Transport Chain**: The excited electron travels down an electron transport chain, releasing energy that is used to produce ATP. This chain connects Photosystem II to Photosystem I. 3. **Photosystem I**: Another photon of light excites the electron again in Photosystem I, and it is captured by another primary electron acceptor. 4. **NADPH Production**: The re-energized electron is then used to reduce NADP+ to NADPH, which is essential for the Calvin cycle. 5. **Water Splitting**: The electrons that replace the excited electrons in Photosystem II come from water. An enzyme splits water into two electrons, two hydrogen ions (H⁺), and one oxygen atom (O). The oxygen atoms pair up to form O₂, which is released as a byproduct. 6. **ATP Synthesis**: The energy released as electrons move down the electron transport chain is used to pump hydrogen ions (H⁺) into the thylakoid space, creating a concentration gradient. These ions flow back through ATP synthase, a protein in the membrane, driving the production of ATP from ADP. In essence, light energy is converted into chemical energy in the form of ATP and NADPH through a series of steps involving the excitation of electrons, their transport through a chain, and the splitting of water molecules. **7.9 The light reactions take place within the thylakoid membranes** The diagram of the light reactions illustrates how the two photosystems and the electron transport chain are embedded in the thylakoid membrane. Each thylakoid contains numerous copies of these components. Light energy absorbed by the photosystems drives the flow of electrons from water to NADPH. As electrons pass down the electron transport chain, the energy released powers the transport of hydrogen ions (H⁺) into thylakoid space. The concentration gradient of H⁺ across the thylakoid membrane then drives H⁺ through ATP synthase, producing ATP. This process, driven by light energy, is called photophosphorylation. The image illustrates the light-dependent reactions of photosynthesis within the thylakoid membrane of a chloroplast. Here\'s a summary of the key elements: 1. Photosystem II: Absorbs light, exciting electrons that are captured by the primary electron acceptor. Water is split, releasing oxygen and protons (H⁺). 2. Electron Transport Chain: Electrons pass through a series of proteins, pumping H⁺ from the stroma into the thylakoid space, creating a high H⁺ concentration. 3. Photosystem I: Absorbs light, re-exciting electrons which are then passed to NADP+, reducing it to NADPH. 4. ATP Synthase: Utilizes the flow of H⁺ back into the stroma to drive the phosphorylation of ADP to ATP. The diagram highlights the movement of electrons and protons, the splitting of water, and the production of oxygen, ATP, and NADPH. This process is crucial for converting solar energy into chemical energy, essential for the survival of plants and all life on Earth. Describe the two forces moving H+ across the thylakoid membrane. 1\) Energy released as electrons are passed down the electron transport chain pumps H+ into the thylakoid space, and 2) the concentration gradient drives H+ from the thylakoid space through ATP synthase.. **7.10 ATP and NADPH power sugar synthesis in the Calvin cycle** The Calvin cycle operates like a sugar factory within a chloroplast. It uses CO₂ from the air, ATP, and NADPH (both generated by the light reactions) to produce an energy-rich, three-carbon sugar called glyceraldehyde 3-phosphate (G3P). ATP provides energy, while NADPH supplies high-energy electrons for reducing CO₂ to sugar. A plant cell uses G3P to make glucose, sucrose, and other organic molecules as needed. Figure 7.10 outlines the four main steps of the Calvin cycle. It is called a cycle because, like the citric acid cycle in cellular respiration, the starting material is regenerated after molecules enter and leave the cycle. The starting material is a five-carbon sugar named ribulose bisphosphate (RuBP). To make a molecule of glyceraldehyde 3-phosphate (G3P), the cycle must turn three times, incorporating three molecules of CO₂. The cycle starts with three CO₂ molecules to end up with a complete G3P molecule. In step 1 of carbon fixation, the enzyme rubisco attaches CO₂ to RuBP, forming an unstable six-carbon molecule that splits into two three-carbon molecules. In the reduction step, ATP and NADPH are used to reduce the three-carbon molecules to G3P. To complete the cycle, RuBP must be regenerated. In the release step, for every three CO₂ molecules fixed, one G3P molecule leaves the cycle as a product. In the regeneration step, the remaining five G3P molecules are rearranged using energy from ATP to regenerate three molecules of RuBP. For the synthesis of one G3P molecule, the Calvin cycle consumes nine ATP and six NADPH molecules, provided by light reactions. Neither the light reactions nor the Calvin cycle alone can make sugar from CO₂. Photosynthesis is an emergent property of the structural organization of a chloroplast, integrating the two stages of photosynthesis. **Terms to Know** **Photophosphorylation**-the production of ATP by chemiosmosis during light reactions of photosynthesis **Calvin cycle**-The second of two stages of photosynthesis; a cyclic series of chemical reactions that occur in the stroma of a chloroplast, using the carbon in CO~2~ and the ATP and NADPH produced by the light reactions to make the energy-rich sugar molecule G3P.