Chapter 6 Contraction of Skeletal Muscle PDF
Document Details
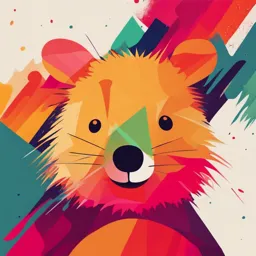
Uploaded by Raelag2502
Uniwersytet Warmińsko-Mazurski w Olsztynie
Tags
Summary
This document details the structure and function of skeletal muscle. It discusses the physiologic anatomy of skeletal muscle fibers, including the sarcolemma, myofibrils, actin, and myosin filaments. The document elucidates the molecular mechanism of muscle contraction, explaining the sliding filament theory.
Full Transcript
CHAPTER 6 UNIT II Contraction of Skeletal Muscle About 40 percent of the 3000 actin...
CHAPTER 6 UNIT II Contraction of Skeletal Muscle About 40 percent of the 3000 actin filaments, which are large polymerized pro- body is skeletal muscle, and tein molecules that are responsible for the actual muscle perhaps another 10 per- contraction. These can be seen in longitudinal view in the cent is smooth and cardiac electron micrograph of Figure 6-2 and are represented muscle. Some of the same diagrammatically in Figure 6-1, parts E through L. The basic principles of contrac- thick filaments in the diagrams are myosin, and the thin tion apply to all three differ- filaments are actin. ent types of muscle. In this chapter, function of skeletal Note in Figure 6-1E that the myosin and actin fila- muscle is considered mainly; the specialized functions of ments partially interdigitate and thus cause the myofi- smooth muscle are discussed in Chapter 8, and cardiac brils to have alternate light and dark bands, as illustrated muscle is discussed in Chapter 9. in Figure 6-2. The light bands contain only actin filaments and are called I bands because they are isotropic to polar- ized light. The dark bands contain myosin filaments, as Physiologic Anatomy of Skeletal Muscle well as the ends of the actin filaments where they over- lap the myosin, and are called A bands because they are Skeletal Muscle Fiber anisotropic to polarized light. Note also the small pro- Figure 6-1 shows the organization of skeletal muscle, jections from the sides of the myosin filaments in Figure demonstrating that all skeletal muscles are composed of 6-1E and L. These are cross-bridges. It is the interaction numerous fibers ranging from 10 to 80 micrometers in between these cross-bridges and the actin filaments that diameter. Each of these fibers is made up of successively causes contraction. smaller subunits, also shown in Figure 6-1 and described Figure 6-1E also shows that the ends of the actin fila- in subsequent paragraphs. ments are attached to a so-called Z disc. From this disc, In most skeletal muscles, each fiber extends the entire these filaments extend in both directions to interdigi- length of the muscle. Except for about 2 percent of the tate with the myosin filaments. The Z disc, which itself fibers, each fiber is usually innervated by only one nerve is composed of filamentous proteins different from the ending, located near the middle of the fiber. actin and myosin filaments, passes crosswise across the The Sarcolemma Is a Thin Membrane Enclosing a myofibril and also crosswise from myofibril to myofibril, Skeletal Muscle Fiber. The sarcolemma consists of a attaching the myofibrils to one another all the way across true cell membrane, called the plasma membrane, and the muscle fiber. Therefore, the entire muscle fiber has an outer coat made up of a thin layer of polysaccharide light and dark bands, as do the individual myofibrils. material that contains numerous thin collagen fibrils. At These bands give skeletal and cardiac muscle their stri- each end of the muscle fiber, this surface layer of the sar- ated appearance. colemma fuses with a tendon fiber. The tendon fibers in The portion of the myofibril (or of the whole muscle turn collect into bundles to form the muscle tendons that fiber) that lies between two successive Z discs is called then insert into the bones. a sarcomere. When the muscle fiber is contracted, as shown at the bottom of Figure 6-5, the length of the sar- Myofibrils Are Composed of Actin and Myosin comere is about 2 micrometers. At this length, the actin Filaments. Each muscle fiber contains several hundred filaments completely overlap the myosin filaments, to several thousand myofibrils, which are demonstrated and the tips of the actin filaments are just beginning to by the many small open dots in the cross-sectional view overlap one another. As discussed later, at this length of Figure 6-1C. Each myofibril (Figure 6-1D and E) is the muscle is capable of generating its greatest force of composed of about 1500 adjacent myosin filaments and contraction. 71 Unit II Membrane Physiology, Nerve, and Muscle SKELETAL MUSCLE A Muscle B C Muscle fasciculus Z disc A I band band Myofibril Muscle fiber Z A I disc band band D H band Z Sarcomere Z G-Actin molecules E H J Myofilaments F-Actin filament K L Myosin filament Myosin molecule M F G H I N Light Heavy meromyosin meromyosin Figure 6-1 Organization of skeletal muscle, from the gross to the molecular level. F, G, H, and I are cross sections at the levels indicated. 72 Chapter 6 Contraction of Skeletal Muscle called sarcoplasm, containing large quantities of potas- sium, magnesium, and phosphate, plus multiple protein enzymes. Also present are tremendous numbers of mito- chondria that lie parallel to the myofibrils. These supply the contracting myofibrils with large amounts of energy UNIT II in the form of adenosine triphosphate (ATP) formed by the mitochondria. Sarcoplasmic Reticulum Is a Specialized Endoplasmic Reticulum of Skeletal Muscle. Also in the sarcoplasm surrounding the myofibrils of each muscle fiber is an extensive reticulum (Figure 6-4), called the sar- coplasmic reticulum. This reticulum has a special organi- zation that is extremely important in controlling muscle Figure 6-2 Electron micrograph of muscle myofibrils showing contraction, as discussed in Chapter 7. The rapidly con- the detailed organization of actin and myosin filaments. Note the tracting types of muscle fibers have especially extensive mitochondria lying between the myofibrils. (From Fawcett DW: The Cell. Philadelphia: WB Saunders, 1981.) sarcoplasmic reticula. Titin Filamentous Molecules Keep the Myosin and General Mechanism of Muscle Contraction Actin Filaments in Place. The side-by-side relation- ship between the myosin and actin filaments is difficult to The initiation and execution of muscle contraction occur maintain. This is achieved by a large number of filamen- in the following sequential steps. tous molecules of a protein called titin (Figure 6-3). Each 1. An action potential travels along a motor nerve to its titin molecule has a molecular weight of about 3 million, endings on muscle fibers. which makes it one of the largest protein molecules in the body. Also, because it is filamentous, it is very springy. 2. At each ending, the nerve secretes a small amount of These springy titin molecules act as a framework that the neurotransmitter substance acetylcholine. holds the myosin and actin filaments in place so that the 3. The acetylcholine acts on a local area of the muscle contractile machinery of the sarcomere will work. One fiber membrane to open multiple “acetylcholine-gated” end of the titin molecule is elastic and is attached to the cation channels through protein molecules floating in Z disk, acting as a spring and changing length as the sar- the membrane. comere contracts and relaxes. The other part of the titin 4. Opening of the acetylcholine-gated channels allows molecule tethers it to the myosin thick filament. The titin large quantities of sodium ions to diffuse to the inte- molecule itself also appears to act as a template for initial rior of the muscle fiber membrane. This causes a formation of portions of the contractile filaments of the local depolarization that in turn leads to opening of sarcomere, especially the myosin filaments. Sarcoplasm Is the Intracellular Fluid Between Myofibrils. The many myofibrils of each muscle fiber are suspended side by side in the muscle fiber. The spaces between the myofibrils are filled with intracellular fluid Myosin (thick filament) M line Titin Actin (thin filament) Z disc Figure 6-4 Sarcoplasmic reticulum in the extracellular spaces Figure 6-3 Organization of proteins in a sarcomere. Each titin between the myofibrils, showing a longitudinal system paralleling molecule extends from the Z disc to the M line. Part of the titin the myofibrils. Also shown in cross section are T tubules (arrows) that molecule is closely associated with the myosin thick filament, lead to the exterior of the fiber membrane and are important for whereas the rest of the molecule is springy and changes length as conducting the electrical signal into the center of the muscle fiber. the sarcomere contracts and relaxes. (From Fawcett DW: The Cell. Philadelphia: WB Saunders, 1981.) 73 Unit II Membrane Physiology, Nerve, and Muscle voltage-gated sodium channels. This initiates an action maximum extent. Also, the Z discs have been pulled by potential at the membrane. the actin filaments up to the ends of the myosin fila- 5. The action potential travels along the muscle fiber ments. Thus, muscle contraction occurs by a sliding fila- membrane in the same way that action potentials travel ment mechanism. along nerve fiber membranes. But what causes the actin filaments to slide inward among the myosin filaments? This is caused by forces 6. The action potential depolarizes the muscle mem- generated by interaction of the cross-bridges from the brane, and much of the action potential electricity myosin filaments with the actin filaments. Under resting flows through the center of the muscle fiber. Here it conditions, these forces are inactive. But when an action causes the sarcoplasmic reticulum to release large potential travels along the muscle fiber, this causes the quantities of calcium ions that have been stored within sarcoplasmic reticulum to release large quantities of cal- this reticulum. cium ions that rapidly surround the myofibrils. The cal- 7. The calcium ions initiate attractive forces between cium ions in turn activate the forces between the myosin the actin and myosin filaments, causing them to slide and actin filaments, and contraction begins. But energy alongside each other, which is the contractile process. is needed for the contractile process to proceed. This 8. After a fraction of a second, the calcium ions are energy comes from high-energy bonds in the ATP pumped back into the sarcoplasmic reticulum by a molecule, which is degraded to adenosine diphosphate Ca++ membrane pump and remain stored in the reticu- (ADP) to liberate the energy. In the next few sections, lum until a new muscle action potential comes along; we describe what is known about the details of these this removal of calcium ions from the myofibrils causes molecular processes of contraction. the muscle contraction to cease. Molecular Characteristics of the Contractile We now describe the molecular machinery of the mus- Filaments cle contractile process. Myosin Filaments Are Composed of Multiple Myosin Molecules. Each of the myosin molecules, shown in Figure 6-6A, has a molecular weight of about 480,000. Molecular Mechanism of Muscle Figure 6-6B shows the organization of many molecules Contraction to form a myosin filament, as well as interaction of this filament on one side with the ends of two actin filaments. Sliding Filament Mechanism of Muscle Con- The myosin molecule (see Figure 6-6A) is composed traction. Figure 6-5 demonstrates the basic mecha- of six polypeptide chains—two heavy chains, each with a nism of muscle contraction. It shows the relaxed state of molecular weight of about 200,000, and four light chains a sarcomere (top) and the contracted state (bottom). In with molecular weights of about 20,000 each. The two heavy the relaxed state, the ends of the actin filaments extend- chains wrap spirally around each other to form a double ing from two successive Z discs barely begin to overlap one another. Conversely, in the contracted state, these actin filaments have been pulled inward among the myo- Head sin filaments, so their ends overlap one another to their Tail I A I Z Z Two heavy chains A Light chains Relaxed Actin filaments I A I Z Z Cross-bridges Hinges Body Contracted B Myosin filament Figure 6-5 Relaxed and contracted states of a myofibril showing Figure 6-6 A, Myosin molecule. B, Combination of many myosin (top) sliding of the actin filaments (pink) into the spaces between molecules to form a myosin filament. Also shown are thousands the myosin filaments (red) and (bottom) pulling of the Z mem- of myosin cross-bridges and interaction between the heads of the branes toward each other. cross-bridges with adjacent actin filaments. 74 Chapter 6 Contraction of Skeletal Muscle helix, which is called the tail of the myosin molecule. One Active sites Troponin complex end of each of these chains is folded bilaterally into a globu- lar polypeptide structure called a myosin head. Thus, there are two free heads at one end of the double-helix myosin molecule. The four light chains are also part of the myosin UNIT II head, two to each head. These light chains help control the F-actin Tropomyosin function of the head during muscle contraction. Figure 6-7 Actin filament, composed of two helical strands of The myosin filament is made up of 200 or more individ- F-actin molecules and two strands of tropomyosin molecules that ual myosin molecules. The central portion of one of these fit in the grooves between the actin strands. Attached to one end filaments is shown in Figure 6-6B, displaying the tails of the of each tropomyosin molecule is a troponin complex that initiates contraction. myosin molecules bundled together to form the body of the filament, while many heads of the molecules hang outward to the sides of the body. Also, part of the body of each myo- Each actin filament is about 1 micrometer long. The sin molecule hangs to the side along with the head, thus bases of the actin filaments are inserted strongly into the providing an arm that extends the head outward from the Z discs; the ends of the filaments protrude in both direc- body, as shown in the figure. The protruding arms and tions to lie in the spaces between the myosin molecules, heads together are called cross-bridges. Each cross-bridge as shown in Figure 6-5. is flexible at two points called hinges—one where the arm leaves the body of the myosin filament, and the other where Tropomyosin Molecules. The actin filament also the head attaches to the arm. The hinged arms allow the contains another protein, tropomyosin. Each molecule of heads to be either extended far outward from the body tropomyosin has a molecular weight of 70,000 and a length of the myosin filament or brought close to the body. The of 40 nanometers. These molecules are wrapped spirally hinged heads in turn participate in the actual contraction around the sides of the F-actin helix. In the resting state, process, as discussed in the following sections. the tropomyosin molecules lie on top of the active sites of The total length of each myosin filament is uniform, the actin strands so that attraction cannot occur between the almost exactly 1.6 micrometers. Note, however, that there actin and myosin filaments to cause contraction. are no cross-bridge heads in the center of the myosin fila- ment for a distance of about 0.2 micrometer because the Troponin and Its Role in Muscle Contraction. hinged arms extend away from the center. Attached intermittently along the sides of the tropomy- Now, to complete the picture, the myosin filament itself osin molecules are still other protein molecules called is twisted so that each successive pair of cross-bridges is troponin. These are actually complexes of three loosely axially displaced from the previous pair by 120 degrees. bound protein subunits, each of which plays a specific This ensures that the cross-bridges extend in all direc- role in controlling muscle contraction. One of the sub- tions around the filament. units (troponin I) has a strong affinity for actin, another (troponin T) for tropomyosin, and a third (troponin C) ATPase Activity of the Myosin Head. Another fea- for calcium ions. This complex is believed to attach the ture of the myosin head that is essential for muscle contrac- tropomyosin to the actin. The strong affinity of the tro- tion is that it functions as an ATPase enzyme. As explained ponin for calcium ions is believed to initiate the contrac- later, this property allows the head to cleave ATP and use tion process, as explained in the next section. the energy derived from the ATP’s high-energy phosphate bond to energize the contraction process. Interaction of One Myosin Filament, Two Actin Actin Filaments Are Composed of Actin, Filaments, and Calcium Ions to Cause Contraction Tropomyosin, and Troponin. The backbone of the actin Inhibition of the Actin Filament by the Troponin- filament is a double-stranded F-actin protein molecule, rep- Tropomyosin Complex; Activation by Calcium resented by the two lighter-colored strands in Figure 6-7. Ions. A pure actin filament without the presence of the The two strands are wound in a helix in the same manner troponin-tropomyosin complex (but in the presence of as the myosin molecule. magnesium ions and ATP) binds instantly and strongly Each strand of the double F-actin helix is composed with the heads of the myosin molecules. Then, if the tro- of polymerized G-actin molecules, each having a molecu- ponin-tropomyosin complex is added to the actin fila- lar weight of about 42,000. Attached to each one of the ment, the binding between myosin and actin does not G-actin molecules is one molecule of ADP. It is believed take place. Therefore, it is believed that the active sites that these ADP molecules are the active sites on the actin on the normal actin filament of the relaxed muscle are filaments with which the cross-bridges of the myosin fil- inhibited or physically covered by the troponin-tropomy- aments interact to cause muscle contraction. The active osin complex. Consequently, the sites cannot attach to the sites on the two F-actin strands of the double helix are heads of the myosin filaments to cause contraction. Before staggered, giving one active site on the overall actin fila- contraction can take place, the inhibitory effect of the ment about every 2.7 nanometers. troponin-tropomyosin complex must itself be inhibited. 75 Unit II Membrane Physiology, Nerve, and Muscle This brings us to the role of the calcium ions. In the amount of work performed by the muscle, the greater the presence of large amounts of calcium ions, the inhibitory amount of ATP that is cleaved, which is called the Fenn effect of the troponin-tropomyosin on the actin filaments effect. The following sequence of events is believed to be is itself inhibited. The mechanism of this is not known, the means by which this occurs: but one suggestion is the following: When calcium ions 1. Before contraction begins, the heads of the cross- combine with troponin C, each molecule of which can bridges bind with ATP. The ATPase activity of the bind strongly with up to four calcium ions, the troponin myosin head immediately cleaves the ATP but leaves complex supposedly undergoes a conformational change the cleavage products, ADP plus phosphate ion, bound that in some way tugs on the tropomyosin molecule and to the head. In this state, the conformation of the head moves it deeper into the groove between the two actin is such that it extends perpendicularly toward the actin strands. This “uncovers” the active sites of the actin, thus filament but is not yet attached to the actin. allowing these to attract the myosin cross-bridge heads and cause contraction to proceed. Although this is a 2. When the troponin-tropomyosin complex binds with hypothetical mechanism, it does emphasize that the nor- calcium ions, active sites on the actin filament are mal relation between the troponin-tropomyosin complex uncovered and the myosin heads then bind with these, and actin is altered by calcium ions, producing a new con- as shown in Figure 6-8. dition that leads to contraction. 3. The bond between the head of the cross-bridge and Interaction Between the “Activated” Actin Filament the active site of the actin filament causes a conforma- and the Myosin Cross-Bridges—The “Walk-Along” tional change in the head, prompting the head to tilt Theory of Contraction. As soon as the actin filament toward the arm of the cross-bridge. This provides the becomes activated by the calcium ions, the heads of power stroke for pulling the actin filament. The energy the cross-bridges from the myosin filaments become that activates the power stroke is the energy already attracted to the active sites of the actin filament, and stored, like a “cocked” spring, by the conformational this, in some way, causes contraction to occur. Although change that occurred in the head when the ATP mol- the precise manner by which this interaction between ecule was cleaved earlier. the cross-bridges and the actin causes contraction is still 4. Once the head of the cross-bridge tilts, this allows partly theoretical, one hypothesis for which considerable release of the ADP and phosphate ion that were pre- evidence exists is the “walk-along” theory (or “ratchet” viously attached to the head. At the site of release of theory) of contraction. the ADP, a new molecule of ATP binds. This binding Figure 6-8 demonstrates this postulated walk-along of new ATP causes detachment of the head from the mechanism for contraction. The figure shows the heads actin. of two cross-bridges attaching to and disengaging from 5. After the head has detached from the actin, the new active sites of an actin filament. It is postulated that when molecule of ATP is cleaved to begin the next cycle, a head attaches to an active site, this attachment simul- leading to a new power stroke. That is, the energy taneously causes profound changes in the intramolecular again “cocks” the head back to its perpendicular condi- forces between the head and arm of its cross-bridge. The tion, ready to begin the new power stroke cycle. new alignment of forces causes the head to tilt toward the arm and to drag the actin filament along with it. This tilt 6. When the cocked head (with its stored energy derived of the head is called the power stroke. Then, immediately from the cleaved ATP) binds with a new active site after tilting, the head automatically breaks away from the on the actin filament, it becomes uncocked and once active site. Next, the head returns to its extended direc- again provides a new power stroke. tion. In this position, it combines with a new active site Thus, the process proceeds again and again until the farther down along the actin filament; then the head tilts actin filaments pull the Z membrane up against the ends again to cause a new power stroke, and the actin filament of the myosin filaments or until the load on the muscle moves another step. Thus, the heads of the cross-bridges becomes too great for further pulling to occur. bend back and forth and step by step walk along the actin filament, pulling the ends of two successive actin fila- Movement Active sites Actin filament ments toward the center of the myosin filament. Each one of the cross-bridges is believed to operate independently of all others, each attaching and pulling in Power a continuous repeated cycle. Therefore, the greater the Hinges stroke number of cross-bridges in contact with the actin filament at any given time, the greater the force of contraction. ATP as the Source of Energy for Contraction— Chemical Events in the Motion of the Myosin Heads. When a muscle contracts, work is performed and Myosin filament energy is required. Large amounts of ATP are cleaved to Figure 6-8 “Walk-along” mechanism for contraction of the form ADP during the contraction process; the greater the muscle. 76 Chapter 6 Contraction of Skeletal Muscle The Amount of Actin and Myosin Filament Normal range of contraction Overlap Determines Tension Developed by the Contracting Muscle Tension during Figure 6-9 shows the effect of sarcomere length and contraction Tension of muscle amount of myosin-actin filament overlap on the active UNIT II Increase in tension tension developed by a contracting muscle fiber. To the during contraction right, shown in black, are different degrees of overlap of the myosin and actin filaments at different sarcomere lengths. At point D on the diagram, the actin filament has pulled Tension all the way out to the end of the myosin filament, with before contraction no actin-myosin overlap. At this point, the tension devel- oped by the activated muscle is zero. Then, as the sarco- 0 mere shortens and the actin filament begins to overlap the 1/2 Normal 2 myosin filament, the tension increases progressively until normal normal the sarcomere length decreases to about 2.2 micrometers. Length At this point, the actin filament has already overlapped Figure 6-10 Relation of muscle length to tension in the muscle all the cross-bridges of the myosin filament but has not both before and during muscle contraction. yet reached the center of the myosin filament. With fur- ther shortening, the sarcomere maintains full tension amount of connective tissue in it; also, the sarcomeres in until point B is reached, at a sarcomere length of about different parts of the muscle do not always contract the 2 micrometers. At this point, the ends of the two actin fil- same amount. Therefore, the curve has somewhat dif- aments begin to overlap each other in addition to overlap- ferent dimensions from those shown for the individual ping the myosin filaments. As the sarcomere length falls muscle fiber, but it exhibits the same general form for from 2 micrometers down to about 1.65 micrometers, at the slope in the normal range of contraction, as noted in point A, the strength of contraction decreases rapidly. At Figure 6-10. this point, the two Z discs of the sarcomere abut the ends Note in Figure 6-10 that when the muscle is at its nor- of the myosin filaments. Then, as contraction proceeds mal resting length, which is at a sarcomere length of about to still shorter sarcomere lengths, the ends of the myosin 2 micrometers, it contracts upon activation with the filaments are crumpled and, as shown in the figure, the approximate maximum force of contraction. However, the strength of contraction approaches zero, but the sarco- increase in tension that occurs during contraction, called mere has now contracted to its shortest length. active tension, decreases as the muscle is stretched beyond its normal length—that is, to a sarcomere length greater Effect of Muscle Length on Force of Contraction in than about 2.2 micrometers. This is demonstrated by the the Whole Intact Muscle. The top curve of Figure 6-10 decreased length of the arrow in the figure at greater than is similar to that in Figure 6-9, but the curve in Figure 6-10 normal muscle length. depicts tension of the intact, whole muscle rather than of a single muscle fiber. The whole muscle has a large Relation of Velocity of Contraction to Load A skeletal muscle contracts rapidly when it contracts against no load—to a state of full contraction in about 0.1 D second for the average muscle. When loads are applied, the B C 100 C velocity of contraction becomes progressively less as the B load increases, as shown in Figure 6-11. That is, when the A A Tension developed Velocity of contraction (cm/sec) 30 (percent) 50 20 D 0 10 0 1 2 3 4 Length of sarcomere (micrometers) Figure 6-9 Length-tension diagram for a single fully contracted 0 sarcomere, showing maximum strength of contraction when the 0 1 2 3 4 sarcomere is 2.0 to 2.2 micrometers in length. At the upper right are the relative positions of the actin and myosin filaments at dif- Load-opposing contraction (kg) ferent sarcomere lengths from point A to point D. (Modified from Figure 6-11 Relation of load to velocity of contraction in a skele- Gordon AM, Huxley AF, Julian FJ: The length-tension diagram of tal muscle with a cross section of 1 square centimeter and a length single vertebrate striated muscle fibers. J Physiol 171:28P, 1964.) of 8 centimeters. 77 Unit II Membrane Physiology, Nerve, and Muscle load has been increased to equal the maximum force that of phosphocreatine in the muscle fiber is also very little— the muscle can exert, the velocity of contraction becomes only about five times as great as the ATP. Therefore, the zero and no contraction results, despite activation of the combined energy of both the stored ATP and the phos- muscle fiber. phocreatine in the muscle is capable of causing maximal This decreasing velocity of contraction with load is muscle contraction for only 5 to 8 seconds. caused by the fact that a load on a contracting muscle is a The second important source of energy, which is used reverse force that opposes the contractile force caused by muscle contraction. Therefore, the net force that is avail- to reconstitute both ATP and phosphocreatine, is “gly- able to cause velocity of shortening is correspondingly colysis” of glycogen previously stored in the muscle cells. reduced. Rapid enzymatic breakdown of the glycogen to pyruvic acid and lactic acid liberates energy that is used to convert Energetics of Muscle Contraction ADP to ATP; the ATP can then be used directly to ener- gize additional muscle contraction and also to re-form the Work Output During Muscle Contraction stores of phosphocreatine. The importance of this glycolysis mechanism is two- When a muscle contracts against a load, it performs work. fold. First, the glycolytic reactions can occur even in the This means that energy is transferred from the muscle to absence of oxygen, so muscle contraction can be sus- the external load to lift an object to a greater height or to tained for many seconds and sometimes up to more than overcome resistance to movement. a minute, even when oxygen delivery from the blood is In mathematical terms, work is defined by the follow- not available. Second, the rate of formation of ATP by the ing equation: glycolytic process is about 2.5 times as rapid as ATP for- W=L×D mation in response to cellular foodstuffs reacting with oxygen. However, so many end products of glycolysis in which W is the work output, L is the load, and D is accumulate in the muscle cells that glycolysis also loses its the distance of movement against the load. The energy capability to sustain maximum muscle contraction after required to perform the work is derived from the chem- about 1 minute. ical reactions in the muscle cells during contraction, as The third and final source of energy is oxidative described in the following sections. metabolism. This means combining oxygen with the Sources of Energy for Muscle Contraction end products of glycolysis and with various other cellu- lar foodstuffs to liberate ATP. More than 95 percent of We have already seen that muscle contraction depends on all energy used by the muscles for sustained, long-term energy supplied by ATP. Most of this energy is required contraction is derived from this source. The foodstuffs to actuate the walk-along mechanism by which the cross- that are consumed are carbohydrates, fats, and protein. bridges pull the actin filaments, but small amounts are For extremely long-term maximal muscle activity—over required for (1) pumping calcium ions from the sarco- a period of many hours—by far the greatest proportion plasm into the sarcoplasmic reticulum after the contrac- of energy comes from fats, but for periods of 2 to 4 hours, tion is over and (2) pumping sodium and potassium ions as much as one half of the energy can come from stored through the muscle fiber membrane to maintain appro- carbohydrates. priate ionic environment for propagation of muscle fiber The detailed mechanisms of these energetic processes action potentials. are discussed in Chapters 67 through 72. In addition, the The concentration of ATP in the muscle fiber, about importance of the different mechanisms of energy release 4 millimolar, is sufficient to maintain full contraction during performance of different sports is discussed in for only 1 to 2 seconds at most. The ATP is split to Chapter 84 on sports physiology. form ADP, which transfers energy from the ATP mol- ecule to the contracting machinery of the muscle fiber. Efficiency of Muscle Contraction. The efficiency of an Then, as described in Chapter 2, the ADP is rephospho- engine or a motor is calculated as the percentage of energy rylated to form new ATP within another fraction of a input that is converted into work instead of heat. The second, which allows the muscle to continue its con- percentage of the input energy to muscle (the chemical traction. There are several sources of the energy for this energy in nutrients) that can be converted into work, even rephosphorylation. under the best conditions, is less than 25 percent, with the The first source of energy that is used to reconstitute remainder becoming heat. The reason for this low effi- the ATP is the substance phosphocreatine, which carries a ciency is that about one half of the energy in foodstuffs is lost during the formation of ATP, and even then, only 40 to high-energy phosphate bond similar to the bonds of ATP. 45 percent of the energy in the ATP itself can later be con- The high-energy phosphate bond of phosphocreatine has verted into work. a slightly higher amount of free energy than that of each Maximum efficiency can be realized only when the mus- ATP bond, as is discussed more fully in Chapters 67 and cle contracts at a moderate velocity. If the muscle contracts 72. Therefore, phosphocreatine is instantly cleaved, and its slowly or without any movement, small amounts of main- released energy causes bonding of a new phosphate ion to tenance heat are released during contraction, even though ADP to reconstitute the ATP. However, the total amount little or no work is performed, thereby decreasing the con- 78 Chapter 6 Contraction of Skeletal Muscle version efficiency to as little as zero. Conversely, if contrac- Duration of tion is too rapid, large proportions of the energy are used to depolarization overcome viscous friction within the muscle itself, and this, Force of contraction too, reduces the efficiency of contraction. Ordinarily, maxi- mum efficiency is developed when the velocity of contrac- Soleus UNIT II tion is about 30 percent of maximum. Gastrocnemius Characteristics of Whole Muscle Contraction Ocular muscle Many features of muscle contraction can be demonstrated by eliciting single muscle twitches. This can be accomplished by 0 40 80 120 160 200 instantaneous electrical excitation of the nerve to a muscle Milliseconds or by passing a short electrical stimulus through the muscle Figure 6-13 Duration of isometric contractions for different types itself, giving rise to a single, sudden contraction lasting for a of mammalian skeletal muscles, showing a latent period between fraction of a second. the action potential (depolarization) and muscle contraction. Isometric Versus Isotonic Contraction. Muscle contrac- tion is said to be isometric when the muscle does not shorten during contraction and isotonic when it does shorten but the Figure 6-13 shows records of isometric contractions of tension on the muscle remains constant throughout the con- three types of skeletal muscle: an ocular muscle, which has traction. Systems for recording the two types of muscle con- a duration of isometric contraction of less than 1/50 second; traction are shown in Figure 6-12. the gastrocnemius muscle, which has a duration of contrac- In the isometric system, the muscle contracts against tion of about 1/15 second; and the soleus muscle, which has a force transducer without decreasing the muscle length, a duration of contraction of about 1/5 second. It is interesting as shown on the right in Figure 6-12. In the isotonic sys- that these durations of contraction are adapted to the func- tem, the muscle shortens against a fixed load; this is illus- tions of the respective muscles. Ocular movements must be trated on the left in the figure, showing a muscle lifting a extremely rapid to maintain fixation of the eyes on specific pan of weights. The characteristics of isotonic contraction objects to provide accuracy of vision. The gastrocnemius depend on the load against which the muscle contracts, as muscle must contract moderately rapidly to provide suffi- well as the inertia of the load. However, the isometric sys- cient velocity of limb movement for running and jumping, tem records strictly changes in force of muscle contraction and the soleus muscle is concerned principally with slow itself. Therefore, the isometric system is most often used contraction for continual, long-term support of the body when comparing the functional characteristics of different against gravity. muscle types. Fast Versus Slow Muscle Fibers. As we discuss more fully Characteristics of Isometric Twitches Recorded from in Chapter 84 on sports physiology, every muscle of the body Different Muscles. The human body has many sizes of is composed of a mixture of so-called fast and slow muscle skeletal muscles—from the small stapedius muscle in the fibers, with still other fibers gradated between these two middle ear, measuring only a few millimeters long and a extremes. Muscles that react rapidly, including anterior tibia- millimeter or so in diameter, up to the large quadriceps lis, are composed mainly of “fast” fibers with only small num- muscle, a half million times as large as the stapedius. bers of the slow variety. Conversely, muscles such as soleus Further, the fibers may be as small as 10 micrometers in that respond slowly but with prolonged contraction are com- diameter or as large as 80 micrometers. Finally, the ener- posed mainly of “slow” fibers. The differences between these getics of muscle contraction vary considerably from one two types of fibers are as follows. muscle to another. Therefore, it is no wonder that the Slow Fibers (Type 1, Red Muscle). (1) Smaller fibers. (2) mechanical characteristics of muscle contraction differ Also innervated by smaller nerve fibers. (3) More extensive among muscles. blood vessel system and capillaries to supply extra amounts of oxygen. (4) Greatly increased numbers of mitochondria, also to support high levels of oxidative metabolism. (5) Fibers contain large amounts of myoglobin, an iron-containing pro- Stimulating Stimulating tein similar to hemoglobin in red blood cells. Myoglobin electrodes electrodes combines with oxygen and stores it until needed; this also greatly speeds oxygen transport to the mitochondria. The Kymograph myoglobin gives the slow muscle a reddish appearance and Muscle the name red muscle. Fast Fibers (Type II, White Muscle). (1) Large fibers for great strength of contraction. (2) Extensive sarcoplasmic Electronic force reticulum for rapid release of calcium ions to initiate con- Weights transducer traction. (3) Large amounts of glycolytic enzymes for rapid To electronic release of energy by the glycolytic process. (4) Less extensive recorder blood supply because oxidative metabolism is of secondary Isotonic system Isometric system importance. (5) Fewer mitochondria, also because oxidative Figure 6-12 Isotonic and isometric systems for recording muscle metabolism is secondary. A deficit of red myoglobin in fast contractions. muscle gives it the name white muscle. 79 Unit II Membrane Physiology, Nerve, and Muscle Mechanics of Skeletal Muscle Contraction Strength of muscle contraction Motor Unit—All the Muscle Fibers Innervated by a Single Nerve Fiber. Each motoneuron that leaves the spinal cord Tetanization innervates multiple muscle fibers, the number depending on the type of muscle. All the muscle fibers innervated by a single nerve fiber are called a motor unit. In general, small muscles that react rapidly and whose control must be exact have more nerve fibers for fewer muscle fibers (for instance, as few as two or three muscle fibers per motor unit in some of the laryngeal muscles). Conversely, large muscles that do not require fine control, such as the soleus muscle, may have several hundred muscle fibers in a motor unit. An average 5 10 15 20 25 30 35 40 45 50 55 figure for all the muscles of the body is questionable, but Rate of stimulation (times per second) a good guess would be about 80 to 100 muscle fibers to a Figure 6-14 Frequency summation and tetanization. motor unit. The muscle fibers in each motor unit are not all bunched together in the muscle but overlap other motor units in maximum, so any additional increase in frequency beyond microbundles of 3 to 15 fibers. This interdigitation allows the that point has no further effect in increasing contractile force. separate motor units to contract in support of one another This occurs because enough calcium ions are maintained in rather than entirely as individual segments. the muscle sarcoplasm, even between action potentials, so Muscle Contractions of Different Force—Force Sum- that full contractile state is sustained without allowing any mation. Summation means the adding together of indi- relaxation between the action potentials. vidual twitch contractions to increase the intensity of Maximum Strength of Contraction. The maximum overall muscle contraction. Summation occurs in two strength of tetanic contraction of a muscle operating at a ways: (1) by increasing the number of motor units con- normal muscle length averages between 3 and 4 kilograms tracting simultaneously, which is called multiple fiber per square centimeter of muscle, or 50 pounds per square summation, and (2) by increasing the frequency of con- inch. Because a quadriceps muscle can have up to 16 square traction, which is called frequency summation and can inches of muscle belly, as much as 800 pounds of tension lead to tetanization. may be applied to the patellar tendon. Thus, one can readily Multiple Fiber Summation. When the central nervous understand how it is possible for muscles to pull their ten- system sends a weak signal to contract a muscle, the smaller dons out of their insertions in bone. motor units of the muscle may be stimulated in preference Changes in Muscle Strength at the Onset of Contraction— to the larger motor units. Then, as the strength of the signal The Staircase Effect (Treppe). When a muscle begins to increases, larger and larger motor units begin to be excited as contract after a long period of rest, its initial strength of well, with the largest motor units often having as much as 50 contraction may be as little as one-half its strength 10 to 50 times the contractile force of the smallest units. This is called muscle twitches later. That is, the strength of contraction the size principle. It is important because it allows the grada- increases to a plateau, a phenomenon called the staircase tions of muscle force during weak contraction to occur in effect, or treppe. small steps, whereas the steps become progressively greater Although all the possible causes of the staircase effect are when large amounts of force are required. The cause of this not known, it is believed to be caused primarily by increas- size principle is that the smaller motor units are driven by ing calcium ions in the cytosol because of the release of more small motor nerve fibers, and the small motoneurons in the and more ions from the sarcoplasmic reticulum with each spinal cord are more excitable than the larger ones, so natu- successive muscle action potential and failure of the sarco- rally they are excited first. plasm to recapture the ions immediately. Another important feature of multiple fiber summation is Skeletal Muscle Tone. Even when muscles are at rest, a that the different motor units are driven asynchronously by certain amount of tautness usually remains. This is called the spinal cord, so contraction alternates among motor units muscle tone. Because normal skeletal muscle fibers do not one after the other, thus providing smooth contraction even contract without an action potential to stimulate the fibers, at low frequencies of nerve signals. skeletal muscle tone results entirely from a low rate of nerve Frequency Summation and Tetanization. Figure 6-14 impulses coming from the spinal cord. These, in turn, are shows the principles of frequency summation and tetaniza- controlled partly by signals transmitted from the brain to the tion. To the left are displayed individual twitch contractions appropriate spinal cord anterior motoneurons and partly by occurring one after another at low frequency of stimulation. signals that originate in muscle spindles located in the mus- Then, as the frequency increases, there comes a point where cle itself. Both of these are discussed in relation to muscle each new contraction occurs before the preceding one is over. spindle and spinal cord function in Chapter 54. As a result, the second contraction is added partially to the Muscle Fatigue. Prolonged and strong contraction of first, so the total strength of contraction rises progressively a muscle leads to the well-known state of muscle fatigue. with increasing frequency. When the frequency reaches a Studies in athletes have shown that muscle fatigue increases critical level, the successive contractions eventually become in almost direct proportion to the rate of depletion of muscle so rapid that they fuse together and the whole muscle con- glycogen. Therefore, fatigue results mainly from inability of traction appears to be completely smooth and continuous, as the contractile and metabolic processes of the muscle fibers shown in the figure. This is called tetanization. At a slightly to continue supplying the same work output. However, exper- higher frequency, the strength of contraction reaches its iments have also shown that transmission of the nerve signal 80 Chapter 6 Contraction of Skeletal Muscle through the neuromuscular junction, which is discussed in is called coactivation of the agonist and antagonist muscles, Chapter 7, can diminish at least a small amount after intense and it is controlled by the motor control centers of the brain prolonged muscle activity, thus further diminishing muscle and spinal cord. contraction. Interruption of blood flow through a contract- The position of each separate part of the body, such as ing muscle leads to almost complete muscle fatigue within 1 an arm or a leg, is determined by the relative degrees of UNIT II or 2 minutes because of the loss of nutrient supply, especially contraction of the agonist and antagonist sets of muscles. loss of oxygen. For instance, let us assume that an arm or a leg is to be Lever Systems of the Body. Muscles operate by apply- placed in a midrange position. To achieve this, agonist and ing tension to their points of insertion into bones, and the antagonist muscles are excited about equally. Remember bones in turn form various types of lever systems. Figure that an elongated muscle contracts with more force than a 6-15 shows the lever system activated by the biceps muscle shortened muscle, which was demonstrated in Figure 6-10, to lift the forearm. If we assume that a large biceps muscle showing maximum strength of contraction at full func- has a cross-sectional area of 6 square inches, the maximum tional muscle length and almost no strength of contraction force of contraction would be about 300 pounds. When the at half-normal length. Therefore, the elongated muscle on forearm is at right angles with the upper arm, the tendon one side of a joint can contract with far greater force than attachment of the biceps is about 2 inches anterior to the ful- the shorter muscle on the opposite side. As an arm or leg crum at the elbow and the total length of the forearm lever moves toward its midposition, the strength of the longer is about 14 inches. Therefore, the amount of lifting power muscle decreases, whereas the strength of the shorter mus- of the biceps at the hand would be only one seventh of the cle increases until the two strengths equal each other. At 300 pounds of muscle force, or about 43 pounds. When the this point, movement of the arm or leg stops. Thus, by vary- arm is fully extended, the attachment of the biceps is much ing the ratios of the degree of activation of the agonist and less than 2 inches anterior to the fulcrum and the force with antagonist muscles, the nervous system directs the posi- which the hand can be brought forward is also much less tioning of the arm or leg. than 43 pounds. We learn in Chapter 54 that the motor nervous system In short, an analysis of the lever systems of the body has additional important mechanisms to compensate for dif- depends on knowledge of (1) the point of muscle inser- ferent muscle loads when directing this positioning process. tion, (2) its distance from the fulcrum of the lever, (3) the length of the lever arm, and (4) the position of the lever. Remodeling of Muscle to Match Function Many types of movement are required in the body, some of All the muscles of the body are continually being remodeled which need great strength and others of which need large to match the functions that are required of them. Their diam- distances of movement. For this reason, there are many dif- eters are altered, their lengths are altered, their strengths are ferent types of muscle; some are long and contract a long altered, their vascular supplies are altered, and even the types distance, and some are short but have large cross-sectional of muscle fibers are altered at least slightly. This remodel- areas and can provide extreme strength of contraction ing process is often quite rapid, within a few weeks. Indeed, over short distances. The study of different types of mus- experiments in animals have shown that muscle contrac- cles, lever systems, and their movements is called kinesi- tile proteins in some smaller, more active muscles can be ology and is an important scientific component of human replaced in as little as 2 weeks. physioanatomy. Muscle Hypertrophy and Muscle Atrophy. When the total “Positioning” of a Body Part by Contraction of Agonist mass of a muscle increases, this is called muscle hypertrophy. and Antagonist Muscles on Opposite Sides of a Joint— When it decreases, the process is called muscle atrophy. “Coactivation” of Antagonist Muscles. Virtually all body Virtually all muscle hypertrophy results from an movements are caused by simultaneous contraction of ago- increase in the number of actin and myosin filaments in nist and antagonist muscles on opposite sides of joints. This each muscle fiber, causing enlargement of the individ- ual muscle fibers; this is called simply fiber hypertrophy. Hypertrophy occurs to a much greater extent when the muscle is loaded during the contractile process. Only a few strong contractions each day are required to cause signifi- cant hypertrophy within 6 to 10 weeks. The manner in which forceful contraction leads to hyper- trophy is not known. It is known, however, that the rate of synthesis of muscle contractile proteins is far greater when hypertrophy is developing, leading also to progressively greater numbers of both actin and myosin filaments in the myofibrils, often increasing as much as 50 percent. In turn, some of the myofibrils themselves have been observed to split within hypertrophying muscle to form new myofibrils, but how important this is in usual muscle hypertrophy is still unknown. Along with the increasing size of myofibrils, the enzyme systems that provide energy also increase. This is especially true of the enzymes for glycolysis, allowing rapid supply of energy during short-term forceful muscle Figure 6-15 Lever system activated by the biceps muscle. contraction. 81 Unit II Membrane Physiology, Nerve, and Muscle When a muscle remains unused for many weeks, the rate Recovery of Muscle Contraction in Poliomyelitis: of degradation of the contractile proteins is more rapid than Development of Macromotor Units. When some but not the rate of replacement. Therefore, muscle atrophy occurs. all nerve fibers to a muscle are destroyed, as commonly The pathway that appears to account for much of the pro- occurs in poliomyelitis, the remaining nerve fibers branch off tein degradation in a muscle undergoing atrophy is the ATP- to form new axons that then innervate many of the paralyzed dependent ubiquitin-proteasome pathway. Proteasomes are muscle fibers. This causes large motor units called macromo- large protein complexes that degrade damaged or unneeded tor units, which can contain as many as five times the normal proteins by proteolysis, a chemical reaction that breaks peptide number of muscle fibers for each motoneuron coming from bonds. Ubiquitin is a regulatory protein that basically labels the spinal cord. This decreases the fineness of control one which cells will be targeted for proteasomal degradation. has over the muscles but does allow the muscles to regain varying degrees of strength. Adjustment of Muscle Length. Another type of hyper- trophy occurs when muscles are stretched to greater than Rigor Mortis normal length. This causes new sarcomeres to be added Several hours after death, all the muscles of the body go into at the ends of the muscle fibers, where they attach to the a state of contracture called “rigor mortis”; that is, the mus- tendons. In fact, new sarcomeres can be added as rapidly cles contract and become rigid, even without action poten- tials. This rigidity results from loss of all the ATP, which is as several per minute in newly developing muscle, illus- required to cause separation of the cross-bridges from the trating the rapidity of this type of hypertrophy. actin filaments during the relaxation process. The muscles Conversely, when a muscle continually remains remain in rigor until the muscle proteins deteriorate about shortened to less than its normal length, sarcomeres at 15 to 25 hours later, which presumably results from autolysis the ends of the muscle fibers can actually disappear. It is caused by enzymes released from lysosomes. All these events by these processes that muscles are continually remod- occur more rapidly at higher temperatures. eled to have the appropriate length for proper muscle contraction. Bibliography Hyperplasia of Muscle Fibers. Under rare conditions Allen DG, Lamb GD, Westerblad H: Skeletal muscle fatigue: cellular mecha- of extreme muscle force generation, the actual number of nisms, Physiol Rev 88:287, 2008. muscle fibers has been observed to increase (but only by a Berchtold MW, Brinkmeier H, Muntener M: Calcium ion in skeletal muscle: few percentage points), in addition to the fiber hypertro- its crucial role for muscle function, plasticity, and disease, Physiol Rev phy process. This increase in fiber number is called fiber 80:1215, 2000. hyperplasia. When it does occur, the mechanism is linear Cheng H, Lederer WJ: Calcium sparks, Physiol Rev 88:1491, 2008. Clanton TL, Levine S: Respiratory muscle fiber remodeling in chronic splitting of previously enlarged fibers. hyperinflation: dysfunction or adaptation? J Appl Physiol 107:324, 2009. Effects of Muscle Denervation. When a muscle Clausen T: Na+-K+ pump regulation and skeletal muscle contractility, loses its nerve supply, it no longer receives the contrac- Physiol Rev 83:1269, 2003. tile signals that are required to maintain normal mus- Dirksen RT: Checking your SOCCs and feet: the molecular mechanisms of Ca2+ entry in skeletal muscle, J Physiol 587:3139, 2009. cle size. Therefore, atrophy begins almost immediately. Fitts RH: The cross-bridge cycle and skeletal muscle fatigue, J Appl Physiol After about 2 months, degenerative changes also begin to 104:551, 2008. appear in the muscle fibers themselves. If the nerve sup- Glass DJ: Signalling pathways that mediate skeletal muscle hypertrophy ply to the muscle grows back rapidly, full return of func- and atrophy, Nat Cell Biol 5:87, 2003. tion can occur in as little as 3 months, but from that time Gordon AM, Regnier M, Homsher E: Skeletal and cardiac muscle contractile activation: tropomyosin “rocks and rolls”, News Physiol Sci 16:49, 2001. onward, the capability of functional return becomes less Gunning P, O’Neill G, Hardeman E: Tropomyosin-based regulation of the and less, with no further return of function after 1 to 2 actin cytoskeleton in time and space, Physiol Rev 88:1, 2008. years. Huxley AF, Gordon AM: Striation patterns in active and passive shortening In the final stage of denervation atrophy, most of the of muscle, Nature (Lond) 193:280, 1962. muscle fibers are destroyed and replaced by fibrous and Kjær M: Role of extracellular matrix in adaptation of tendon and skeletal muscle to mechanical loading, Physiol Rev 84:649, 2004. fatty tissue. The fibers that do remain are composed of Lynch GS, Ryall JG: Role of beta-adrenoceptor signaling in skeletal muscle: a long cell membrane with a lineup of muscle cell nuclei implications for muscle wasting and disease, Physiol Rev 88:729, 2008. but with few or no contractile properties and little or MacIntosh BR: Role of calcium sensitivity modulation in skeletal muscle no capability of regenerating myofibrils if a nerve does performance, News Physiol Sci 18:222, 2003. regrow. Phillips SM, Glover EI, Rennie MJ: Alterations of protein turnover underlying disuse atrophy in human skeletal muscle, J Appl Physiol 107:645, 2009. The fibrous tissue that replaces the muscle fibers dur- Powers SK, Jackson MJ: Exercise-induced oxidative stress: cellular mech- ing denervation atrophy also has a tendency to continue anisms and impact on muscle force production, Physiol Rev 88:1243, shortening for many months, which is called contracture. 2008. Therefore, one of the most important problems in the Sandri M: Signaling in muscle atrophy and hypertrophy, Physiology practice of physical therapy is to keep atrophying muscles (Bethesda) 160, 2008. Sieck GC, Regnier M: Plasticity and energetic demands of contraction in from developing debilitating and disfiguring contractures. skeletal and cardiac muscle, J Appl Physiol 90:1158, 2001. This is achieved by daily stretching of the muscles or use Treves S, Vukcevic M, Maj M, et al: Minor sarcoplasmic reticulum mem- of appliances that keep the muscles stretched during the brane components that modulate excitation-contraction coupling in atrophying process. striated muscles, J Physiol 587:3071, 2009. 82