Chapter 6 - Lipids.docx
Document Details
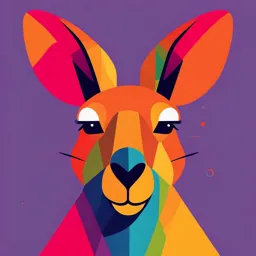
Uploaded by StableWombat
Simon Fraser University
Full Transcript
Chapter 6: Lipids Lipids are molecules that are partly or entirely hydrophobic, meaning that they do not dissolve readily in water. For instance, when an ounce of oil, which is full of lipids, is dropped into a glass of water, the two will naturally sepa- rate. The main lipids that we will cover in...
Chapter 6: Lipids Lipids are molecules that are partly or entirely hydrophobic, meaning that they do not dissolve readily in water. For instance, when an ounce of oil, which is full of lipids, is dropped into a glass of water, the two will naturally sepa- rate. The main lipids that we will cover in this chapter are triglycerides, fatty acids (or fats), sterols and phospholipids. Technically the fat-soluble vitamins A, D, E and K are also dietary lipids, but their roles will be discussed in the chapter on vitamins. By the end of this chapter, you will be able to: Differentiate between triglycerides, sterols and phospholipids. Describe fatty acids based on their length, degree of saturation and geometric organization. Outline how lipids are transported in the small intestine, lymphatic system and bloodstream. List the functions of lipids in the body. Describe how the consumption of different lipids affects health. Triglycerides are the main dietary lipid. They consist of a glycerol backbone with three fatty acids attached to it (Figure 6.1). Typically, when we talk about dietary fat, we are referring to these fatty acids. Fatty acids can be categorized according to their degree of saturation, their length and/or their geometric organization. Figure 6.1: Triglyceride structure. Fatty acids differ in their degree of saturation. Figure 6.2 shows both a saturated fatty acid as well as three unsat- urated fatty acids. The ends of both types of fats are the same. One end has a –CH3 group (represented by the end of the line). This is the fatty acid’s methyl, or omega end. The other end has a carboxylic acid group (–COOH). This is the acid end. Figure 6.2: Saturated, monounsaturated and polyunsaturated fatty acids. The main difference between saturated and unsaturated fatty acids is that unsaturated fatty acids have one or more double bonds in their chain. The presence of a double bond kinks the chain, so it is no longer a straight-chain fatty acid. A fundamental rule in nature is that structure dictates function. An apparently minor change like the addition of a double bond can make fatty acids behave very differently. We will explore this later in the chapter when we discuss the health effect of various lipids. Fatty acids with one double bond are called monounsaturated fatty acids (MUFAs) (Figure 6.2b). Unsaturated fat- ty acids with two or more double bonds are polyunsaturated fatty acids (PUFAs) (Figure 6.2c, d). Recall that double bonds result in kinks in the chain – the more double bonds, the more kinks. Figure 6.2 also shows two examples of omega fatty acids, specifically an omega-3 (Figure 6.2c) and an omega-6 (Figure 6.2d) fatty acid. The names refer to the location of the double bond in the chain. Recall that the methyl end is called the omega end. From this end, carbon atoms are counted until the first double bond is reached. If the first double bond is at the third carbon atom from the omega end, it is an omega-3 fatty acid. If found at the sixth carbon atom from the omega side, it is an omega-6 fatty acid. An omega-9 fatty acid has its fist double bond at the ninth position from the omega end (not shown). The human body cannot synthesize PUFAs with the double bond before the ninth position. Accordingly, omega-3 and omega-6 fatty acids are essential fatty acids, but omega-9 fatty acids are not. Two fatty acids are essential to humans: alpha-linolenic acid (omega-3) and linoleic acid (omega-6) (Figure 6.3). They cannot be synthesized by the human body and must be consumed from the diet. Interestingly, these were both previously known as vitamin F before they were found to be better classified as lipids as opposed to fat-soluble vitamins. Alpha-linolenic acid can be used to synthesize two other important omega-3 fatty acids, docosahexaenoic acid (DHA) and eicosapentaenoic acid (EPA) (Figure 6.3a). These omega-3 fatty acids are not found in many foods, so intake may be limited. Chia and flax seeds contain high amounts of alpha-linolenic acid, while fish, fish oil, beef and lamb pro- vide EPA and DHA. Figure 6.3: Essential fatty acids. The omega-6 fatty acid linoleic acid can be used to synthesize another essential omega-6 fatty acid, arachidonic acid (Figure 6.3b). Omega-6 fatty acids are common in our current food system; good sources include soybeans, corn and vegetable oils. Both omega-3 fatty acids and omega-6 fatty acids can be used to synthesize signalling molecules called eicosanoids. Eicosanoids derived from omega-3 fatty acids have anti-inflammatory properties, while those derived from omega-6 fatty acids have pro-inflammatory properties (Saini & Keum, 2018). Whether and how this affects health will be discussed later in the chapter. Another way we categorize fatty acids is by their length (Figure 6.4). Long-chain fatty acids have a chain of 14 or more carbon atoms (Figure 6.4a). Most fatty acids we consume have 18–22 carbon atoms. Medium-chain fatty acids, like those found in tropical oils, have between 6 and 12 carbon atoms in their length (Figure 6.4b). Figure 6.4: Fatty acid length. Short-chain fatty acids (SCFAs) are those that have less than 6 carbon atoms in their chain. The diet is a poor di- rect source of them. The majority of the body’s SCFAs are produced in the large intestine by bacteria (Figure 6.4c). Recall from Chapter 5 that bacteria in the large intestine ferment certain carbohydrates that the small intestine was not able to digest, producing SCFAs in the process. These then serve as the main energy source for the cells of the colon. Whatever is left over is then absorbed and supplies the body with SCFAs. The final way fatty acids are named is according to their geometric organization (Figure 6.5). Unsaturated fat- ty acids can be either cis- or trans-fatty acids. Figure 6.5a shows the fatty acid cis-oleic acid, an 18-carbon long-chain monounsaturated fatty acid. It has a kink in the chain at the double bond. Figure 6.5b shows trans-oleic acid. While the number of carbon, hydrogen and oxygen atoms is the same as the cis-oleic acid, the trans-oleic acid does not have a kink in the chain. Figure 6.5: Structure of cis- and trans-fatty acids. The main difference between cis- and trans-fatty acids is where the hydrogens lie in space around the double bond. Notice that in the cis configuration, the hydrogens are on the same side of the double bond. This creates a small force that pushes the rest of the chain away and produces the observed kink. In the trans fatty acid, the hydrogen atoms are on opposite sides of the double bond. This does not push the rest of the chain away and makes them resemble a saturated fatty acid (Figure 6.2a). Recall that structure dictates function. While chemically these two molecules are com- posed of the same atoms in the same order, they are different geometrically. This makes them behave differently in the body. The food industry converts cis-fatty acids into trans-fatty acids through a process called hydrogenation. This is done because trans-fatty acids are more stable and therefore have a longer shelf life. Food manufacturers want to reduce food waste and maximize sales potential, which is why hydrogenation became so popular. However, years of research have established significant concerns regarding these artificial trans fats in our food system. They are known to signifi- cantly increase the risk of cardiovascular disease (CVD) and other health issues (Mozaffarian et al., 2006). Accordingly, there is a push to eliminate trans fats from our food system. Another group of lipids is the sterol group. Sterols have a ring formation made up of a hydrocarbon chain (Figure 6.6). Though the sterol shown in Figure 6.6 looks very different to triglycerides, sterols are considered lipids because they are also hydrophobic. Sterols play a variety of structural and functional roles in the body. For instance, some sterols provide structure to the cell mem- brane, while other sterols can be used to form the hor- mones that facilitate body functions. In the diet, sterols can be consumed from both plant and animal products. Figure 6.6: The structure of cholesterol. The most common animal-derived sterol is cholesterol. Cholesterol is a crucial molecule with several key roles in the body. About a third of the human cell membrane is composed of cholesterol. Its presence in the outer layer of cells promotes membrane structure, while also helping to control what can enter and exit cells. Cholesterol is also a precursor for several important molecules in the body, including vitamin D, which regulates calcium balance, estrogen and testos- terone, which are important sex hormones, and cortisol, which is the main stress hormone. The liver and other body tissues can synthesize their own cholesterol, so it is not needed from the diet. It is therefore not considered an essential nutrient. Plant-derived sterols are also known as plant sterols or phytosterols. These sterols are similar to the animal-de- rived sterol cholesterol but have a slightly different molecular structure. Vegetable oils are the richest source of plant sterols. However, when oils are refined, such as through hydrogenation, their relative composition of plant sterols is lowered (Phillips et al., 2002). Plant sterols can also be found in nuts, seeds and fruits. Like animal-derived sterols, plant sterols form the precursors for a variety of hormones that help plants grow and develop. When humans consume plant sterols, they compete with cholesterol, which limits its absorption. Phospholipids are a critical component of the outer surface of every human cell – the cell membrane. The body can make phospholipids itself, so they are not essential from the diet. Figure 6.7 shows a phospholipid and a triglyceride. These two structures share many structural similarities. For instance, they both have fatty acids attached to a glycerol backbone. However, the triglyceride has three fatty acids, while the phospholipid has two fatty acids; in the place of the third fatty acid there is a phosphate group. This phosphate group gives phospholipids their name. Figure 6.7: Triglycerides and phospholipids share structural features. Recall that triglycerides are completely hydrophobic. Conversely, phospholipids are amphiphilic – meaning they have both a hydrophobic (water-hating) and hydrophilic (water-loving) end. The fatty acid groups are hydrophobic, while the phosphate group is hydrophilic. Their amphiphilic nature gives phospholipids their special properties and functions. When several phospholipids are immersed in water, they arrange themselves as seen in Figure 6.8 – with their phosphate heads facing the watery environment and their fatty acid tails facing each other. The membrane of each cell is composed of this phospholipid bilayer. This membrane is quite special as it allows the water content within the cell to be fully separated from the water content outside the cell. Only water and non-polar substances like fats can readily pass through this membrane. Most other molecules and ions are not able to pass through the bilayer unless there is a trans- porter. Recall the different ways that nutrients are absorbed from Chapter 3. Carbohydrates, amino acids, minerals and water-soluble vitamins require a protein transporter (and potentially energy) to be able to move from one side of the cell to the other. This is critical, as the body needs to tightly regulate what moves into and out of cells. Figure 6.8: The phospholipid bilayer. Phospholipids also have an important role in the food industry, as they help form emulsions. This concept is illustrated by comparing natural peanut butter and processed peanut butter. Natural peanut butter separates into a lower, protein-rich portion and an upper, lipid-rich portion. This is because protein is hydrophilic, while the lipid in the nut butter is hydrophobic. These two types of structures naturally separate into two solutions. On the other hand, pro- cessed peanut butter has phospholipid emulsifiers, which allow it to form a more uniform solution called an emulsion. This occurs because the hydrophobic ends of the phospholipids arrange themselves facing the lipids in the peanut butter, and the hydrophilic ends arrange themselves to face the protein-rich parts of the peanut butter. This allows the lipid-rich parts to mix with the protein-rich parts and form a more uniform solution that does not separate. Vitamins A, D, E and K are all technically lipids since they are hydrophobic. Unlike triglycerides, however, they do not provide energy to the body. Instead, they are responsible for several important physiological processes. In nutrition, we typically do not classify these as lipids and instead categorize them as fat-soluble vitamins. They will therefore be discussed in the Chapter 8. We will focus our discussion on the digestion of triglycerides, as they account for approximately 90% of dietary lipids. Triglycerides cannot be absorbed as ingested; they must first be broken down into fatty acids and their glycerol backbone. Figure 6.9 gives an overview of lipid digestion. Lipid digestion begins in the mouth with the release of lingual lipase. This enzyme removes a fatty acid from the triglyceride. The action of lingual lipase continues as we swallow the lipid-containing food into our esophagus. The stomach’s acidic environment stops the activity of this enzyme, but lip- id digestion continues here due to the secretion of gastric lipase by the stomach’s cells. Lingual and gastric lipase are particularly important for digesting small- and medium-chain fatty acids and act to begin lipid digestion. However, most lipid digestion occurs in the small intestine with the assistance of two important molecules. Bile emulsifies larger lipid structures into smaller ones, while pancreatic lipase further breaks down lipids into smaller components. If lipids are not digested by lipases, they cannot be digested, nor absorbed (Box 6.1). Bile is made in the liver and stored in the gallbladder. When lipids enter the small intestine, bile is secreted into the duodenum to begin emulsifying these fats. Bile has an amphiphilic structure, making it an excellent emulsifier. Recall from Chapter 3 that bile salts, the main components of bile, arrange themselves with their hydrophobic tails pointing towards the lipid center and their hydrophilic heads facing the watery environment of the small intestine, forming a mi- celle. Sterols, fat-soluble vitamins and other hydrophobic structure can also be found within micelles. Further, this emul- sification of lipids allows large lipid globules to be divided into smaller, more uniformly distributed droplets. This process increases the surface area on which pancreatic lipase can act, making its work more efficient. Pancreatic lipase works on the triglycerides in the micelle to separate fatty acids. This prepares them for absorption. Box 6.1: Orlistat – a drug that blocks fat absorption. Orlistat is a drug that inhibits fat absorption in the stomach and small intestine. In Canada, it is available by prescription under the brand name Xenical. A lower-dose over-the-counter version called Alli is also available. They are both typically used as an obesity treatment. Orlistat binds to the active site of the enzymes gastric and pancreatic lipase, restricting the break down of triglycerides into free fatty acids. Since triglycerides cannot be absorbed without being digested, this also blocks fat absorption. In general, fat absorption decreases by 30% with its use (Henness & Perry, 2006). Since less fat is absorbed, there are less calories from fat absorbed and an energy deficit that promotes weight reduction is favoured. Indeed, several randomized control trials have shown a modest decrease in fat mass and body weight with orlistat intake compared to placebo (Golay et al., 2005; Ozcelik et al., 2004; Tiikkainen et al., 2004). A 2018 review of 27 randomized control trials also found a mild but significant decrease in blood pressure with orlistat use (Sahebkar et al., 2018). Though mostly well tolerat- ed, those who take the drug and consume a diet higher in fat may be more likely to experience gastrointes- tinal symptoms such as oily stools, flatulence with discharge, increased defecation and fecal incontinence (Henness & Perry, 2006). These symptoms can be very uncomfortable and even embarrassing for some. Accordingly, a patient may choose to minimize lipid intake while taking orlistat or seek alternative weight management options. Figure 6.10 outlines lipid absorption. At the absorptive villus of the small intestine, the micelle breaks down. Its digested fat components pass through the small intestine-facing membranes of the intestinal cells. Since the cell mem- brane is composed of phospholipids, lipids can easily pass through the spaces between them through passive diffusion. Once inside the small intestine cells, long-chain fatty acids bond with a glycerol molecule to reassemble into triglycerides. As these and other lipid components move out of the small intestine cell, they become wrapped in a membrane mainly composed of phospholipids, but also containing protein and cholesterol. This new structure is called a lipoprotein. This specific lipoprotein is called a chylomicron. Figure 6.10: Lipid absorption. Transporting lipids requires a series of lipoprotein molecules. The word lipoprotein combines the words lipid and protein. Indeed, the outer shell of a lipoprotein is composed of phospholipids with embedded large proteins (Figure 6.11). Since most lipids are hydropho- bic, the hydrophilic shell provides a structure that allows them to dissolve and move readily through watery environments such as the blood and lymph. The main purpose of a lipoprotein is to carry lipids around the body. Accordingly, lipids that are being transported, including tri- glycerides, cholesterol and fat-soluble vitamins, are found within its core. Lipoproteins are mostly made of lipids, which have low density. The more lipids there are in a lipoprotein, the less dense they are. At the tissues, the lipids found within the core can be delivered where they are needed or to adipose tissue where they can be stored. As lipoproteins lose triglycerides, their density increases. Therefore, very low-density lipoproteins (VLDL) have the most triglycerides, while high-density lipoproteins have the least triglycerides. Figure 6.12 summarizes how lipoproteins transport lipids around the body. Chylomicrons transport lipids be- tween the small intestine and the liver. Unlike most other absorbed dietary components, these chylomicrons and the lipids they contain are too large to enter the blood vessels of the villi. Instead, they are absorbed into lacteals – the one-way lymphatic vessels found within the villi (Figure 6.10). The exception here are medium-chain fatty acids, which do drain directly into the blood vessels of the villi. Once chylomicrons are depostied into the bloodstream from the lymphat- ic vessels, they circulate through the body, dropping off lipids to body tissues as needed. Blood vessels near lipid-desiring tissues, such as muscle and fat tissue, express an enzyme called lipoprotein lipase (LPL) on their surface. LPL promotes the uptake of lipoprotein contents, particularly fatty acids, into cells. The remaining chylomicron structure and its compo- nents end up at the liver, where the chylomicron breaks down into its components. Figure 6.12: Lipoproteins transport and deliver lipids. The liver is not a primary storage location for lipids, so it constructs another lipoprotein to help send off lipids to the rest of the body for use or storage. The liver makes a lipoprotein called very low-density lipoprotein (VLDL) that is low in density because it is mainly composed of triglycerides. VLDL is the main triglyceride delivery system from the liver. Again, cells expressing LPL can uptake the triglyceride components, removing them from VLDL. As these lipids leave, the entire lipoprotein becomes denser, and it is converted into an intermediate density lipoprotein and eventually low-density lipoprotein (LDL). LDL has far fewer relative triglycerides compared to VLDL and is proportionally higher in cholesterol. LDL is thus the body’s primary cholesterol-delivery structure, delivering this sterol where it is needed. As we will learn later, LDL can potentially become quite reactive and even build up in artery walls, promoting cardiovascular issues. It is thus often referred to as bad cholesterol. LDL is not intrinsically bad, but chronically high levels may increase the risk of CVD. In addition to synthesizing VLDL, the liver also makes high-density lipoprotein (HDL). HDL has a higher protein content and a lower lipid content, making it higher in density. HDL can pick up cholesterol as it travels through the blood- stream and return it to the liver, thus lowering the amount of cholesterol circulating in the blood. Higher levels of HDL are associated with a lower risk of CVD, which is why it is often referred to as good cholesterol. Lipid Functions Energy Provision Lipids are one of the three ener- gy-yielding nutrients, affording 9 kcal of energy per gram. The main lipid we use for energy is the triglyceride. Figure 6.13 shows the lipid metabolism process that was covered in Chapter 3. Recall that the glycerol backbone can be used to synthe- size glucose, which can then be metab- olized into acetyl CoA. Fatty acids also form acetyl CoA by breaking down two carbon atoms at a time through beta-ox- idation. Figure 6.13: Lipid metabolism. There are two potential fates for the acetyl CoA produced through the metabolism of triglycerides: If an individual consumes a diet that contains sufficient carbohydrates (>~130 g/day), acetyl CoA enters the citric acid cycle, and cellular respiration proceeds as was first described in Chapter 3 (Figure 6.12a). This is because for acetyl CoA to enter the citric acid cycle, it must react with oxaloacetate. Adequate carbohydrate intake is required to maintain oxaloacetate levels. This is what is meant by the phrase, fat burns in a carbohydrate flame. On a very low-carbohydrate diet and during times of fasting, oxaloacetate levels are insufficient, and lipid-derived acetyl CoA is metabolized differently (Figure 6.12b). This alternative metabolism produces ketones, through ketogenesis. The body can adapt to preferentially use ketones instead of carbohydrates as a primary energy source. In the early stages of this adaptation, a person may experience flu-like symptoms. Later, the main symptoms are decreased appetite and an odour similar to nail polish remover on the breath (Kang et al., 2004; Yancy et al., 2004). When we consume more energy than the body requires, excess lipids will be stored in our fat cells for later use (Figure 6.14). Together, our fat cells make up our adipose tissue, or fat tissue. Chylomicrons deliver excess lipids from the small intestine to adipose tissue. Conversely, VLDL is the primary system that delivers lipids from the liver to adipose tissue. In an energy positive state, excess dietary carbohydrates and proteins that are not otherwise required will be con- verted into lipids and then also stored in our fat cells. Figure 6.14: Our energy status determines whether lipids are stored or metabolized. When we are in an energy deficit, our bodies break down lipids from adipose tissue. These can then be metabo- lized to capture energy as ATP in order to fuel the body’s needs. This system helps protect us from times when food and energy are scarce. This was a lot more important during hunter-gatherer days when the availability of the next meal was often unknown. Today, with an overabundance of food, energy storage is more likely to lead to obesity and its related complications. The membrane of a cell helps contain its contents, while also regulating what can pass into and out of that cell. Human cell membranes are primarily composed of phospholipids (Figure 6.8). These phospholipids are also found in the structure of key cellular organelle such as the nucleus, mitochondria and endoplasmic reticulum. We require dietary lipids to facilitate the absorption of vitamins A, D, E and K – the fat-soluble vitamins. These vitamins are packaged, absorbed and transported along with other dietary lipids within micelles (small intestine) and lipoproteins (blood). If we do not have an immediate need for these vitamins, they are stored within our fat cells for later use. Thus, in addition to energy storage, our fat cells are also responsible for fat-soluble vitamin storage. Individuals who do not consume enough fat or have issues with fat absorption are at risk for fat-soluble vitamin deficiencies. Lipids form the precursors for several key molecules in the body. Cholesterol is the precursor for a wide-range of important signalling molecules, including steroid hormones, bile salts, vitamin-D, and the sex hormones estrogen and testosterone. Omega-3 and omega-6 fatty acids can be used to synthesize a wide range of eicosanoids. These signalling mole- cules have diverse roles in the body, including effects on blood vessels, blood clotting and inflammation. Essential fatty acids can also be used to synthesize endocannabinoids. These signalling molecules have effects throughout the body, but their receptors are highly expressed in the brain. They have potential effects on cognitive function, appetite, mood and memory. Whether lipids contribute to our health or not depends on several factors. This includes which types of lipids we regularly consume, how much of them we consume, the source of these lipids and our health. This section gives an overview of the health-related issues pertaining to lipids. Specifically, it covers which lipids, when consumed in higher or lower amounts, influence health and disease. One of the cardiovascular system’s main roles is to carry oxygen and nutrient-rich blood to tissues so they can fuel themselves. It also removes waste products, so they do not build up. CVD compromises this delivery system and can have profound effects on the health and wellbeing of individuals. In Canada, more than a quarter of all deaths are due to CVD (Government of Canada, 2018), with the majority of these deaths being due to heart attacks or stroke. Heart attacks and most strokes occur due, in part, to fatty materials building up in artery walls, narrowing the arteries and restricting blood flow. This artery narrowing is called atherosclerosis (Figure 6.15). A blood clot can get stuck in a narrowed artery, stopping blood flow altogether. This leads to the death of tissue evidenced in the heart and brain that is evidenced in a heart attack or stroke, respectively. Figure 6.15: Atherosclerosis. There are risk factors for atherosclerosis that we have no control over, such as family history and age. However, the risk for this disease can be greatly reduced by modifying specific lifestyle factors. It is well established that not smok- ing, consistent exercise, maintaining a healthy weight and managing stress levels decrease the risk for CVD. Indeed, these are some of the key recommendations from the Heart and Stroke Foundation of Canada and the American Heart Asso- ciation (American Heart Association, n.d.; Heart and Stroke Foundation of Canada, n.d.). Diet also plays a critical role in determining the risk. Consuming a diet rich in whole foods with lots of vegetables and fruits is recommended (Heart and Stroke Foundation of Canada, n.d.). Also, certain lipid and non-lipid dietary factors can affect the risk. Trans fatty acids are well established to increase the ratio of LDL to HDL and increase the risk of CVD as well as all-cause mortality (Mozaffarian et al., 2006; Souza et al., 2015). This relationship has been known, without contention, for many years and prompted a 2006 review of the evidence to conclude that, “On a per-calorie basis, trans fats appear to increase the risk of [heart disease] more than any other macronutrient, conferring a substantially increased risk at low levels of consumption (1 to 3% of total energy intake)” (Mozaffarian et al., 2006). This statement and the large body of research on the topic have led to many position statements from top health organizations against the use of commercial trans fats. Canada has been ahead of the world in the effort against trans fat in the diet. In 2005, Canada became the first country to mandate that trans fat be listed on nutrition labels (Health Canada, 2006). In 2018, Health Canada took another major step and banned the use of partially hydrogenated oils, the main source of trans fat in the Canadian diet (Health Canada, 2018a). Diets that are higher in saturated fats are associated with higher levels of LDL. Decades of evidence has shown that higher levels of and more years of exposure to LDL are associated with a higher risk for CVD (Abdullah et al., 2018; Gao et al., 2017). Conversely, a reduction in LDL levels has been shown to reverse the development of atherosclerosis (Silverman et al., 2016; Storey et al., 2018). When saturated fat levels are reduced, levels of blood cholesterol also tend to decrease (Vartiainen et al., 2010). A 2015 systematic review of 15 randomized control trials further found that reduc- ing saturated fat intake reduced the risk of CVD (Hooper et al., 2015). The authors concluded that replacing these with polyunsaturated fats may be specifically beneficial. In 2017, the European Atherosclerosis Society Consensus Panel concluded that the link between high LDL and atherosclerosis was causal, stating, “Consistent evidence from numerous and multiple different types of clinical and ge- netic studies unequivocally establishes that LDL causes [atherosclerosis],” (Ference et al., 2017). This has been referred to as the lipid hypothesis, which suggests that one of the main drivers of CVD are the lipids found in our diet and blood. It is important to note that the lipid hypothesis and specifically the link between dietary saturated fat intake and CVD is not a clear-cut case. It is still a topic of much debate (Heileson, 2019; Malhotra et al., 2017). The issue is partly that most of the evidence that supports the link between saturated fat and CVD comes from epidemiological studies, which cannot establish a causal relationship. Also, a direct link between saturated fat and CVD mortality has not been es- tablished (Souza et al., 2015). This area of research will continue to evolve as we develop methods that allow us to better study the link between saturated fat, dietary lipids and cardiovascular health. Contrary to the CVD-promoting effects of saturated fats, PUFAs may afford protection. Several studies have found that when saturated fats in the diet were replaced with PUFAs, LDL levels and the risk of CVD decreased (Hooper et al., 2015; Li et al., 2015; Mozaffarian et al., 2010). To minimize the amount of saturated fat in our diets but also include more PUFAs, a sound option is to consume a whole foods-based diet that is rich in plants. Plants, such as nuts, seeds and vegetable oils, as well as fatty fish like salmon and trout tend to be higher in PUFAs. The 2019 Canadian food guide recommends replacing saturated fats with more sources of so-called healthy fats such as PUFAs (Health Canada, 2018c). Following this advice will not only reduce the amount of saturated and trans fat, but will also afford the many cardiovascular benefits of whole foods and plants (Kim et al., 2019; Srour et al., 2019). Recall that the essential fatty acids can be used to synthesize hormone-like molecules called eicosanoids. The effects of eicosanoids depend on whether they were derived from omega-3 or omega-6 fatty acids. Eicosanoids derived from omega-3 fatty acids have been touted for their cardioprotective benefits such as decreasing inflammation, blood cholesterol, vasoconstriction and blood clotting. However, scientists are still debating whether supplementing omega-3 fatty acids promotes a decreased risk of CVD. A systematic review of 86 randomized control trials found little or no effect of increasing EPA and DHA levels on reducing cardiovascular events (Abdelhamid et al., 2020). Conversely, a 2019 review of 13 randomized control trials found a slight decrease in heart attacks and cardio- vascular deaths with omega-3 fatty acid supplementation (Hu Yang et al., 2019). Their ability to independently decrease the risk of CVD is still a topic of debate and research, though they are not believed to increase the risk. Thus, replacing saturated fats with these PUFAs by eating more fish, fish oil and nuts instead of fattier red meat may be indicated. Eicosanoids derived from omega-6 fatty acids have been thought for some time to increase the risk of CVD due to their potential to increase inflammation and blood clotting. However, results of randomized control trials have been mixed, either showing a reduction in CVD risk or no effect (Harris & Shearer, 2014). A 2018 systematic review of 19 ran- domized control trials found that increased levels of omega-6 fatty acids did not increase the risk of CVD and was further found to have a small effect on lowering chance of a heart attack (Hooper et al., 2018). They also found that increasing omega-6 fatty acids is linked with a decrease in blood cholesterol. In addition to their effects on the cardiovascular system, there has also been extensive study into the effects of omega-3 fatty acids on healthy brain aging (Dyall, 2015; Swanson et al., 2012). DHA is particularly important in the brain and contributes to the structure and function of neuron membranes as well as neuron growth. Their anti-inflammato- ry effects may also contribute to brain health. A systematic review of 25 randomized control trials found that omega-3 supplementation is associated with improvements in the blood biomarkers that are linked with healthy brain aging (Derbyshire, 2018). Accordingly, this fatty acid’s potential to reduce the risk of Alzheimer’s disease is the topic of much research. Systematic reviews of the evidence have found that DHA levels correlate with delaying Alzheimer’s onset (Ajith, 2018; Canhada et al., 2018), but their full effects are still being explored. Cholesterol is found in animal products such as red meat, shellfish and eggs (Box 6.2). It is logical to think that to reduce LDL cholesterol, less dietary cholesterol must be consumed, but that is not how it works. We do not eat LDL; the liver makes it from available lipids. For most people, dietary cholesterol has a minimal effect on increasing LDL and cardiovascular risk (Fernandez, 2012; Soliman, 2018). This is because the body seems to produce less cholesterol when it is consumed form the diet, thus maintaining cholesterol levels despite dietary intake (Jones et al., 1996). This is not true for cholesterol hyper-responders (Katan et al., 1986). In about 25–30% of the population, cholesterol consumption does lead to increases in LDL. This is believed to be due to genetic differences in these individuals (Clifton & Abbey, 1997). Since most of us do not experience a rise in LDL and/or CVD risk due to dietary cholesterol, Health Canada does not state an explicit upper limit for cholesterol (McDonald, 2004). What is recommended is that most of our food choic- es come from plant products, with a reduction in animal products (Health Canada, 2018b). This not only decreases the amount of cholesterol in the diet, but it also reduces saturated fat consumption while raising the amount of unsaturated fats. Box 6.2: Eggs and cardiovascular health. Eggs are very nutrient dense, having a high amount of protein, fat, vitamins and minerals for about 75 kcal per egg. They are not only eaten on their own, but are also important in baking because the phospho- lipids in egg yolks make excellent emulsifiers. In addition to these properties, some eggs are also a good source of omega-3 fatty acids. Omega-3 fatty acids are not added to the eggs, as typically happens in food fortification. Instead, when chickens are fed flaxseeds, they convert the alpha-linolenic acid into DHA, and the both of these omega-3 fatty acids are incorporated into the egg yolk, making these eggs even more nutrient dense. But what about the cholesterol in eggs? Indeed, each egg contains about 225 mg of cholesterol. How- ever, for most of us, dietary cholesterol is not associated with CVD risk. Furthermore, in a meta-analysis that contained 22 epidemiological studies, it was found that egg consumption did not increase CVD risk or mortality (Shin et al., 2013). However, they did find a higher risk for diabetes in those who consumed more eggs. In a 2017 review, it was concluded that consuming three eggs per day for three months did not in- crease CVD risk in people with an elevated risk and that consuming cholesterol-free eggs did not decrease CVD risk compared to whole eggs (Clayton et al., 2017). The authors further suggested that the carotenoids (Vitamin A) found in eggs might have a role in reducing inflammation, which is protective for CVD. Taken together, in moderation, eggs can be included as part of a healthy diet. Nuts are high in fatty acids as well as plant sterols (Box 6.3). Plant sterols, also known as phytosterols are similar to animal-derived cholesterol, but are found in plants such as nuts, fruits and seeds. They are known to reduce low den- sity lipoprotein levels by competing with cholesterol for absorption and altering cholesterol metabolism (Calpe-Berdiel et al., 2009; Katan et al., 2003). They have accordingly been studied for their potential cardioprotective effects, but the evidence is murky at best. Some studies have actually found a slight increase in CVD disease risk with higher phytosterol levels (Assmann et al., 2006; Rajaratnam et al., 2000; Sudhop et al., 2002). However, a systematic review and meta-anal- ysis that evaluated 17 epidemiological studies found no association between plant sterol levels in the blood and CVD risk (Genser et al., 2012). Health Canada’s Food Directorate has approved the fortification of certain foods with plant sterols, including mayonnaise, margarine and salad dressings (Health Canada, 2010) and the Heart and Stroke Foundation of Canada recommends consuming 2 g of plant sterols per day to reduce LDL levels (Heart and Stroke Foundation of Canada, 2018). While plant sterols do lead to lowered LDL, whether that translates to lower CVD risk has not been established. Also, since some evidence points to a slight increased risk, plant sterol recommendations have been called in to question and more research is recommended (Köhler et al., 2017; Vergès & Fumeron, 2015). Box 6.4: An Indigenous lens: “Nuts are like the pan fish of the forest.” One of the best non-animal sources of lipids are nuts. Macadamia nuts, pine nuts and pecans are especially high in fat, having around 70 g of fat per 100 g serving. In English, the term pecan is used to refer to a type of nut. However, this word is derived from the word Pigan, which in certain Indigenous languages refers to all nuts. In the book, Braiding Sweetgrass (Kimmerer, 2013), Robin Wall Kim- merer, a botanist and member of the Citizen Potawatomi Nation, tells the story of how ancestors knew that these nuts were highly nutritious: The Boys may have come home fishless, but they brought back nearly as much protein as if they’d had a stringer of catfish. Nuts are like the pan fish of the forest, full of protein and especially fat – “poor man’s meat,” and they were poor. Today we eat them daintily, shelled and toasted, but in the old times they’d boil them up in a por- ridge. The fat floated to the top like a chicken soup and they skimmed it and stored it as nut butter: good winter food. High in calories and vitamins – everything you need to sustain life. After all, that’s the whole point of nuts: to provide the embryo with all that is needed to start a new life. (p. 13) Her story continues by honouring how important trees were for pro- viding this “poor man’s meat” and how important it is to live in harmony with them: Living by the precepts of the Honourable Harvest – to take only what is given, to use it well, to be grateful for the gift and to reciprocate the gifts is easy in a pecan grove. We reciprocate the gifts by taking care of the grove, protecting it from harm, planting seeds so that new groves will shade the prairie and feed the squirrels. (p. 20–21) These reflections illustrate both the nutritional knowledge and respect for nature found in many Indigenous cultures. To summarize, the effect lipids have on cardiovascular health depends on the type of lipid (Table 6.1). However, more research is still needed to fully understand how these different lipids affect the risk for CVD. Table 6.1: The effect of various lipids on cardiovascular health. While it was believed for some time that eating fat makes us fat. This is not exactly true and is an oversimplifica- tion of energy balance. Body fat and dietary fat are not the same thing and no single nutrient causes obesity. While lipids offer more calories per gram than protein and carbohydrates, it is the total amount of energy consumed from all sources that determines whether body fat is gained, not the energy source. Consuming too many calories from any energy-yield- ing nutrient can lead to extra fat storage on the body, but only if this consumption exceeds the body’s energy needs. We will explore the complex causes of obesity as well as its prevention and management in Chapter 10. Lipids are hydrophobic nutrients. They include triglycerides, sterols and phospholipids. Since lipids are hydropho- bic, they require transporters to move them around the small intestine and blood. Once at the tissues, they can be used for energy, stored for future energy or used to make certain lipid-derived molecules. In addition to their role in energy provision, lipids may also affect our cardiovascular health. Their effects depend on the specific type of lipid consumed. Lipids are energy-yielding nutrients that are hydrophobic. Triglycerides, fatty acids, sterols and phospholipids are all lipids, but the main dietary source of lipids are triglycerides. Triglycerides have three fatty acids attached to a glycerol backbone. These fatty acids vary based on their length, degree of saturation and geometry. Lipids do not dissolve in water, so they need transporters in order to be carried in the small intes- tine, the lymph and the blood. In the blood, lipoproteins carry lipids around the body, dropping off lipids as needed. Chylomicrons and very low-density lipoproteins primarily deliver fatty acids, while low-density lipoproteins primar- ily deliver cholesterol to body cells. Conversely, high density lipoprotein removes cholesterol from the blood. Lipids have several functions in the body, including energy provision, energy storage, delivery and storage of fat-soluble vitamins, cell membrane structure and signalling. Diets that are higher in trans fat are associated with a higher risk of CVD. These are mostly found in processed foods. High saturated fat intake is associated with increases in LDL. Elevated LDL is associated with a high- er CVD risk. However, it is not fully established as to whether saturated fat intake directly increases cardiovascular mortality. Consuming more unsaturated sources of fat from plant products compared to saturated fat sourc- es from animal products is recommended for decreasing CVD risk. Essential fatty acids are PUFAs that have a double bond at carbon position 3 or 6. Omega-3 and omega-6 fatty acids can be used to synthesize signalling molecules called eicosanoids, which have various cardiovascular effects. Omega-3 fatty acids are generally thought to lower the risk of CVD, but this relationship is still a topic of debate. The omega-3 fatty acid DHA is implicated in healthy brain aging. 181 The AMDR recommends that 20–35% of calories come from lipids. The AMDR for children 1–3 year of age is higher: 30–40% of calories from lipids. Consume most lipids from whole sources, including unprocessed nuts, seeds, plants, plant oils and animal products such as fish and eggs. Ideally, eliminate artificial trans fat from the diet. This can be done by avoiding or minimizing the consumption of processed food. Consume more foods that have unsaturated fat compared to foods higher in saturated fat. This can be achieved by consuming more plants than animals. Consume fish, fish oils, nuts, vegetable oils and ground flaxseed to get adequate omega-3 and omega-6 fatty acids, the essential fatty acids. Abdelhamid, A., Brown, T., Brainard, J., Biswas, P., Thorpe, G., Moore, H., Deane, K., Summerbell, C., Worthington, H., Song, F., & Hooper, L. (2020). Omega-3 intake for cardiovascular disease. Cochrane. https://doi.org/10.1002/14651858. CD003177.pub5 Abdullah Shuaib M., Defina Laura F., Leonard David, Barlow Carolyn E., Radford Nina B., Willis Benjamin L., Rohat- gi Anand, McGuire Darren K., de Lemos James A., Grundy Scott M., Berry Jarett D., & Khera Amit. (2018). Long-Term Association of Low-Density Lipoprotein Cholesterol With Cardiovascular Mortality in Individuals at Low 10-Year Risk of Atherosclerotic Cardiovascular Disease. Circulation, 138(21), 2315–2325. https://doi.org/10.1161/CIRCULATIONA- HA.118.034273 Ajith, T. A. (2018). A Recent Update on the Effects of Omega-3 Fatty Acids in Alzheimer’s Disease. Current Clinical Phar- macology, 13(4), 252–260. https://doi.org/10.2174/1574884713666180807145648 American Heart Association. (n.d.). Www.Heart.Org. https://www.heart.org/en Assmann, G., Cullen, P., Erbey, J., Ramey, D. R., Kannenberg, F., & Schulte, H. (2006). Plasma sitosterol elevations are as- sociated with an increased incidence of coronary events in men: Results of a nested case-control analysis of the Prospec- tive Cardiovascular Münster (PROCAM) study. Nutrition, Metabolism, and Cardiovascular Diseases: NMCD, 16(1), 13–21. https://doi.org/10.1016/j.numecd.2005.04.001 Calpe-Berdiel, L., Escolà-Gil, J. C., & Blanco-Vaca, F. (2009). New insights into the molecular actions of plant sterols and stanols in cholesterol metabolism. Atherosclerosis, 203(1), 18–31. https://doi.org/10.1016/j.atherosclerosis.2008.06.026 Canhada, S., Castro, K., Perry, I. S., & Luft, V. C. (2018). Omega-3 fatty acids’ supplementation in Alzheimer’s disease: A systematic review. Nutritional Neuroscience, 21(8), 529–538. https://doi.org/10.1080/1028415X.2017.1321813 Clayton, Z. S., Fusco, E., & Kern, M. (2017). Egg consumption and heart health: A review. Nutrition, 37, 79–85. https://doi. org/10.1016/j.nut.2016.12.014 Clifton, P. M., & Abbey, M. (1997). Genetic control of response to dietary fat and cholesterol. World Review of Nutrition and Dietetics, 80, 1–14. https://doi.org/10.1159/000059579 Derbyshire, E. (2018). Brain Health across the Lifespan: A Systematic Review on the Role of Omega-3 Fatty Acid Supple- ments. Nutrients, 10(8). https://doi.org/10.3390/nu10081094 Dyall, S. C. (2015). Long-chain omega-3 fatty acids and the brain: A review of the independent and shared effects of EPA, DPA and DHA. Frontiers in Aging Neuroscience, 7. https://doi.org/10.3389/fnagi.2015.00052 Ference, B. A., Ginsberg, H. N., Graham, I., Ray, K. K., Packard, C. J., Bruckert, E., Hegele, R. A., Krauss, R. M., Raal, F. J., Schunkert, H., Watts, G. F., Borén, J., Fazio, S., Horton, J. D., Masana, L., Nicholls, S. J., Nordestgaard, B. G., van de Slu- is, B., Taskinen, M.-R., Catapano, A. L. (2017). Low-density lipoproteins cause atherosclerotic cardiovascular disease. 1. Evidence from genetic, epidemiologic, and clinical studies. A consensus statement from the European Atherosclerosis Society Consensus Panel. European Heart Journal, 38(32), 2459–2472. https://doi.org/10.1093/eurheartj/ehx144 Fernandez, M. L. (2012). Rethinking dietary cholesterol. Current Opinion in Clinical Nutrition and Metabolic Care, 15(2), 117–121. https://doi.org/10.1097/MCO.0b013e32834d2259 Gao, S., Zhao, D., Wang, M., Zhao, F., Han, X., Qi, Y., & Liu, J. (2017). Association Between Circulating Oxidized LDL and Atherosclerotic Cardiovascular Disease: A Meta-analysis of Observational Studies. Canadian Journal of Cardiology, 33(12), 1624–1632. https://doi.org/10.1016/j.cjca.2017.07.015 Genser, B., Silbernagel, G., De Backer, G., Bruckert, E., Carmena, R., Chapman, M. J., Deanfield, J., Descamps, O. S., Rietzschel, E. R., Dias, K. C., & März, W. (2012). Plant sterols and cardiovascular disease: A systematic review and me- ta-analysis. European Heart Journal, 33(4), 444–451. https://doi.org/10.1093/eurheartj/ehr441 Golay, A., Laurent-Jaccard, A., Habicht, F., Gachoud, J.-P., Chabloz, M., Kammer, A., & Schutz, Y. (2005). Effect of orlistat in obese patients with binge eating disorder. Obesity Research, 13(10), 1701–1708. https://doi.org/10.1038/oby.2005.208 Government of Canada, S. C. (2018, May 10). Deaths, by cause, Chapter IX: Diseases of the circulatory system (I00 to I99). https://www150.statcan.gc.ca/t1/tbl1/en/tv.action?pid=1310014701 Harris William S., & Shearer Gregory C. (2014). Omega-6 Fatty Acids and Cardiovascular Disease. Circulation, 130(18), 1562–1564. https://doi.org/10.1161/CIRCULATIONAHA.114.012534 Health Canada. (2006, June 1). ARCHIVED - TRANSforming the Food Supply [Transparency - other]. Aem. https://www. canada.ca/en/health-canada/services/nutrients/fats/task-force-trans-fat/transforming-food-supply-report.html Health Canada. (2018a, September 17). Canadian Ban on Trans Fats Comes into Force Today [News releases]. Gcnws. https://www.canada.ca/en/health-canada/news/2018/09/canadian-ban-on-trans-fats-comes-into-force-today.html Health Canada. (2018b, October 4). Welcome to Canada’s food guide. https://food-guide.canada.ca/en/, https://food- guide.canada.ca/ Health Canada. (2018c, October 10). Choosing foods with healthy fats. https://food-guide.canada.ca/en/healthy-eat- ing-recommendations/make-it-a-habit-to-eat-vegetables-fruit-whole-grains-and-protein-foods/choosing-foods-with- healthy-fats/, https://food-guide.canada.ca/healthy-eating-recommendations/make-it-a-habit-to-eat-vegetables-fruit- whole-grains-and-protein-foods/choosing-foods-with-healthy-fats Health Canada, H. (2010, May 26). Plant Sterols (Phytosterols) in Foods [Assessments]. Aem. https://www.canada.ca/en/ health-canada/services/food-nutrition/food-labelling/health-claims/assessments/plant-sterols-phytosterols-foods-nutri- tion-health-claims-food-labelling.html Heart and Stroke Foundation of Canada. (n.d.). Get healthy. Heart and Stroke Foundation of Canada. Retrieved February 10, 2020, from https://www.heartandstroke.ca/en/get-healthy/ Heart and Stroke Foundation of Canada. (2018). Managing cholesterol. Heart and Stroke Foundation of Canada. https:// www.heartandstroke.ca/en/heart/risk-and-prevention/condition-risk-factors/high-cholesterol/ Heileson, J. L. (2019). Dietary saturated fat and heart disease: A narrative review. Nutrition Reviews. https://doi. org/10.1093/nutrit/nuz091 Henness, S., & Perry, C. M. (2006). Orlistat. Drugs, 66(12), 1625–1656. https://doi.org/10.2165/00003495-200666120- 00012 Hooper, L., Al-Khudairy, L., Abdelhamid, A. S., Rees, K., Brainard, J. S., Brown, T. J., Ajabnoor, S. M., O’Brien, A. T., Win- stanley, L. E., Donaldson, D. H., Song, F., & Deane, K. H. (2018). Omega-6 fats for the primary and secondary prevention of cardiovascular disease. Cochrane Database of Systematic Reviews, 7. https://doi.org/10.1002/14651858.CD011094.pub3 Hooper, L., Martin, N., Abdelhamid, A., & Smith, G. D. (2015). Reduction in saturated fat intake for cardiovascular disease. Cochrane Database of Systematic Reviews, 6. https://doi.org/10.1002/14651858.CD011737 Hu Yang, Hu Frank B., & Manson JoAnn E. (2019). Marine Omega-3 Supplementation and Cardiovascular Disease: An Updated Meta-Analysis of 13 Randomized Controlled Trials Involving 127 477 Participants. Journal of the American Heart Association, 8(19), e013543. https://doi.org/10.1161/JAHA.119.013543 Jones, P. J., Pappu, A. S., Hatcher, L., Li, Z. C., Illingworth, D. R., & Connor, W. E. (1996). Dietary cholesterol feeding sup- presses human cholesterol synthesis measured by deuterium incorporation and urinary mevalonic acid levels. Arterio- sclerosis, Thrombosis, and Vascular Biology, 16(10), 1222–1228. https://doi.org/10.1161/01.atv.16.10.1222 Kang, H. C., Chung, D. E., Kim, D. W., & Kim, H. D. (2004). Early- and Late-onset Complications of the Ketogenic Diet for Intractable Epilepsy. Epilepsia, 45(9), 1116–1123. https://doi.org/10.1111/j.0013-9580.2004.10004.x Katan, M. B., Beynen, A. C., de Vries, J. H., & Nobels, A. (1986). Existence of consistent hypo- and hyperresponders to dietary cholesterol in man. American Journal of Epidemiology, 123(2), 221–234. https://doi.org/10.1093/oxfordjournals. aje.a114231 Katan, Martijn B., Grundy, S. M., Jones, P., Law, M., Miettinen, T., Paoletti, R., & Stresa Workshop Participants. (2003). Efficacy and safety of plant stanols and sterols in the management of blood cholesterol levels. Mayo Clinic Proceedings, 78(8), 965–978. https://doi.org/10.4065/78.8.965 Kim, H., Caulfield, L. E., Garcia-Larsen, V., Steffen, L. M., Coresh, J., & Rebholz, C. M. (2019). Plant-Based Diets Are Asso- ciated With a Lower Risk of Incident Cardiovascular Disease, Cardiovascular Disease Mortality, and All-Cause Mortality in a General Population of Middle-Aged Adults. Journal of the American Heart Association, 8(16). https://doi.org/10.1161/ JAHA.119.012865 Kimmerer, R. W. (2013). Braiding Sweetgrass: Indigenous Wisdom, Scientific Knowledge and the Teachings of Plants. Milkweed Editions. Köhler, J., Teupser, D., Elsässer, A., & Weingärtner, O. (2017). Plant sterol enriched functional food and atherosclerosis. British Journal of Pharmacology, 174(11), 1281–1289. https://doi.org/10.1111/bph.13764 Li, Y., Hruby, A., Bernstein, A. M., Ley, S. H., Wang, D. D., Chiuve, S. E., Sampson, L., Rexrode, K. M., Rimm, E. B., Willett, W. C., & Hu, F. B. (2015). Saturated Fats Compared With Unsaturated Fats and Sources of Carbohydrates in Relation to Risk of Coronary Heart Disease: A Prospective Cohort Study. Journal of the American College of Cardiology, 66(14), 1538– 1548. https://doi.org/10.1016/j.jacc.2015.07.055 Malhotra, A., Redberg, R. F., & Meier, P. (2017). Saturated fat does not clog the arteries: Coronary heart disease is a chronic inflammatory condition, the risk of which can be effectively reduced from healthy lifestyle interventions. British Journal of Sports Medicine, 51(15), 1111–1112. https://doi.org/10.1136/bjsports-2016-097285 McDonald, B. E. (2004). The Canadian experience: Why Canada decided against an upper limit for cholesterol. Journal of the American College of Nutrition, 23(6 Suppl), 616S-620S. https://doi.org/10.1080/07315724.2004.10719432 Mozaffarian, D., Katan, M. B., Ascherio, A., Stampfer, M. J., & Willett, W. C. (2006). Trans Fatty Acids and Cardiovascular Disease. New England Journal of Medicine, 354(15), 1601–1613. https://doi.org/10.1056/NEJMra054035 Mozaffarian, D., Micha, R., & Wallace, S. (2010). Effects on Coronary Heart Disease of Increasing Polyunsaturated Fat in Place of Saturated Fat: A Systematic Review and Meta-Analysis of Randomized Controlled Trials. PLoS Medicine, 7(3), e1000252. https://doi.org/10.1371/journal.pmed.1000252 Ozcelik, O., Dogan, H., & Kelestimur, H. (2004). Effects of a weight-reduction program with orlistat on serum leptin levels in obese women: A 12-week, randomized, placebo-controlled study. Current Therapeutic Research, 65(2), 127–137. https://doi.org/10.1016/S0011-393X(04)90025-2 Phillips, K. M., Ruggio, D. M., Toivo, J. I., Swank, M. A., & Simpkins, A. H. (2002). Free and Esterified Sterol Composition of Edible Oils and Fats. Journal of Food Composition and Analysis, 15(2), 123–142. https://doi.org/10.1006/jfca.2001.1044 Rajaratnam, R. A., Gylling, H., & Miettinen, T. A. (2000). Independent association of serum squalene and noncholesterol sterols with coronary artery disease in postmenopausal women. Journal of the American College of Cardiology, 35(5), 1185–1191. https://doi.org/10.1016/s0735-1097(00)00527-1 Sahebkar, A., Simental-Mendía, L. E., Kovanen, P. T., Pedone, C., Simental-Mendía, M., & Cicero, A. F. G. (2018). Effects of orlistat on blood pressure: A systematic review and meta-analysis of 27 randomized controlled clinical trials. Journal of the American Society of Hypertension, 12(2), 80–96. https://doi.org/10.1016/j.jash.2017.12.002 Saini, R. K., & Keum, Y.-S. (2018). Omega-3 and omega-6 polyunsaturated fatty acids: Dietary sources, metabolism, and significance - A review. Life Sciences, 203, 255–267. https://doi.org/10.1016/j.lfs.2018.04.049 Shin, J. Y., Xun, P., Nakamura, Y., & He, K. (2013). Egg consumption in relation to risk of cardiovascular disease and dia- betes: A systematic review and meta-analysis. The American Journal of Clinical Nutrition, 98(1), 146–159. https://doi. org/10.3945/ajcn.112.051318 Silverman, M. G., Ference, B. A., Im, K., Wiviott, S. D., Giugliano, R. P., Grundy, S. M., Braunwald, E., & Sabatine, M. S. (2016). Association Between Lowering LDL-C and Cardiovascular Risk Reduction Among Different Therapeutic Interven- tions: A Systematic Review and Meta-analysis. JAMA, 316(12), 1289–1297. https://doi.org/10.1001/jama.2016.13985 Soliman, G. A. (2018). Dietary Cholesterol and the Lack of Evidence in Cardiovascular Disease. Nutrients, 10(6), 780. https://doi.org/10.3390/nu10060780 Souza, R. J. de, Mente, A., Maroleanu, A., Cozma, A. I., Ha, V., Kishibe, T., Uleryk, E., Budylowski, P., Schünemann, H., Bey- ene, J., & Anand, S. S. (2015). Intake of saturated and trans unsaturated fatty acids and risk of all cause mortality, cardio- vascular disease, and type 2 diabetes: Systematic review and meta-analysis of observational studies. BMJ, 351. https:// doi.org/10.1136/bmj.h3978 Srour, B., Fezeu, L. K., Kesse-Guyot, E., Allès, B., Méjean, C., Andrianasolo, R. M., Chazelas, E., Deschasaux, M., Hercberg, S., Galan, P., Monteiro, C. A., Julia, C., & Touvier, M. (2019). Ultra-processed food intake and risk of cardiovascular dis- ease: Prospective cohort study (NutriNet-Santé). BMJ (Clinical Research Ed.), 365, l1451. https://doi.org/10.1136/bmj. l1451 Storey, B. C., Staplin, N., Haynes, R., Reith, C., Emberson, J., Herrington, W. G., Wheeler, D. C., Walker, R., Fellström, B., Wanner, C., Landray, M. J., Baigent, C., Storey, B. C., Staplin, N., Haynes, R., Reith, C., Emberson, J., Herrington, W. G., Wheeler, D. C., … Collins, R. (2018). Lowering LDL cholesterol reduces cardiovascular risk independently of presence of inflammation. Kidney International, 93(4), 1000–1007. https://doi.org/10.1016/j.kint.2017.09.011 Sudhop, T., Gottwald, B. M., & von Bergmann, K. (2002). Serum plant sterols as a potential risk factor for coronary heart disease. Metabolism: Clinical and Experimental, 51(12), 1519–1521. https://doi.org/10.1053/meta.2002.36298 Swanson, D., Block, R., & Mousa, S. A. (2012). Omega-3 Fatty Acids EPA and DHA: Health Benefits Throughout Life1. Ad- vances in Nutrition, 3(1), 1–7. https://doi.org/10.3945/an.111.000893 Tiikkainen, M., Bergholm, R., Rissanen, A., Aro, A., Salminen, I., Tamminen, M., Teramo, K., & Yki-Järvinen, H. (2004). Effects of equal weight loss with orlistat and placebo on body fat and serum fatty acid composition and insulin resistance in obese women. The American Journal of Clinical Nutrition, 79(1), 22–30. https://doi.org/10.1093/ajcn/79.1.22 Vartiainen, E., Laatikainen, T., Peltonen, M., Juolevi, A., Mannisto, S., Sundvall, J., Jousilahti, P., Salomaa, V., Valsta, L., & Puska, P. (2010). Thirty-five-year trends in cardiovascular risk factors in Finland. International Journal of Epidemiology, 39(2), 504–518. https://doi.org/10.1093/ije/dyp330 Vergès, B., & Fumeron, F. (2015). Potential risks associated with increased plasma plant-sterol levels. Diabetes & Metabo- lism, 41(1), 76–81. https://doi.org/10.1016/j.diabet.2014.11.003 Yancy, W. S., Jr, Olsen, M. K., Guyton, J. R., Bakst, R. P., & Westman, E. C. (2004). A low-carbohydrate, ketogenic diet versus a low-fat diet to treat obesity and hyperlipidemia: A randomized, controlled trial. Annals Of Internal Medicine, 140(10), 769–777.