Chapter 5: The Working Cell PDF
Document Details
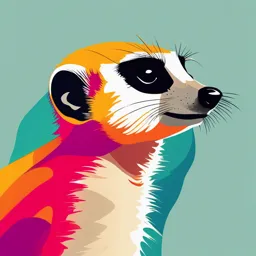
Uploaded by PeacefulBlueLaceAgate
Tags
Summary
This document describes the fluid mosaic model of cell membranes and the process of passive transport. It includes diagrams and explanations of how molecules move across cell membranes without requiring energy from the cell.
Full Transcript
**Chapter 5: The Working Cell** **5.1 Membranes are fluid mosaics of lipids and proteins with many functions** The fluid mosaic model describes a membrane\'s structure as diverse protein molecules suspended in a fluid phospholipid bilayer. This module explains the structure and function of the pla...
**Chapter 5: The Working Cell** **5.1 Membranes are fluid mosaics of lipids and proteins with many functions** The fluid mosaic model describes a membrane\'s structure as diverse protein molecules suspended in a fluid phospholipid bilayer. This module explains the structure and function of the plasma membrane, which encloses a living cell. The plasma membrane exhibits selective permeability, allowing some substances to cross more easily than others. Additionally, it regulates the exchange of materials and visualizes the activity in and across the membranes of two adjacent cells. A diagram of a cell membrane Description automatically generated Can you identify six different types of functions of proteins in a plasma membrane? Attachment to the cytoskeleton and ECM, signal reception and relay, enzymatic activity, cell-cell recognition, intercellular joining, and transport. **Terms to Know** **Phospholipids*-***A lipid made up of glycerol joined to two fatty acids and a phosphate group, giving the molecule two non-polar hydrophobic tails and a polar hydrophilic head. Phospholipids form bilayers that function as biological membranes. **Hydrophilic-**Water-loving"; pertaining to polar or charged molecules (or parts of molecules) that are soluble in water. **fluid mosaic model*-***The currently accepted model of cell membrane structure, depicting the membrane as a mosaic of protein molecules suspended in a fluid bilayer of phospholipid molecules. ------------------------------------------------------------------------------------------------------------------------------------------------------------------------------------------------------------------------------------------------------- -------------------------------------------------------------------------------------------------------------------------------------------------------------------------------------------------------------------------------------------------------------------------------------------------------------------------------------------------- --------------------------------------------------------------------------------------------------------------------------------------------------------------------------------------------------- **Hydrophobic**- "Water-fearing"; pertaining to nonpolar molecules (or parts of molecules) that do not dissolve in water. **lipid bilayer-**The lipid bilayer is a double layer of phospholipid molecules that forms the core structure of cell membranes. It has hydrophilic (water-loving) heads facing outward and hydrophobic (water-fearing) tails facing inward, creating a semi-permeable barrier that regulates the movement of substances in and out of the cell. **selective permeability-**A property of biological membranes that allows them to regulate the passage of substances across them. **5.3 Passive transport is diffusion across a membrane with no energy investment** Molecules possess thermal energy due to their constant motion. This motion leads to diffusion, where particles spread out into available space. Randomly moving molecules can diffuse through air, water, and even into and out of cells. The figures illustrate diffusion across a membrane. Figure 5.3A shows yellow dye in a solution separated from pure water by an artificial membrane with microscopic pores, making it permeable to the dye. Dye molecules move randomly, but there is a net movement from the side with higher concentration to the side with lower concentration, diffusing down the concentration gradient. Eventually, both sides will have equal dye concentrations, reaching dynamic equilibrium where molecules still move but with no net change in concentration. Figure 5.3B illustrates the important point that two or more substances diffuse independently of each other; that is, each diffuses down its own concentration gradient. Passive transport occurs when molecules diffuse across a cell membrane without the cell doing any work. Much of the traffic across membranes happens by diffusion. For instance, oxygen (O~2~) enters cells and carbon dioxide (CO~2~) exits cells through diffusion down concentration gradients. Oxygen (O~2~) and carbon dioxide (CO~2~) are small, nonpolar molecules that easily diffuse across the phospholipid bilayer of a membrane. Ions and polar molecules can also diffuse across the hydrophobic interior of a membrane if they are moving down their concentration gradients and have transport proteins to assist them. Why is diffusion across a membrane called passive transport? The cell does not expend energy to transport substances that are diffusing down their concentration gradients. **Terms to Know** **Diffusion***-*The random movement of particles that results in the net movement of a substance down its concentration gradient from a region where it is more concentrated to a region where it is less concentrated. **concentration gradient***-*A region along which the density of a chemical substance increases or decreases. Cells often maintain concentration gradients of ions across their membranes. When a concentration gradient exists, substances tend to move from where they are more concentrated to where they are less concentrated. **Hypotonic**-Referring to a solution that, when surrounding a cell, will cause the cell to take up water. ------------------------------------------------------------------------------------------------------------------------------------------------------------------------------------------------------------------------- ------------------------------------------------------------------------------------------------------------------------------------------------------------------------------------------------------------------------------------------------------------------------------------------------------------------------------------- -------------------------------------------------------------------------------------------------------------------- **facilitated diffusion-**The passage of a substance through a specific transport protein across a biological membrane down its concentration gradient. **Osmosis**-The diffusion of free water across a selectively permeable membrane. **Osmoregulation***-*The homeostatic maintenance of solute concentrations and water balance by a cell or organism. **passive transport**-The diffusion of a substance across a biological membrane, with no expenditure of energy. **Hypertonic-**Referring to a solution that, when surrounding a cell, will cause the cell to lose water.  **5.4 Osmosis is the diffusion of water across a membrane** Water is a crucial substance that crosses membranes by passive transport. The next module will explore the balance of water between a cell and its environment. Before that, it examines osmosis, the diffusion of water across a selectively permeable membrane, which allows some substances to cross more easily than others. The top of Figure 5.4 illustrates a membrane permeable to water but not to a solute (like glucose) separating two solutions with different solute concentrations. The solution on the right side of the U-shaped tube initially has a higher solute concentration than the left side. Water will cross the membrane until the solute concentrations are nearly equal on both sides, as shown in the U-tube on the right. The close-up view at the bottom of Figure 5.4 helps explain the molecular-level process of osmosis. Polar water molecules cluster around hydrophilic (water-loving) solute molecules. This clustering means there are fewer free water molecules on the right side of the U-tube, where the solute concentration is higher. On the left side, with fewer solute molecules, there are more free water molecules available to move. Water moves down its concentration gradient, from the side with more free water molecules (lower solute concentration) to the side with fewer free water molecules (higher solute concentration). This movement results in the difference in water levels seen in the U-tube. In simpler terms, water moves to where there are more solute molecules to try and equalize the concentration on both sides. This process continues until the concentrations are nearly equal, resulting in the difference in water levels you see in the U-tube. Predict the net water movement between two solutions---a 0.5% sucrose solution and a 2% sucrose solution---separated by a membrane not permeable to sucrose. Water will move from the 0.5% sucrose solution (lower solute concentration) to the 2% sucrose solution (higher solute concentration). **5.5 Water balance between cells and their surroundings is crucial to organisms** Biologists use specific vocabulary to describe water movement between a cell and its surroundings. Tonicity refers to a surrounding solution\'s ability to cause a cell to gain or lose water. It mainly depends on the concentration of solutes in the solution relative to the concentration of solutes inside the cell. Figure 5.5, on the facing page, illustrates the effects of placing animal and plant cells in solutions of different tonicities---solutions that have lower, equal, or higher concentrations of solutes compared to the cell. When an animal cell, like a red blood cell, is in an isotonic solution, its volume remains constant because the solute concentration inside the cell and in the solution are equal. This balance means the cell gains and loses water at the same rate. In the body, red blood cells are transported in isotonic plasma, and IV fluids must also be isotonic. Most animal body cells are bathed in isotonic extracellular fluid, and seawater is isotonic to many marine animals\' cells, like sea stars and crabs. In a hypotonic solution, \"hypo\" means less, so there is less solute outside the cell compared to inside. This causes water to move into the cell. In a hypertonic solution, \"hyper\" means more, so there is more solute outside the cell compared to inside, causing water to move out of the cell. This movement of water aims to balance the solute concentrations on both sides of the membrane. To survive in a hypotonic or hypertonic environment, an animal must prevent excessive water uptake or loss and regulate solute concentration in its body fluids. This control of water balance is called osmoregulation. For example, freshwater fish live in a hypotonic environment, so water enters their cells by osmosis, and their kidneys must constantly remove excess water. Water balance issues differ for plant, prokaryote, and fungi cells due to their cell walls. In a hypotonic environment, a plant cell becomes turgid (very firm), which is healthy for most plants. The cell wall exerts turgor pressure, preventing the cell from bursting. Non-woody plants rely on turgid cells for support. In an isotonic solution, there is no net water movement, making the cell flaccid (limp), and the plant may wilt. In a hypertonic environment, a plant cell loses water, shrivels, and its plasma membrane pulls away from the cell wall, a process called plasmolysis. This causes the plant to wilt and can be lethal. Bacteria and fungi cells also undergo plasmolysis in hypertonic environments. Concentrated salt solutions can preserve foods by causing plasmolysis in food-spoiling bacteria or fungi, leading to their death. Explain the function of the contractile vacuoles in a freshwater Paramecium (shown in Figure 4.11A) in terms of what you have just learned about water balance in cells. The pond water in which Paramecium lives is hypotonic to the cell. The contractile vacuoles expel the water that constantly enters the cell by osmosis. **5.6 Transport proteins can facilitate diffusion across membranes** Nonpolar molecules like O2 and CO2 can easily dissolve in the lipid bilayer of a membrane and diffuse through it. However, polar or charged substances need help to pass through the hydrophobic center of the membrane. Hydrophilic molecules and ions use specific transport proteins to move across the membrane in a process called facilitated diffusion. This type of passive transport does not require energy and is driven by the concentration gradient. Figure 5.6 illustrates two types of transport proteins. One type provides a channel for specific molecules or ions to pass through the membrane. The other type, called a carrier protein, binds to its passenger, changes shape, and releases the molecule on the other side. Both types of transport proteins facilitate the diffusion of substances across the membrane down their concentration gradient without requiring energy. Facilitated diffusion helps substances like sugars, amino acids, ions, and even water cross cell membranes. Although water molecules are small, their polar nature makes their diffusion through the membrane\'s hydrophobic interior slow. For many cells, this slow diffusion is sufficient. However, cells with higher water-permeability needs, such as plant cells, red blood cells, and kidney tubule cells, rely on a protein channel called aquaporin for rapid water diffusion. How do transport proteins contribute to a membrane's selective permeability? Transport proteins contribute to a membrane\'s selective permeability by allowing specific molecules and ions to pass through while blocking others. Channel shape and create passageways for certain substances, while carrier proteins bind to specific molecules, change shape, and release them on the other side. **5.8 Cells expend energy in the active transport of a solute** In active transport, a cell uses energy to move a solute against its concentration gradient, from an area of lower concentration to an area of higher concentration. The energy for this process is provided by ATP. Active transport helps cells maintain different internal concentrations of small molecules and ions compared to their surroundings. For instance, animal cells have higher potassium (K+) and lower sodium (Na+) concentrations inside than outside. The sodium-potassium pump, a transport protein, actively moves Na+ out and K+ into the cell, which is crucial for generating nerve signals. Figure 5.8 depicts an active transport system that pumps a solute out of the cell against its concentration gradient. The process starts with solute molecules attaching to specific binding sites on the transport protein on the cytoplasmic side of the plasma membrane. Using energy from ATP, the transport protein changes shape, releasing the solute on the other side of the membrane. The transport protein then returns to its original shape, ready for the next cycle. Here are the steps for the active transport system shown in Figure 5.8: 1. Solute molecules on the cytoplasmic side of the plasma membrane attach to specific binding sites on the transport protein. 2. With energy provided by ATP, the transport protein changes shape, releasing the solute on the other side of the membrane. 3. The transport protein returns to its original shape, ready for its next passengers. Cells actively transport Ca^2+^ out of the cell. Is calcium more concentrated inside or outside of the cell? Explain. Outside: Active transport moves calcium against its concentration gradient. **5.10 Cells transform energy and matter as they perform work** Cells actively transport substances across membranes and build those membranes and their embedded proteins. Cells act as miniature chemical factories where thousands of reactions occur within a microscopic space, involving the transformation of energy, a core theme in biology. Before delving into how cells work, the chapter introduces basic concepts of energy. **Forms of Energy** Energy is the capacity to cause change or do work. There are two basic forms of energy: kinetic energy and potential energy. Kinetic energy is the energy of motion, allowing moving objects to perform work by transferring motion to other matter. For example, your legs moving bicycle pedals. Thermal energy, a type of kinetic energy, is associated with the random movement of atoms or molecules and is called heat when transferred between objects. Light, another type of kinetic energy, can be harnessed for photosynthesis. Potential energy is the energy that matter possesses due to its location or structure. Examples include water behind a dam and a bicycle at the top of a hill. Molecules have potential energy because of the arrangement of electrons in their bonds. Chemical energy, a type of potential energy, can be released in chemical reactions and is used to power cellular work. **Energy Transformations** Thermodynamics is the study of energy transformations in a collection of matter. Scientists refer to the matter under study as the system and everything outside it as the surroundings. A system can be anything from an electric power plant to a single cell or the entire planet. Organisms are open systems, meaning they exchange both energy and matter with their surroundings. The first law of thermodynamics, or the law of energy conservation, states that energy in the universe is constant. It can be transferred and transformed but cannot be created or destroyed. For example, a power plant converts energy from coal into electricity, and a plant cell converts light energy into chemical energy. Both are energy transformers, not energy producers. During energy transfers or transformations, some energy becomes unavailable to do work as it is converted to thermal energy and released as heat. Entropy, a measure of disorder, increases with each energy conversion. According to the second law of thermodynamics, every energy conversion increases the entropy of the universe. Figure 5.10 illustrates the two laws of thermodynamics using a car and a cell. Both transform chemical energy into work, increasing entropy. In a car engine, oxygen mixes with gasoline in an explosive reaction, pushing pistons and moving the wheels. The waste products are mostly carbon dioxide and water. Only about 25% of the gasoline\'s energy converts to kinetic energy, with the rest lost as heat. Cells use oxygen in reactions that release energy from fuel molecules through a process called cellular respiration. This process converts the chemical energy stored in organic molecules into ATP, which the cell uses to perform work. The waste products are carbon dioxide and water. Cells are more efficient than cars, converting about 34% of the chemical energy into energy for cellular work, while the remaining 66% generates heat, explaining why vigorous exercise makes you warm. According to the second law of thermodynamics, energy transformations increase disorder in the universe. However, biological order is maintained because cells use ordered forms of matter and energy from their surroundings. Cells extract chemical energy from glucose and release disordered heat, carbon dioxide, and water. Thus, cells are islands of low entropy in an increasingly random universe. How does the second law of thermodynamics explain the diffusion of a solute across a membrane? Diffusion leads to equal concentrations of solute across a membrane, which increases entropy because it creates a more disordered arrangement. When solute molecules are evenly distributed, they are in a state of higher randomness compared to when they are concentrated on one side. This increase in disorder aligns with the second law of thermodynamics, which states that energy transformations increase the entropy of the universe. **Terms to Know** **active transport**-The movement of a substance across a biological membrane against its concentration gradient, aided by specific transport proteins and requiring an input of energy (often as ATP). **5.11 Chemical reactions either release or store energy** Chemical reactions can be exergonic or endergonic. Exergonic reactions release energy (\"energy outward\"). They start with reactants that have more potential energy in their covalent bonds than the products. The reaction releases energy equal to the difference in potential energy between the reactants and products. When wood burns, its cellulose, an energy-rich carbohydrate made of glucose monomers, releases energy as heat and light. The reaction produces carbon dioxide and water as byproducts. Cellulose is a larger molecule composed of many glucose monomers linked together. Because it has more bonds, it stores more potential energy. When cellulose burns, it releases more energy compared to a single glucose molecule due to the greater number of high-energy bonds being broken. Cells release energy from fuel molecules through cellular respiration, like burning, as both are exergonic processes. However, burning releases all energy at once in a single step, while cellular respiration involves many steps, making it a \"slow burn.\" Much of the energy from cellular respiration escapes as heat, but a significant amount is stored in ATP, the cell\'s immediate energy source. Endergonic reactions require a net input of energy and produce products rich in potential energy. These reactions start with reactants that have relatively little potential energy. As the reaction occurs, energy is absorbed from the surroundings, resulting in products with more chemical energy than the reactants. Photosynthesis is an endergonic process where plant cells make sugar. It begins with energy-poor reactants, such as carbon dioxide and water, and uses energy from sunlight to produce energy-rich sugar molecules. This process increases the potential energy of the products compared to the reactants, thanks to the input of sunlight. Living cells perform thousands of exergonic and endergonic reactions, collectively known as metabolism. Metabolism can be visualized as a network of chemical reactions, or metabolic pathways, that either build complex molecules or break them down into simpler compounds. Cellular respiration is an example of a metabolic pathway, where a series of reactions gradually release the potential energy stored in sugar. All an organism\'s activities require energy, which is obtained from sugar and other molecules through exergonic reactions in cellular respiration. Cells use this energy in endergonic reactions to build molecules and perform cellular work. Energy coupling involves using energy released from exergonic reactions to drive endergonic reactions, with ATP molecules playing a key role in this process. Remembering that energy must be conserved, what do you think becomes of the energy extracted from food during cellular respiration? Some of it is stored in ATP molecules; the rest is released as heat. **Terms to Know** **Energy**-The capacity to cause change, especially to perform work. **chemical energy**-Energy available in molecules for release in a chemical reaction; a form of potential energy. **Entropy**-A measure of disorder, or randomness. See also second law of thermodynamics. -------------------------------------------------------------------------------------------------------------------------------------- -------------------------------------------------------------------------------------------------------------------------------------------------------------------------------------------------------------------------------------------------------------------------------------------------------------------------------------------------------------------------------------------------- --------------------------------------------------------------------------------------------------------------------------------------------------------------------------------------------------------------- **endergonic reactions**-An energy-requiring chemical reaction, which yields products with more potential energy than the reactants. **cellular respiration***-*The aerobic harvesting of energy from food molecules; the energy-releasing chemical breakdown of food molecules, such as glucose, and the storage of potential energy in a form that cells can use to perform work; involves glycolysis, pyruvate oxidation and the citric acid cycle, and oxidative phosphorylation (the electron transport chain and chemiosmosis). **exergonic reactions**-An energy-releasing chemical reaction in which the reactants contain more potential energy than the products. **Heat***-*Thermal energy in transfer from one body of matter to another. **potential energy**-The energy that matter possesses because of its location or spatial arrangement. Water behind a dam possesses potential energy, and so do chemical bonds. **Thermodynamics**-The study of energy transformation that occurs in a collection of matter. See also first law of thermodynamics; second law of thermodynamics. **kinetic energy***-*The energy associated with the motion of objects. Moving matter does work by imparting motion to other matter. **first law of thermodynamics**-The principle of conservation of energy. Energy can be transferred and transformed, but it cannot be created or destroyed. **second law of thermodynamics**-The principle stating that every energy conversion reduces the order of the universe, increasing its entropy. Ordered forms of energy are at least partly converted to heat. **cellular metabolism**-The totality of an organism's chemical reactions. **5.12 ATP drives cellular work by coupling exergonic and endergonic reactions** ATP, or adenosine triphosphate, is essential for nearly all cellular activities. It consists of an organic molecule called adenosine and a tail of three negatively charged phosphate groups. The repulsion between these like charges makes the triphosphate tail of ATP like a compressed spring, storing potential energy for cellular work. The bonds between ATP\'s phosphate groups are unstable and can be easily broken by hydrolysis, which involves the addition of water. When the bond to the third phosphate group breaks, ATP becomes ADP (adenosine diphosphate), and energy is released. The hydrolysis of ATP releases energy, making it an exergonic reaction. Cells couple this reaction to endergonic (energy-requiring) reactions by transferring a phosphate group from ATP to another molecule. This process, known as phosphorylation, is crucial for cellular work, as it energizes molecules by adding a phosphate group to them. Cells perform three main types of work, all powered by ATP: 1. **Chemical Work**: ATP phosphorylates reactants, providing energy for the endergonic synthesis of products. 2. **Transport Work**: ATP drives the active transport of solutes across membranes against their concentration gradients by phosphorylating transport proteins. 3. **Mechanical Work**: In muscle cells, ATP hydrolysis causes motor proteins to change shape and pull on other protein filaments, leading to cell contraction. ATP is a renewable resource that cells continuously use and regenerate. The ATP cycle involves energy coupling: energy from exergonic reactions, like glucose breakdown during cellular respiration, is used to generate ATP by bonding a phosphate group to ADP. The hydrolysis of ATP then releases energy to drive endergonic reactions. This cycle operates rapidly, with a working muscle cell consuming and regenerating 10 million ATP molecules each second. Explain how ATP transfers energy from exergonic to endergonic processes in the cell. Exergonic processes phosphorylate ADP to form ATP. ATP transfers energy to endergonic processes, often by phosphorylating other molecules. **Terms to Know** **ATP**-Adenosine triphosphate, the main energy source for cells. ATP releases energy when its phosphate bonds are hydrolyzed. **energy coupling**-In cellular metabolism, the use of energy released from an exergonic reaction to drive an endergonic reaction. **ADP**-(adenosine diphosphate) is a molecule that consists of adenosine and two phosphate groups. It plays a key role in cellular energy transfer, as it can be converted to ATP (adenosine triphosphate) by adding a phosphate group, storing energy for the cell to use. **Phosphorylation**-The transfer of a phosphate group, usually from ATP to a molecule. Nearly all cellular work depends on ATP energizing other molecules by phosphorylation. **5.13 Enzymes speed up the cell\'s chemical reactions by lowering energy barriers** Ordered structures naturally tend toward disorder, and high-energy systems move to more stable, low-energy states. Complex molecules in cells, like proteins and DNA, are rich in potential energy but don\'t spontaneously break down into simpler components. They remain intact for the same reason wood doesn\'t spontaneously combust or gasoline doesn\'t explode on its own. Before a chemical reaction can start, an energy barrier called activation energy must be overcome. This energy is needed to contort or weaken bonds in reactant molecules, allowing them to break and form new bonds. Activation energy can be thought of as the energy required for reactant molecules to move to a higher-energy, unstable state, enabling the reaction to proceed. The activation energy barrier prevents highly ordered molecules in cells from spontaneously breaking down. However, life relies on numerous chemical reactions that constantly alter a cell\'s molecular makeup. For a cell to survive, these metabolic reactions must occur quickly and precisely. The challenge is how to overcome this energy barrier for the necessary reactions to proceed efficiently. Adding heat can speed up reactions by increasing molecular movement and breaking bonds more easily. For example, a match ignites kindling, and a spark plug ignites gasoline. However, heating a cell would accelerate all chemical reactions, not just the necessary ones, and excessive heat would be lethal to the cell. Enzymes are biological catalysts that speed up reactions without being consumed. Most enzymes are proteins, though some RNA molecules also function as enzymes. They work by lowering the activation energy needed for a reaction to start, allowing the reaction to proceed more rapidly. This lowered energy barrier enables enzyme-catalyzed reactions to occur much faster than they would without enzymes. **Terms to Know** **energy of activation**-the amount of energy that reactants must absorb before a chemical reaction can start. **Substrate**-a specific substance (reactant) on which an enzyme acts. Each enzyme recognizes only the specific substrate or substrates of the reaction it catalyzes. **5.14 A specific enzyme catalyzes each cellular reaction** Enzymes catalyze reactions by lowering the activation energy barrier. They do this by contorting the bonds in reactant molecules into a higher-energy, unstable state, allowing the reaction to proceed. Without enzymes, the activation energy barrier might never be overcome. For example, sucrose can sit for years without breaking down, but with a small amount of the enzyme sucrase, it hydrolyzes into glucose and fructose within seconds. Enzymes are highly selective in the reactions they catalyze due to their unique three-dimensional shapes, which determine their specificity. The specific reactant an enzyme acts on is called its substrate. The substrate fits into the enzyme\'s active site, typically a pocket or groove on the enzyme\'s surface. This specificity ensures that only particular substrate molecules can fit into the enzyme\'s active sites, allowing precise catalysis of reactions. **The Catalytic Cycle** The catalytic cycle of an enzyme, such as sucrase, involves several steps: 1\. The enzyme begins with an empty active site. 2\. Sucrose enters the active site, attaching by weak bonds. The active site changes shape slightly, embracing the substrate more snugly, like a firm handshake. This induced fit may contort substrate bonds or position chemical groups of the amino acids in the active site to catalyze the reaction. In reactions involving multiple reactants, the active site holds the substrates in the proper orientation for the reaction to occur. 3\. The strained bond of sucrose reacts with water, converting (hydrolyzing) it into glucose and fructose. 4\. The enzyme releases the products and remains unchanged, with its active site ready for another substrate molecule. This cycle can repeat rapidly, with a single enzyme molecule acting on thousands or even millions of substrate molecules per second. **Optimal Conditions for Enzymes** An enzyme\'s shape is crucial to its function, and this shape is influenced by environmental conditions. Each enzyme has optimal conditions, such as temperature, under which it is most effective. Higher temperatures can denature enzymes, altering their shape and function. Most human enzymes work best at 35--40°C (95--104°F), close to normal body temperature. However, enzymes in prokaryotes living in hot springs have optimal temperatures of 70°C (158°F) or higher. Scientists utilize these high-temperature enzymes to rapidly replicate DNA sequences from small samples. Most enzymes function best at a neutral pH, around 6--8. However, there are exceptions, such as pepsin, a digestive enzyme in the stomach, which works optimally at pH 2. This highly acidic environment would denature most proteins, but pepsin\'s structure is stable and active under these conditions. **Cofactors** Many enzymes need nonprotein helpers called cofactors, which bind to the active site and aid in catalysis. Some cofactors are inorganic, like zinc, iron, and copper ions. Organic cofactors are called coenzymes. Many vitamins are crucial in nutrition because they act as coenzymes or are precursors to coenzymes. For example, folic acid, a B vitamin, is a coenzyme for several enzymes involved in nucleic acid synthesis. If all a cell\'s metabolic pathways operated simultaneously, it would lead to chemical chaos. A cell\'s regulated metabolism relies on the coordination of key molecular players. Cells control enzyme activity by either switching genes on or off or regulating the activity of enzymes once they are made. This ensures that enzymes are active only when and where they are needed. Explain how an enzyme speeds up a specific reaction. An enzyme lowers the activation energy needed for a reaction when its specific substrate enters its active site. With an induced fit, the enzyme strains bonds that need to break or positions substrates in an orientation that aids the conversion of reactants to products. **5.15 Enzyme inhibition can regulate enzyme activity in a cell** An inhibitor is a chemical that interferes with an enzyme\'s activity. Scientists study inhibitors to understand enzyme function. Some inhibitors resemble the enzyme\'s normal substrate and compete for entry into the active site, reducing the enzyme\'s productivity. This competitive inhibition can be overcome by increasing the substrate concentration, making it more likely for a substrate molecule to enter the active site instead of an inhibitor. A noncompetitive inhibitor binds to a different site on the enzyme, causing a change in the enzyme\'s shape. This alteration prevents the substrate from fitting into the active site. Cells use inhibitors to regulate metabolism. Many chemical reactions in cells are organized into metabolic pathways, where each step is catalyzed by a specific enzyme. If a cell produces more of a product than needed, the product can inhibit an enzyme early in the pathway, a process called feedback inhibition. This inhibition is reversible, allowing the pathway to function again when the product is used up. Explain an advantage of feedback inhibition to a cell. Feedback inhibition is beneficial to cells because it helps regulate metabolic pathways efficiently. When a cell produces more of a product than it needs, the product can inhibit an enzyme early in the pathway, preventing the unnecessary accumulation of the product. This conserves resources and energy, ensuring that the cell only produces what it needs. Additionally, feedback inhibition is reversible, allowing the pathway to function again when the product is used up, maintaining balance within the cell.