Microbiology Chapter 4: Functional Anatomy of Prokaryotic and Eukaryotic Cells PDF
Document Details
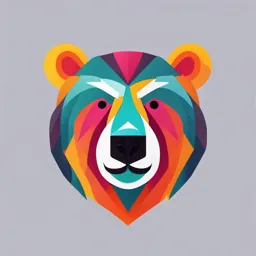
Uploaded by UseableAgate1291
San Joaquin Valley College
2016
Tags
Summary
This chapter from a textbook on microbiology provides an overview of the functional anatomy of prokaryotic and eukaryotic cells. It details various cellular components and structures, including the cell wall, membrane, and other organelles found in these cells. The text includes examples like bacterial shapes and explains concepts such as osmosis and active transport.
Full Transcript
Microbiology an Introduction Twelfth Edition Chapter 4 Functional Anatomy of Prokaryotic and Eukaryotic Cells...
Microbiology an Introduction Twelfth Edition Chapter 4 Functional Anatomy of Prokaryotic and Eukaryotic Cells Copyright © 2016 Pearson Education, Inc. All Rights Reserved Comparing Prokaryotic and Eukaryotic Cells: An Overview Prokaryote comes from the Greek words for prenucleus. Eukaryote comes from the Greek words for true nucleus. Copyright © 2016 Pearson Education, Inc. All Rights Reserved Comparing Prokaryotic and Eukaryotic Cells: An Overview Prokaryote Eukaryote One circular chromosome, not DNA in nucleus in a membrane Paired chromosomes, No histones in nuclear membrane No organelles Histones Bacteria: peptidoglycan cell Organelles walls Simple cell walls, when Archaea: pseudomurein cell present walls Divides by mitosis Divides by binary fission Copyright © 2016 Pearson Education, Inc. All Rights Reserved The Size, Shape, and Arrangement of Bacterial Cells Average size: 0.2 to 2.0 µm diameter × 2 to 8 µm length Most bacteria are monomorphic (single shape) A few are pleomorphic (many shapes) Copyright © 2016 Pearson Education, Inc. All Rights Reserved The Size, Shape, and Arrangement of Bacterial Cells Bacillus (rod-shaped) Coccus (spherical) Spiral – Vibrio – Spirillum – Spirochete Star-shaped Rectangular Copyright © 2016 Pearson Education, Inc. All Rights Reserved Figure 4.5a Star-Shaped and Rectangular Prokaryotes Copyright © 2016 Pearson Education, Inc. All Rights Reserved Figure 4.5b Star-Shaped and Rectangular Prokaryotes Copyright © 2016 Pearson Education, Inc. All Rights Reserved The Size, Shape, and Arrangement of Bacterial Cells Pairs: diplococci, diplobacilli Chains: streptococci, streptobacilli Groups of four: tetrads Cubelike groups of eight: sarcinae Clusters: staphylococci Copyright © 2016 Pearson Education, Inc. All Rights Reserved Figure 4.6 The Structure of a Prokaryotic Cell Copyright © 2016 Pearson Education, Inc. All Rights Reserved Glycocalyx Outside the cell wall Viscous and gelatinous Made of polysaccharide and/or polypeptide Two types – Capsule: neatly organized and firmly attached to cell – Slime layer: unorganized and loose Contribute to virulence – Capsules prevent phagocytosis – Helps form biofilms and prevent dehydration/ nutrients Copyright © 2016 Pearson Education, Inc. All Rights Reserved Flagella Filamentous appendages outside of the cell Propel bacteria (by rotation or tumble) Made of protein flagellin Copyright © 2016 Pearson Education, Inc. All Rights Reserved Flagella Three parts: – Filament: outermost region – Hook: attaches to the filament – Basal body: consists of rod and pairs of rings; anchors flagellum to the cell wall and membrane Copyright © 2016 Pearson Education, Inc. All Rights Reserved Flagella Flagella allow bacteria to move toward or away from stimuli (taxis) Flagella rotate to "run" or "tumble" Copyright © 2016 Pearson Education, Inc. All Rights Reserved Axial Filaments Also called endoflagella Found in spirochetes Anchored at one end of a cell Rotation causes cell to move like a corkscrew Copyright © 2016 Pearson Education, Inc. All Rights Reserved Fimbriae and Pili Fimbriae – Hairlike appendages that allow for attachment (to each other and surfaces) – Needed for colonization – Biofilms Copyright © 2016 Pearson Education, Inc. All Rights Reserved Fimbriae and Pili Pili – Involved in motility (gliding and twitching motility) – Conjugation pili involved in DNA transfer from one cell to another Copyright © 2016 Pearson Education, Inc. All Rights Reserved The Cell Wall Shape of cell Prevents osmotic lysis and protects the cell membrane Made of peptidoglycan (in bacteria) Contributes to pathogenicity Chemical composition helps differentiate types of bacteria Copyright © 2016 Pearson Education, Inc. All Rights Reserved Composition and Characteristics Bacterial Cell Wall Peptidoglycan (murein) – Polymer of a repeating disaccharide in rows: N-acetylglucosamine (NAG) N-acetylmuramic acid (NAM) Rows are linked by polypeptides Copyright © 2016 Pearson Education, Inc. All Rights Reserved Gram-Positive Cell Walls Thick peptidoglycan cell wall Teichoic acids Susceptible to penicillin (Penicillin inhibits peptide bridges in peptidoglycan) Copyright © 2016 Pearson Education, Inc. All Rights Reserved Gram-Negative Cell Walls Thin peptidoglycan and outer membrane Low susceptibility to penicillin (Penicillin inhibits peptide bridges in peptidoglycan) More susceptible to mechanical breakage Copyright © 2016 Pearson Education, Inc. All Rights Reserved Gram Stain Copyright © 2016 Pearson Education, Inc. All Rights Reserved Atypical Cell Walls Acid-fast cell walls – Waxy lipid (mycolic acid) bound to peptidoglycan – Stain with carbolfuchsin Example: – Mycobacterium – Nocardia Mycobacterium Tuberculosis Copyright © 2016 Pearson Education, Inc. All Rights Reserved Atypical Cell Walls Mycoplasmas – Lack cell walls – Sterols in plasma membrane to prevent lysis Archaea – Wall-less, or – Walls of pseudomurein (lack NAM and D-amino acids) Copyright © 2016 Pearson Education, Inc. All Rights Reserved The Plasma (Cytoplasmic) Membrane Phospholipid bilayer that encloses the cytoplasm Peripheral proteins on the membrane surface Integral and transmembrane proteins penetrate the membrane Copyright © 2016 Pearson Education, Inc. All Rights Reserved Structure Fluid mosaic model – Membrane is as viscous as olive oil – Proteins move freely for various functions – Phospholipids rotate and move laterally – Self-sealing Copyright © 2016 Pearson Education, Inc. All Rights Reserved Functions The plasma membrane's selective permeability allows the passage of some molecules, but not others Contain enzymes for ATP production Some membranes have photosynthetic pigments on foldings called chromatophores Damage to the membrane by alcohols, quaternary ammonium (detergents), and polymyxin antibiotics causes leakage of cell contents Copyright © 2016 Pearson Education, Inc. All Rights Reserved The Movement of Materials Across Membranes Passive processes: substances move from high concentration to low concentration; no energy expended Active processes: substances move from low concentration to high concentration; energy expended Copyright © 2016 Pearson Education, Inc. All Rights Reserved Passive Processes Simple diffusion: movement of a solute from an area of high concentration to an area of low concentration Continues until molecules reach equilibrium Copyright © 2016 Pearson Education, Inc. All Rights Reserved Passive Processes Facilitated diffusion: solute combines with a transporter protein in the membrane Transports ions and larger molecules across a membrane with the concentration gradient Copyright © 2016 Pearson Education, Inc. All Rights Reserved Passive Processes Osmosis: the movement of water across a selectively permeable membrane from an area of high water to an area of lower water concentration Through lipid layer Aquaporins (water channels) Osmotic pressure: the pressure needed to stop the movement of water across the membrane Copyright © 2016 Pearson Education, Inc. All Rights Reserved Figure 4.18a-b The Principle of Osmosis Copyright © 2016 Pearson Education, Inc. All Rights Reserved Passive Processes Isotonic solution: solute concentrations equal inside and outside of cell; water is at equilibrium Hypotonic solution: solute concentration is lower outside than inside the cell; water moves into cell Hypertonic solution: solute concentration is higher outside of cell than inside; water moves out of cell Cell tonicity is 0.9% NaCl Copyright © 2016 Pearson Education, Inc. All Rights Reserved Active Processes Active transport: requires a transporter protein and ATP; goes against gradient Group translocation: requires a transporter protein and phosphoenolpyruvic acid (PEP); substance is altered as it crosses the membrane and cannot cross back Copyright © 2016 Pearson Education, Inc. All Rights Reserved Cytoplasm The substance inside the plasma membrane Eighty percent water plus proteins, carbohydrates, lipids, and ions Cytoskeleton (small fibers in the cytoplasm) Copyright © 2016 Pearson Education, Inc. All Rights Reserved The Nucleoid and Plasmids Bacterial chromosome: circular thread of DNA that contains the cell's genetic information in nucleoid. Plasmids: extrachromosomal genetic elements; carry non-crucial genes (e.g., antibiotic resistance, production of toxins) Copyright © 2016 Pearson Education, Inc. All Rights Reserved Ribosomes Sites of protein synthesis Made of protein and ribosomal RNA subunits 70S – 50S + 30S subunits The Prokaryotic Ribosome Copyright © 2016 Pearson Education, Inc. All Rights Reserved Endospores Resting cells; produced when nutrients are depleted Resistant to desiccation, heat, chemicals, and radiation when released Not for reproduction Produced by Bacillus and Clostridium Sporulation: endospore formation Germination: endospore returns to vegetative state and can resume metabolism Copyright © 2016 Pearson Education, Inc. All Rights Reserved Eukaryotic Cells Prokaryotic and Eukaryotic Cells – Prokaryote comes from the Greek words for prenucleus. – Eukaryote comes from the Greek words for true nucleus. Copyright © 2016 Pearson Education, Inc. All Rights Reserved Figure 4.22a Eukaryotic Cells Showing Typical Structures Copyright © 2016 Pearson Education, Inc. All Rights Reserved Figure 4.22b Eukaryotic Cells Showing Typical Structures (b) Transmission electron micrograph of plant cell Copyright © 2016 Pearson Education, Inc. All Rights Reserved Flagella and Cilia Projections used for locomotion or moving substances along the cell surface Flagella—long projections; few in number Cilia—short projections; numerous Copyright © 2016 Pearson Education, Inc. All Rights Reserved Flagella and Cilia Both consist of microtubules made of the protein tubulin Microtubules are organized as nine pairs in a ring, plus two microtubules in the center (9 + 2 array) Allow flagella to move in a wavelike manner Prokaryotic flagella rotate, eukaryotic flagella wave Copyright © 2016 Pearson Education, Inc. All Rights Reserved The Cell Wall and Glycocalyx Cell Wall – Found in plants, algae, and fungi – Made of carbohydrates (cellulose—plants, chitin— fungi, glucan and mannan—yeasts) Glycocalyx – Carbohydrates bonded to proteins and lipids in the plasma membrane (sticky) – Found in animal cells – Strengthens plasma membrane Copyright © 2016 Pearson Education, Inc. All Rights Reserved The Plasma (Cytoplasmic) Membrane Similar in structure to prokaryotic cell membranes – Phospholipid bilayer – Integral and peripheral proteins Differences in structure – Sterols—complex lipids – resists lysis – Carbohydrates—for attachment and cell-to-cell recognition Copyright © 2016 Pearson Education, Inc. All Rights Reserved The Plasma (Cytoplasmic) Membrane Similar in function to prokaryotic cell membranes – Selective permeability – Simple diffusion, facilitated diffusion, osmosis, active transport Differences in function – Endocytosis— engulf solid or liquid into the cell – Phagocytosis: pseudopods extend and engulf solid particles – Pinocytosis: membrane folds inward, bringing in fluid and dissolved substances Copyright © 2016 Pearson Education, Inc. All Rights Reserved Cytoplasm Cytoplasm: substance inside the plasma and outside the nucleus Cytosol: fluid portion of cytoplasm Cytoskeleton: made of microfilaments and intermediate filaments; gives shape and support Cytoplasmic streaming: movement of the cytoplasm throughout a cell Copyright © 2016 Pearson Education, Inc. All Rights Reserved Ribosomes Sites of protein synthesis 80S – Consists of the large 60S subunit and the small 40S subunit – Membrane-bound: attached to endoplasmic reticulum – Free: in cytoplasm 70S – In chloroplasts and mitochondria Copyright © 2016 Pearson Education, Inc. All Rights Reserved The Nucleus Nucleus – Double membrane structure (nuclear envelope) that contains the cell's DNA – DNA is complexed with histone proteins to form chromatin – During mitosis and meiosis, chromatin condenses into chromosomes Copyright © 2016 Pearson Education, Inc. All Rights Reserved Endoplasmic Reticulum Folded transport network Rough ER: studded with ribosomes; sites of protein synthesis Smooth ER: no ribosomes; synthesizes cell membranes, fats, and hormones Copyright © 2016 Pearson Education, Inc. All Rights Reserved Golgi Complex Transport organelle Modifies proteins from the ER Transports modified proteins via secretory vesicles to the plasma membrane Copyright © 2016 Pearson Education, Inc. All Rights Reserved Mitochondria Double membrane Contain inner folds (cristae) and fluid (matrix) Involved in cellular respiration (ATP production) Copyright © 2016 Pearson Education, Inc. All Rights Reserved Chloroplasts Locations of photosynthesis Contain flattened membranes (thylakoids) that contain chlorophyll Copyright © 2016 Pearson Education, Inc. All Rights Reserved Organelles Lysosomes – Vesicles formed in the Golgi complex – Contain digestive enzymes Vacuoles – Cavities in the cell formed from the Golgi complex – Bring food into cells; provide shape and storage Copyright © 2016 Pearson Education, Inc. All Rights Reserved Organelles Peroxisomes – Oxidize fatty acids; destroy H2O2 Centrosomes – Networks of protein fibers and centrioles – Form the mitotic spindle; critical role in cell division Copyright © 2016 Pearson Education, Inc. All Rights Reserved Microbiology an Introduction Twelfth Edition Chapter 5 Microbial Metabolism Copyright © 2016 Pearson Education, Inc. All Rights Reserved Dental Plaque Consists of Bacteria Copyright © 2016 Pearson Education, Inc. All Rights Reserved Big Picture: Metabolism (1 of 2) Metabolism is the buildup and breakdown of nutrients within a cell These chemical reactions provide energy and create substances that sustain life Copyright © 2016 Pearson Education, Inc. All Rights Reserved Big Picture pg. 108 Copyright © 2016 Pearson Education, Inc. All Rights Reserved Big Picture: Metabolism (2 of 2) Although microbial metabolism can cause disease and food spoilage, many pathways are beneficial rather than pathogenic Copyright © 2016 Pearson Education, Inc. All Rights Reserved Big Picture pg. 109 Copyright © 2016 Pearson Education, Inc. All Rights Reserved Catabolic and Anabolic Reactions (2 of 3) Catabolism: breaks down complex molecules; provides energy and building blocks for anabolism; exergonic Anabolism: uses energy and building blocks to build complex molecules; endergonic Copyright © 2016 Pearson Education, Inc. All Rights Reserved Figure 5.1 The role of ATP in Coupling Anabolic and Catabolic Reactions Copyright © 2016 Pearson Education, Inc. All Rights Reserved Catabolic and Anabolic Reactions (3 of 3) Metabolic pathways are sequences of enzymatically catalyzed chemical reactions in a cell Metabolic pathways are determined by enzymes Enzymes are encoded by genes Copyright © 2016 Pearson Education, Inc. All Rights Reserved Collision Theory The collision theory states that chemical reactions occur when atoms, ions, and molecules collide Activation energy is the collision energy required for a chemical reaction to occur Reaction rate is the frequency of collisions containing enough energy to bring about a reaction – Reaction rate can be increased by enzymes or by increasing temperature, pressure, or concentration Copyright © 2016 Pearson Education, Inc. All Rights Reserved Enzymes and Chemical Reactions (1 of 2) Catalysts speed up chemical reactions without being altered Enzymes are biological catalysts Enzymes act on a specific substrate and lower the activation energy Copyright © 2016 Pearson Education, Inc. All Rights Reserved Enzymes and Chemical Reactions (2 of 2) Substrate contacts the enzyme’s active site to form an enzyme-substrate complex Substrate is transformed and rearranged into products, which are released from the enzyme Enzyme is unchanged and can react with other substrates Copyright © 2016 Pearson Education, Inc. All Rights Reserved Figure 5.3a The Mechanism of Enzymatic Action Copyright © 2016 Pearson Education, Inc. All Rights Reserved Figure 5.3b The Mechanism of Enzymatic Action Copyright © 2016 Pearson Education, Inc. All Rights Reserved Enzyme Specificity and Efficiency Enzymes have specificity for particular substrates Turnover number is the number of substrate molecules an enzyme converts to a product per second – Generally 1 to 10,000 Copyright © 2016 Pearson Education, Inc. All Rights Reserved Naming Enzymes Names of enzymes usually end in ase; grouped based on the reaction they catalyze Oxidoreductase: oxidation-reduction reactions Transferase: transfer functional groups Hydrolase: hydrolysis Lyase: removal of atoms without hydrolysis Isomerase: rearrangement of atoms Ligase: joining of molecules; uses ATP Copyright © 2016 Pearson Education, Inc. All Rights Reserved Figure 5.4 Components of a Holoenzyme Copyright © 2016 Pearson Education, Inc. All Rights Reserved Factors Influencing Enzyme Activity (1 of 2) Temperature pH Substrate concentration Inhibitors Copyright © 2016 Pearson Education, Inc. All Rights Reserved Factors Influencing Enzyme Activity (2 of 2) High temperature and extreme pH denature proteins If the concentration of substrate is high (saturation), the enzyme catalyzes at its maximum rate Copyright © 2016 Pearson Education, Inc. All Rights Reserved Figure 5.6 Denaturation of a Protein Copyright © 2016 Pearson Education, Inc. All Rights Reserved Figure 5.5a Factors that Influence Enzymatic Activity, Plotted for a Hypothetical Enzyme (a) Temperature. The enzymatic activity (rate of reaction catalyzed by the enzyme) increases with increasing temperature until the enzyme, a protein, is denatured by heat and inactivated. At this point, the reaction rate falls steeply. Copyright © 2016 Pearson Education, Inc. All Rights Reserved Figure 5.5b Factors that Influence Enzymatic Activity, Plotted for a Hypothetical Enzyme (b) pH. The enzyme illustrated is most active at about pH 5.0. Copyright © 2016 Pearson Education, Inc. All Rights Reserved Figure 5.5c Factors that Influence Enzymatic Activity, Plotted for a Hypothetical Enzyme (c) Substrate concentration. With increasing concentration of substrate molecules, the rate of reaction increases until the active sites on all the enzyme molecules are filled, at which point the maximum rate of reaction is reached. Copyright © 2016 Pearson Education, Inc. All Rights Reserved Inhibitors (1 of 2) Competitive inhibitors fill the active site of an enzyme and compete with the substrate Copyright © 2016 Pearson Education, Inc. All Rights Reserved Figure 5.7a-b Enzyme Inhibitors Copyright © 2016 Pearson Education, Inc. All Rights Reserved Unnumbered Figure pg. 115 Copyright © 2016 Pearson Education, Inc. All Rights Reserved Inhibitors (2 of 2) Noncompetitive inhibitors interact with another part of the enzyme (allosteric site) rather than the active site in a process called allosteric inhibition Copyright © 2016 Pearson Education, Inc. All Rights Reserved Figure 5.7a-c Enzyme Inhibitors Copyright © 2016 Pearson Education, Inc. All Rights Reserved Feedback Inhibition End-product of a reaction allosterically inhibits enzymes from earlier in the pathway Copyright © 2016 Pearson Education, Inc. All Rights Reserved Figure 5.8 Feedback Inhibition Copyright © 2016 Pearson Education, Inc. All Rights Reserved Ribozymes RNA that function as catalysts by cutting and splicing RNA Copyright © 2016 Pearson Education, Inc. All Rights Reserved Oxidation-Reduction Reactions (1 of 3) Oxidation: removal of electrons Reduction: gain of electrons Redox reaction: an oxidation reaction paired with a reduction reaction Copyright © 2016 Pearson Education, Inc. All Rights Reserved Figure 5.9 Oxidation-Reduction Copyright © 2016 Pearson Education, Inc. All Rights Reserved Oxidation-Reduction Reactions (3 of 3) In biological systems, electrons and protons are removed at the same time; equivalent to a hydrogen atom Biological oxidations are often dehydrogenations Copyright © 2016 Pearson Education, Inc. All Rights Reserved Metabolic Pathways of Energy Production Series of enzymatically catalyzed chemical reactions Extracts energy from organic compounds and stores it in chemical form (ATP) Copyright © 2016 Pearson Education, Inc. All Rights Reserved Carbohydrate Catabolism (2 of 2) The breakdown of carbohydrates to release energy – Glycolysis – Krebs cycle – Electron transport chain (system) Copyright © 2016 Pearson Education, Inc. All Rights Reserved Glycolysis (1 of 4) The oxidation of glucose to pyruvic acid produces ATP and NADH Copyright © 2016 Pearson Education, Inc. All Rights Reserved Cellular Respiration Oxidation of molecules liberates electrons to operate an electron transport chain Final electron acceptor comes from outside the cell and is inorganic ATP is generated by oxidative phosphorylation Copyright © 2016 Pearson Education, Inc. All Rights Reserved Aerobic Respiration (1 of 5) Krebs cycle – Pyruvic acid (from glycolysis) is oxidized and decarboxylation (loss of CO2) occurs – The resulting two-carbon compound attaches to coenzyme A, forming acetyl CoA and NADH Copyright © 2016 Pearson Education, Inc. All Rights Reserved Aerobic Respiration (2 of 5) Krebs cycle – Oxidation of acetyl CoA produces NADH, FADH2, and ATP, and liberates CO2 as waste Copyright © 2016 Pearson Education, Inc. All Rights Reserved Carbohydrate Catabolism Each NADH can be oxidized in the electron transport chain to produce 3 molecules of ATP Each FADH2 can produce 2 molecules of ATP Copyright © 2016 Pearson Education, Inc. All Rights Reserved Anaerobic Respiration (1 of 2) The final electron acceptor in the electron transport chain is NOT O2 – Yields less energy than aerobic respiration Copyright © 2016 Pearson Education, Inc. All Rights Reserved Anaerobic Respiration (2 of 2) Copyright © 2016 Pearson Education, Inc. All Rights Reserved Table 5.3 ATP Yield During Prokaryotic Aerobic Respiration of One Glucose Molecule TABLE 5.3 ATP Yield during Prokaryotic Aerobic Respiration of One Glucose Molecule Copyright © 2016 Pearson Education, Inc. All Rights Reserved Fermentation (1 of 3) Releases energy from the oxidation of organic molecules Does not require oxygen Does not use the Krebs cycle or ETC Uses an organic molecule as the final electron acceptor Produces only small amounts of ATP Copyright © 2016 Pearson Education, Inc. All Rights Reserved Figure 5.18a Fermentation Copyright © 2016 Pearson Education, Inc. All Rights Reserved Fermentation (2 of 3) Lactic acid fermentation: produces lactic acid – Homolactic fermentation: produces lactic acid only – Heterolactic fermentation: produces lactic acid and other compounds Glucose is oxidized to pyruvic acid, which is then reduced by NADH Copyright © 2016 Pearson Education, Inc. All Rights Reserved Fermentation (3 of 3) Alcohol fermentation: produces ethanol + CO2 Glucose is oxidized to pyruvic acid; pyruvic acid is converted to acetaldehyde and CO2; NADH reduces acetaldehyde to ethanol Copyright © 2016 Pearson Education, Inc. All Rights Reserved Figure 5.19 Types of Fermentation Copyright © 2016 Pearson Education, Inc. All Rights Reserved Figure 5.18b Fermentation Copyright © 2016 Pearson Education, Inc. All Rights Reserved Table 5.4 Some Industrial Uses for Different Types of Fermentations* (1 of 2) TABLE 5.4 Some Industrial Uses for Different Types of Fermentations* Fermentation End- Industrial or Starting Material Microorganism Product(s) Commercial Use Ethanol Beer, wine Starch, sugar Saccharomyces cerevisiae (yeast, a fungus) Blank Fuel Agricultural wastes Saccharomyces cerevisiae (yeast) Acetic Acid Vinegar Ethanol Acetobacter Lactic Acid Cheese, yogurt Milk Lactobacillus, Streptococcus Blank Rye bread Grain, sugar Lactobacillus delbrueckii Blank Sauerkraut Cabbage Lactobacillus plantarum Blank Summer sausage Meat Pediococcus Copyright © 2016 Pearson Education, Inc. All Rights Reserved Table 5.4 Some Industrial Uses for Different Types of Fermentations* (2 of 2) Fermentation End- Industrial or Starting Material Microorganism Product(s) Commercial Use Propionic Acid and Swiss cheese Lactic acid Propionibacterium Carbon Dioxide freudenreichii Acetone and Butanol Pharmaceutical, Molasses Clostridium industrial uses acetobutylicum Citric Acid Flavoring Molasses Aspergillus (fungus) Methane Fuel Acetic acid Methanosarcina (archaeon) Sorbose Vitamin C (ascorbic Sorbitol Gluconobacter acid) *Unless otherwise noted, the microorganisms listed are bacteria. Copyright © 2016 Pearson Education, Inc. All Rights Reserved Figure 5.11 An Overview of Respiration and Fermentation (2 of 2) Copyright © 2016 Pearson Education, Inc. All Rights Reserved Lipid and Protein Catabolism (2 of 2) Extracellular proteases Protein Amino acids Deamination, decarboxylation, dehydrogenation, desulfurization Organic acid Krebs cycle Copyright © 2016 Pearson Education, Inc. All Rights Reserved Figure 5.20 Lipid Catabolism Copyright © 2016 Pearson Education, Inc. All Rights Reserved Figure 5.21 Catabolism of Various Organic Food Molecules Copyright © 2016 Pearson Education, Inc. All Rights Reserved Biochemical Tests and Bacterial Identification (3 of 3) Fermentation test: bacteria that catabolize carbohydrate or protein produce acid, causing the pH indicator to change color Oxidase test: identifies bacteria that have cytochrome oxidase (e.g., Pseudomonas) Copyright © 2016 Pearson Education, Inc. All Rights Reserved Photosynthesis (2 of 3) Light-dependent (light) reactions: conversion of light energy into chemical energy (ATP and NADPH) Light-independent (dark) reactions: ATP and NADPH are used to reduce CO2 to sugar (carbon fixation) via the Calvin-Benson cycle Copyright © 2016 Pearson Education, Inc. All Rights Reserved Photosynthesis (3 of 3) Oxygenic: 6 CO2 + 12 H2 O + Light energy C6 H12 O6 + 6 H2 O + 6 O2 Anoxygenic: 6 CO2 + 12 H2S + Light energy C6H12O 6 + 6 H2O + 12 S Copyright © 2016 Pearson Education, Inc. All Rights Reserved Figure 5.25a Photophosphorylation Copyright © 2016 Pearson Education, Inc. All Rights Reserved Figure 5.25b Photophosphorylation Copyright © 2016 Pearson Education, Inc. All Rights Reserved Metabolic Diversity Among Organisms (2 of 4) Phototrophs use light energy Photoautotrophs use energy in the Calvin-Benson cycle to fix CO2 to sugar – Oxygenic: produces O2 – Anoxygenic: does not produce O2 Copyright © 2016 Pearson Education, Inc. All Rights Reserved Table 5.6 Photosynthesis Compared in Selected Eukaryotes and Prokaryotes TABLE 5.6 Photosynthesis Compared in Selected Eukaryotes and Prokaryotes Prokaryotes Prokaryotes Prokaryotes Characteristic Eukaryotes Cyanobacteria Green Bacteria Purple Bacteria Substance That H atoms of H atoms of H2O Sulfur, sulfur Sulfur, sulfur Reduces CO2 H2O compounds, H2 gas compounds, H2 gas Oxygen Oxygenic Oxygenic (and Anoxygenic Anoxygenic Production anoxygenic) Type of Chlorophyll a Chlorophyll a Bacteriochlorophyll a Bacteriochlorophyll Chlorophyll a or b Site of Chloroplasts Thylakoids Chlorosomes Chromatophores Photosynthesis with thylakoids Environment Aerobic Aerobic (and Anaerobic Anaerobic anaerobic) Copyright © 2016 Pearson Education, Inc. All Rights Reserved Metabolic Diversity Among Organisms (4 of 4) Nutritional Type Energy Source Carbon Source Example Photoautotroph Light CO2 Oxygenic: Cyanobacteria, plants Anoxygenic: Green bacteria, purple bacteria Photoheterotroph Light Organic compounds Green bacteria, purple nonsulfur bacteria Chemoautotroph Inorganic CO2 Iron-oxidizing bacteria Chemical Chemoheterotroph Chemical Organic compounds Fermentative bacteria Animals, protozoa, fungi, bacteria Copyright © 2016 Pearson Education, Inc. All Rights Reserved Microbiology an Introduction Twelfth Edition Chapter 6 Microbial Growth Copyright © 2016 Pearson Education, Inc. All Rights Reserved Microbial Growth Microbial growth = increase in number of cells, not cell size Copyright © 2016 Pearson Education, Inc. All Rights Reserved The Requirements for Growth Physical requirements – Temperature – pH – Osmotic pressure Chemical requirements – Carbon – Nitrogen, sulfur, and phosphorous – Trace elements – Oxygen – Organic growth factors Copyright © 2016 Pearson Education, Inc. All Rights Reserved Physical Requirements Temperature – Minimum growth temperature – Optimum growth temperature – Maximum growth temperature Copyright © 2016 Pearson Education, Inc. All Rights Reserved Physical Requirements – Psychrophiles—cold- – Thermophiles loving (-15 to 200C) Optimum growth temperature of 50 – Mesophiles—moderate- to 60°C temperature-loving (25- Found in hot springs and organic 400C). compost – Psychrotrophs – Hyperthermophiles Grow between 0°C Optimum growth temperature > 80°C and 20-30°C Optimum 4oC Cause food refrigerator food spoilage Copyright © 2016 Pearson Education, Inc. All Rights Reserved Figure 6.2 Food Preservation Temperatures (Psychrotrophs) The Effect of the Amount of Food on its Cooling Rate in a Refrigerator and its Chance of Spoilage Copyright © 2016 Pearson Education, Inc. All Rights Reserved pH Most bacteria grow between pH 6.5 and 7.5 Molds and yeasts grow between pH 5 and 6 Acidophiles grow in acidic environments Acidity inhibits most microbial growth and is used frequently for food preservation (e.g.: pickling). Alkalinity inhibits microbial growth, but not commonly used for food preservation. Copyright © 2016 Pearson Education, Inc. All Rights Reserved pH Acidophiles: “Acid loving”. – Grow at very low pH (0.1 to 5.4) – Lactobacillus produces lactic acid, tolerates mild acidity. Neutrophiles: – Grow at pH 5.4 to 8.5. – Includes most human pathogens. Alkaliphiles: “Alkali loving”. – Grow at alkaline or high pH (7 to 12 or higher) – Soil bacterium Agrobacterium grows at pH 12. Copyright © 2016 Pearson Education, Inc. All Rights Reserved Osmotic Pressure Hypertonic environments (higher in solutes than inside the cell) cause plasmolysis due to high osmotic pressure Copyright © 2016 Pearson Education, Inc. All Rights Reserved Osmotic Pressure Cells are 80 to 90% water. Hypertonic solutions: High osmotic pressure removes water from cell, causing shrinkage of cell membrane (plasmolysis). Used to control spoilage and microbial growth. – Sugar in jelly. – Salt on meat. Hypotonic solutions: Low osmotic pressure causes water to enter the cell. In most cases cell wall prevents excessive entry of water. Microbe may lyse or burst if cell wall is weak. Copyright © 2016 Pearson Education, Inc. All Rights Reserved Osmotic Pressure Halophiles: Require moderate to large salt concentrations. Ocean water contains 3.5% salt. – Most bacteria in oceans. Extreme or Obligate Halophiles: Require very high salt concentrations (20 to 30%). – Bacteria in Dead Sea, brine vats. Facultative Halophiles: Do not require high salt concentrations for growth, but tolerate 2% salt or more. Copyright © 2016 Pearson Education, Inc. All Rights Reserved Chemical Requirements Carbon – Structural backbone of organic molecules – Chemoheterotrophs use organic molecules as carbon – Autotrophs use CO2 Nitrogen – Component of proteins, DNA, and ATP – Most bacteria decompose protein material for the nitrogen source – Some bacteria use NH4+ or NO3- from organic matter – A few bacteria use N2 in nitrogen fixation Copyright © 2016 Pearson Education, Inc. All Rights Reserved Chemical Requirements Sulfur – Used in amino acids, thiamine, and biotin – Most bacteria decompose protein for the sulfur source – Some bacteria use SO4 2 or H2S Phosphorus – Used in DNA, RNA, and ATP – Found in membranes ‒ PO43 is a source of phosphorus Copyright © 2016 Pearson Education, Inc. All Rights Reserved Chemical Requirements Oxygen: Organisms that use molecular oxygen (O2), produce more energy from nutrients than anaerobes. Obligate Aerobes: Require oxygen to live. – Disadvantage: Oxygen is not found in all environments and dissolves poorly in water. – Example: Pseudomonas, common nosocomial pathogen. Copyright © 2016 Pearson Education, Inc. All Rights Reserved Chemical Requirements Facultative Anaerobes: Prefer to use oxygen, but can grow in its absence. Have complex set of enzymes – Examples: E. coli, Staphylococcus, yeasts, and many intestinal bacteria. Obligate Anaerobes: Cannot use oxygen and are harmed by the presence of toxic forms of oxygen. – Examples: Clostridium bacteria that cause tetanus and botulism. Copyright © 2016 Pearson Education, Inc. All Rights Reserved Chemical Requirements Aerotolerant Anaerobes: Can’t use oxygen, but tolerate its presence. Can break down toxic forms of oxygen. Oxygen has no effect on growth. – Example: Lactobacillus carries out fermentation regardless of oxygen presence. Microaerophiles: Require oxygen, but at low concentrations. Sensitive/toxic to oxygen. – Example: Campylobacter. Copyright © 2016 Pearson Education, Inc. All Rights Reserved Trace Elements & Organic Growth Factors Organic compounds obtained from the environment Vitamins, amino acids, purines, and pyrimidines Trace elements: Inorganic elements required in small amounts Usually as enzyme cofactors Include iron, copper, molybdenum, and zinc Copyright © 2016 Pearson Education, Inc. All Rights Reserved Biofilms Microbial communities Form slime or hydrogels that adhere to surfaces – Bacteria communicate cell-to- cell via quorum sensing Share nutrients Shelter bacteria from harmful environmental factors Copyright © 2016 Pearson Education, Inc. All Rights Reserved Biofilms Found in digestive system and sewage treatment systems; can clog pipes 1000x resistant to microbicides Involved in 70% of infections – Catheters, heart valves, contact lenses, dental caries Copyright © 2016 Pearson Education, Inc. All Rights Reserved Culture Media Culture medium: nutrients prepared for microbial growth Sterile: no living microbes Inoculum: introduction of microbes into a medium Culture: microbes growing in or on a culture medium Copyright © 2016 Pearson Education, Inc. All Rights Reserved Culture Media Agar – Complex polysaccharide – Used as a solidifying agent for culture media in Petri plates, slants, and deeps – Generally not metabolized by microbes – Liquefies at 100°C – Solidifies at ~40°C Copyright © 2016 Pearson Education, Inc. All Rights Reserved Culture Media Chemically defined media: exact chemical composition is known – Fastidious organisms are those that require many growth factors provided in chemically defined media Complex media: extracts and digests of yeasts, meat, or plants; chemical composition varies batch to batch Nutrient broth Nutrient agar Copyright © 2016 Pearson Education, Inc. All Rights Reserved Table 6.2 A Chemically Defined Medium for Growing a Typical Chemoheterotroph, Such as Escherichia Coli Table 6.2 A Chemically Defined Medium for Growing a Typical Chemoheterotroph, Such as Escherichia coli Constituent Amount Glucose 5.0 g Ammonium phosphate, monobasic (NH4H2PO4) 1.0 g Sodium chloride (NaCl) 5.0 g Magnesium sulfate (MgSO4·7H2O) 0.2 g Potassium phosphate, dibasic (K2HPO4) 1.0 g Water 1 liter Copyright © 2016 Pearson Education, Inc. All Rights Reserved Table 6.4 Composition of Nutrient Agar, a Complex Medium for the Growth of Heterotrophic Bacteria Table 6.4 Composition of Nutrient Agar, a Complex Medium for the Growth of Heterotrophic Bacteria Constituent Amount Peptone (partially digested protein) 5.0 g Beef extract 3.0 g Sodium chloride 8.0 g Agar 15.0 g Water 1 liter Copyright © 2016 Pearson Education, Inc. All Rights Reserved Anaerobic Growth Media and Methods Reducing media – Used for the cultivation of anaerobic bacteria – Contain chemicals (sodium thioglycolate) that combine O2 to deplete it – Heated to drive off O2 Copyright © 2016 Pearson Education, Inc. All Rights Reserved Figure 6.6 A Anaerobic Jar for Cultivating Anaerobic Bacteria on Petri Plates Copyright © 2016 Pearson Education, Inc. All Rights Reserved Figure 6.7 An Anaerobic Chamber Copyright © 2016 Pearson Education, Inc. All Rights Reserved Special Culture Techniques Capnophiles Microbes that require high CO2 conditions – CO2 packet – Candle jar Copyright © 2016 Pearson Education, Inc. All Rights Reserved Special Culture Techniques Biosafety levels – BSL-1: no special precautions; basic teaching labs – BSL-2: lab coat, gloves, eye protection – BSL-3: biosafety cabinets to prevent airborne transmission – BSL-4: sealed, negative pressure; "hot zone" Exhaust air is filtered twice through HEPA filters Copyright © 2016 Pearson Education, Inc. All Rights Reserved Figure 6.8 Technicians in a Biosafety Level 4 (BSL-4) Laboratory. Copyright © 2016 Pearson Education, Inc. All Rights Reserved Selective and Differential Media Selective media – Suppress unwanted microbes and encourage desired microbes – Contain inhibitors to suppress growth of unwanted – Saboraud’s Dextrose Agar pH of 5.6 prevents bacterial growth and used to isolate fungi Copyright © 2016 Pearson Education, Inc. All Rights Reserved Selective and Differential Media Differential media – Allow distinguishing of colonies of different microbes on the same plate Some media have both selective and differential characteristics Copyright © 2016 Pearson Education, Inc. All Rights Reserved Figure 6.10 Differential Medium Copyright © 2016 Pearson Education, Inc. All Rights Reserved Enrichment Culture Encourages the growth of a desired microbe by increasing very small numbers of a desired organism to detectable levels Unlike selective medium, does not necessarily suppress the growth of other microbes. – Used mainly for fecal and soil samples. After incubation in enrichment medium, greater numbers of the organisms, increase the likelihood of positive identification. Usually a liquid Copyright © 2016 Pearson Education, Inc. All Rights Reserved Table 6.5 Culture Media Table 6.5 Culture Media Type Purpose Chemically Defined Growth of chemoautotrophs and photoautotrophs; microbiological assays Complex Growth of most chemoheterotrophic organisms Reducing Growth of obligate anaerobes Selective Suppression of unwanted microbes; encouraging desired microbes Differential Differentiation of colonies of desired microbes from others Enrichment Similar to selective media but designed to increase numbers of desired microbes to detectable levels Copyright © 2016 Pearson Education, Inc. All Rights Reserved Obtaining Pure Cultures A pure culture contains only one species or strain A colony is a population of cells arising from a single cell or spore or from a group of attached cells A colony is often called a colony-forming unit (CFU) The streak plate method is used to isolate pure cultures Copyright © 2016 Pearson Education, Inc. All Rights Reserved Figure 6.11 The Streak Plate Method for Isolating Pure Bacterial Cultures Copyright © 2016 Pearson Education, Inc. All Rights Reserved Preserving Bacterial Cultures Deep-freezing: −50° to −95°C Lyophilization (freeze-drying): frozen (−54° to −72°C) and dehydrated in a vacuum Copyright © 2016 Pearson Education, Inc. All Rights Reserved Bacterial Division Increase in number of cells, not cell size Binary fission Budding (few bacterium) Conidiospores (actinomycetes) Fragmentation of filaments Copyright © 2016 Pearson Education, Inc. All Rights Reserved Figure 6.12a Binary Fission in Bacteria Copyright © 2016 Pearson Education, Inc. All Rights Reserved Generation Time Time required for a cell to divide – 20 minutes to 24 hours Binary fission doubles the number of cells each generation Total number of cells = 2number of generations Growth curves are represented logarithmically Copyright © 2016 Pearson Education, Inc. All Rights Reserved Phases of Growth Lag phase Log phase Stationary phase Death phase Copyright © 2016 Pearson Education, Inc. All Rights Reserved Microbial Phases of Growth Bacterial Growth Curve: When bacteria are inoculated into a liquid growth medium, we can plot of the number of cells in the population over time. Four phases of Bacterial Growth: 1. Lag Phase: Period of adjustment to new conditions. Little or no cell division occurs, population size doesn’t increase. Phase of intense metabolic activity, in which individual organisms grow in size. May last from one hour to several days. Copyright © 2016 Pearson Education, Inc. All Rights Reserved Microbial Phases of Growth 2. Log Phase: Cells begin to divide and generation time reaches a constant minimum. Period of most rapid growth. Number of cells produced > Number of cells dying Cells are at highest metabolic activity. Cells are most susceptible to adverse environmental factors at this stage. Radiation Antibiotics Copyright © 2016 Pearson Education, Inc. All Rights Reserved Microbial Phases of Growth 3. Stationary Phase: Population size begins to stabilize. Number of cells produced = Number of cells dying Overall cell number does not increase. Cell division begins to slow down. Factors that slow down microbial growth: Accumulation of toxic waste materials Acidic pH of media Limited nutrients Insufficient oxygen supply Copyright © 2016 Pearson Education, Inc. All Rights Reserved Microbial Phases of Growth 4. Death or Decline Phase: Population size begins to decrease. Number of cells dying > Number of cells produced Cell number decreases at a logarithmic rate. Cells lose their ability to divide. A few cells may remain alive for a long period of time. Copyright © 2016 Pearson Education, Inc. All Rights Reserved Direct Measurement of Microbial Growth Direct measurements–count microbial cells – Plate count – Filtration – Most probable number (MPN) method – Direct microscopic count Copyright © 2016 Pearson Education, Inc. All Rights Reserved Plate Counts Count colonies on plates that have 30 to 300 colonies (CFUs) To ensure the right number of colonies, the original inoculum must be diluted via serial dilution Counts are performed on bacteria mixed into a dish with agar (pour plate method) or spread on the surface of a plate (spread plate method) Copyright © 2016 Pearson Education, Inc. All Rights Reserved Figure 6.16 Serial Dilutions and Plate Counts Copyright © 2016 Pearson Education, Inc. All Rights Reserved Figure 6.17 Methods of Preparing Plates for Plate Counts Copyright © 2016 Pearson Education, Inc. All Rights Reserved Filtration Solution passed through a filter that collects bacteria Filter is transferred to a Petri dish and grows as colonies on the surface Copyright © 2016 Pearson Education, Inc. All Rights Reserved Direct Microscopic Count Volume of a bacterial suspension placed on a slide Average number of bacteria per viewing field is calculated Uses a special Petroff-Hausser cell counter Number of cells counted Number of bacteria/ml = Volume of area counted Copyright © 2016 Pearson Education, Inc. All Rights Reserved Figure 6.20 Direct Microscopic Count of Bacteria with a Petroff-Hausser Cell Counter Copyright © 2016 Pearson Education, Inc. All Rights Reserved Estimating Bacterial Numbers by Indirect Methods Turbidity—measurement of cloudiness with a spectrophotometer Metabolic activity—amount of metabolic product is proportional to the number of bacteria (glucose consumption) Dry weight—bacteria are filtered, dried, and weighed; used for filamentous organisms Copyright © 2016 Pearson Education, Inc. All Rights Reserved Figure 6.21 Turbidity Estimation of Bacterial Numbers Copyright © 2016 Pearson Education, Inc. All Rights Reserved