Chapter 4 - Part 1 PDF
Document Details
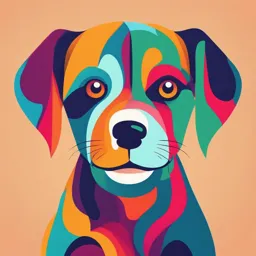
Uploaded by EarnestLake
Gerald Karp
Tags
Related
- SEF045 Biology - Cell Membrane Structure & Function PDF
- Plasma Membrane (Structure) PDF
- Lesson 3: Plasma Membrane Structure and Transport (PDF)
- Lecture 7 - Plasma Membrane Structure and Function (1) PDF
- Cellular Biology - Plasma Membrane Structure PDF
- Plasma Membrane, Structure & Function (BIS 3023) PDF
Summary
This document provides an overview of the structure and function of the plasma membrane. It discusses the role of lipids, proteins, and other components in maintaining membrane integrity and functionality. It also touches on the historical development of understanding cell membranes.
Full Transcript
Chapter - 4 The Structure and Function of the Plasma Membrane Gerald Karp, 9th edition Learning Outcomes: Properties and history of studies on Plasma Membrane (PM) Lipid composition of membranes (Phosphoglycerides, Sphingolipids and Cholesterol) Fluid Mosaic Model Membrane Proteins (Inte...
Chapter - 4 The Structure and Function of the Plasma Membrane Gerald Karp, 9th edition Learning Outcomes: Properties and history of studies on Plasma Membrane (PM) Lipid composition of membranes (Phosphoglycerides, Sphingolipids and Cholesterol) Fluid Mosaic Model Membrane Proteins (Integral., Peripheral and Lipid anchor) Membrane lipids and membrane fluidity Dynamic nature of the PM Ways of solute movement across cell membrane Membrane potential (Resting Potential, Action Potential) Propagation of AP as an impulse Neurotransmitter and its mechanism of action Action of drugs on synapses Introduction to the Plasma Membrane Outer edge of a differentiated muscle cell grown Fig 4.1 Erythrocyte (it was stained with heavy metal called osmium)-dark staining seen by Electron microscopy The trilaminar appearance of membranes as revealed by electron micrograph of the plasma membrane and sarcoplasmic reticulum. • Plasma membrane: The outer boundary of the cell that separates it from the world is a thin, fragile structure about 5 – 10 nm thick. • Not detectable with light microscope need electron microscope. • The 2 dark-staining layers in the electron micrographs correspond primarily to the inner & outer polar surfaces of the bilayer. • All membranes examined closely (plasma, nuclear or cytoplasmic) from plants, animals or microorganisms have the same ultrastructure. Introduction to the Plasma Membrane An Overview of Membrane Functions 1) Compartmentalization 2) Scaffold for biochemical activities 3) Selective permeability barrier 4) Transporting solutes 5) Responding to external signals 6) Intercellular interaction 7) Energy transduction Fig 4.2: A summary of membrane functions in a plant cell. Introduction to the Plasma Membrane An Overview of Membrane Functions 1) Compartmentalization: Membranes form continuous sheets that enclose the cell and intracellular compartments. (hydrolytic enzymes, acid hydrolases are sequestered in a membrane-bound vacuole). 2) Scaffold for biochemical activities: Membranes are themselves compartments. They act as scaffolds. (site of enzyme localization, e.g CO2 fixation in the outer surface of the chloroplast). 3) Selective permeability barrier: Membranes have Gated channels that control the movement of selected molecules (e.g. water molecules can penetrate rapidly through the PM) 4) Transporting solutes: Membrane proteins facilitate the movement of substances form one side to another side. Typically against a concentration gradient (e.g. H+ ions against con. gradient) 5) Responding to external signals: Membrane receptors transduce signals from outside the cell in response to specific ligands. (e.g. plant hormone Abscisic acid, a stress hormone, controls the Calcium efflux from the internal sources and close stomata) 6) Intercellular interaction: Membranes mediate recognition and interaction between adjacent cells.(e.g. openings between adjoining plant cells called plasmodesmata allow the flow of material from one cell into its neighbors). 7) Energy transduction: Membranes are involved in the processes by which one type of energy is converted into another type. (e.g. Conversion of ADP to ATP in the inner membrane of the mitochondrion) How did we discover the composition of plasma membrane? 7 Introduction to the Plasma Membrane First insights of the composition of the outer layer of cells came from: The realization that like dissolves in like For stuff to enter a cell, it must dissolve in the outer layer The more lipid-soluble a solvent is, the more readily it would enter the cell; therefore the layer must be made of lipids A Brief History of Studies on Plasma Membrane Structure Q) How do we know that PM is lipid bilayer? Overton placed plant root hairs into hundreds of different solutions containing a diverse array of solutes. The more lipid-soluble the solute, the more rapidly it would enter the root hair cells He concluded that the dissolving power of the outer boundary layer of the cell matched that of a fatty oil. How? Observe under microscope the changes in the protoplasm’s volume. Tested 500 compunds Introduction to the Plasma Membrane A Brief History of Studies on Plasma Membrane Structure In early 19 century, two Dutch scientist, E.Gorter and F. Grendel proposed that cell membranes might contain a lipid bilayer. How? Calculating the surface area of a lipid preparation They used RBCs to determine the PM chemical nature. Why? • Using red blood cells, their lipid components can cover approximately twice the cell’s surface area Lipid bilayer • Ratio = surface area of water covered by the extracted lipid/surface area of the extracted RBC • The lipid bilayer accounted for the 2:1 ratio of lipid to cell surface area • The most energetically favored orientation for polar head groups is facing the aqueous compartments outside of the bilayer. Langmuir animation: https://www.youtube.com/watch?v=j8yqyRr2VQg Fig 4.3: Bimolecular layer of phospholipids with water soluble head groups facing outward Introduction to the Plasma Membrane • Cell physiologists determined that there must be more to the structure of membranes than simply a lipid bilayer: Lipid solubility was not the sole determining factor as to whether a substance could penetrate the plasma membrane Surface tensions of membranes were calculated to be much lower than those of pure lipid structures - Why? • • These could be explained by the presence of proteins within plasma membranes The proteins themselves can be single proteins, or part of complexes •Remember: the membrane is fluid, and thus dynamic Fig 4.4: Organization of proteins embedded in the lipid bilayer The Lipid Composition of Membranes • Membranes are lipid–protein assemblies held together by noncovalent bonds (fluid mosaic model) – The lipid bilayer is a structural backbone and barrier to prevent random movements of materials into and out of the cell – The proteins determine the specialized activities of that cell type. • The ratio of lipid to protein varies, depending on the type of cellular membrane, the type of organism, and the type of cell: Fig 4.5: Electron micrograph of a nerve cell axon The Lipid Composition of Membranes The Lipid Composition of Membranes Membrane Lipids • Membrane lipids are amphipathic (both hydrophilic and hydrophobic regions) with three main types: – Phosphoglycerides – Sphingolipids – Cholesterol 1. Phosphoglycerides • Lipid containing a phosphate group is called phospholipid • They include the glycerol-backbone and called Phosphoglycerides. Phosphoglycerides or Glycerophospholipids are diglycerides; two hydroxyl groups of glycerol are esterified to fatty acids; the third is esterified to a hydrophilic phosphate group Fatty acid • The kinds and relative proportions of phospholipids vary greatly among types of membranes Q) Can you identify the difference between triglycerides and phosphoglycerides? 1. Phosphoglycerides Most phosphoglycerides have a small hydrophilic group linked to phosphate: choline, ethanolamine, serine or inositol – This group, together with the negatively charged phosphate, forms a highly water-soluble domain, called the head group. Fatty acyl chains are hydrophobic, unbranched hydrocarbons approximately 16 to 22 carbons in length. A fatty acid may be fully saturated, or unsaturated (monounsaturated, or polyunsaturated). Q) What about fats and oils? (saturated, unsaturated?) Phosphoglycerides often contain one unsaturated and one saturated fatty acyl chain. 2. Sphingolipids • Sphingolipids are less abundant class of membrane lipids and are derivatives of sphingosine, an amino alcohol that contains a long hydrocarbon chain • Sphingolipids consist of sphingosine linked to a fatty acid by its amino group (which is then called a ceramide) • Substitutions (subs) to the terminal alcohol of ceramides can create new molecules: Sphingomyelin: the only phospholipid that has no glycerol backbone and composed of ceramide + phosphatidylcholine (e.g. found in nerve tissues, RBCs and ocular lenses) Glycolipid: the molecule is a carbohydrate Cerebroside: the molecule is a simple sugar Ganglioside: small cluster of simple sugar and sialic acid The fatty acyl chains of sphingolipids are longer and more saturated than Phosphoglycerides Nervous system is rich in glycolipids Fig 4.6: 2. Sphingolipids Cerebrosides are neutral glycolipids; each molecule has an uncharged sugar as its head group A ganglioside has an oligosaccharide head group with one or more negatively charged sialic acid residues • People who cannot synthesize ganglioside (G M3) suffer from a serious neurological disease and results in severe seizures and blindness Cerebrosides and gangliosides are especially prominent in brain and nerve cells Human ABO blood group is an example of glycosphingolipids and they have an enzyme that adds N-acetylgalactosamine to the end of the RBC membrane glycolipids Review Slide of chemical structures of membrane lipids Polar P Glycerol Fatty acid chain The chemical structure of membrane lipids Fig 4.6: Summary Slide for Lipids serine Sphingosine Fatty acid R OH NH2 Sphingosine+Fatty acid= Ceramide) choline OR (an 18 carbon amino alcohol with an unsaturated hydrocarbon chain) Sphingolipids (Ceramide) NH2 Sphingomyelin (no glycerol backbone) Ceramide+Phosphatidylcholine =Sphingomyelin Summary Slide for Lipids OR NH2 Sphingolipids (Ceramide) choline Sphingomyelin Cereboside Ganglioside 3. Cholesterol • Cholesterol is a smaller and less amphipathic lipid . • Can make up to 50% of animal membrane lipids • The hydrophilic -OH groups are oriented toward membrane surfaces • Carbon rings are flat and rigid; interfere with movement of phospholipid fatty acid tails • Plant cells contain cholesterol-like compounds (sterols) that fulfill a similar function like membrane fluidity and permeability Fig 4.7: Cholesterol molecules (green) oriented with their small hydrophilic end facing the external surface of the bilayer The Lipid Composition of Membranes The Nature and Importance of the Lipid Bilayer The Lipid Composition of Membranes • Cells membranes have distinct lipid compositions, differing in lipid types, head groups, and species of fatty acyl chain(s) – Lipid composition can determine the physical state of the membrane and influence membrane protein activity • The entire lipid bilayer is only about 5-10 nm thick; and since hydrocarbon chains are not exposed to the aqueous environment, membranes are always continuous, unbroken, and very dynamic (fluid/flexible) structures • All these physical traits allows the cell to function, examples are: – Cell division and the formation of secretion vesicles – Maintaining the proper internal environment (i.e keeping chemical and electrical gradients, amongst other things) The Lipid Composition of Membranes The Nature and Importance of the Lipid Bilayer • An important feature of the lipid bilayer is its ability to selfassemble- HOW? • In vitro, we can add phospholipid molecules and they would assemble spontaneously to form liposomes, with a bilayer structure • Liposomes are vehicles to deliver drugs or DNA within the body; they can be linked to the liposome wall or contained at high concentration within its lumen. The walls of the liposomes are constructed to contain specific proteins (hormone, antibody) and take them to the target cells. • To protect the liposomes from the immune destruction, stealth liposome is created that contains a polymer called polyethylene glycol (PEG). • Liposomes are good to treat some medicines such as chemotherapy or fungal infections that have toxic effect on the healthy cells or could damage organs. https://www.youtube.com/watch?v=vUqwIL5lgS8 Fig 4.9: Liposomes: synthetic vesicles Question Are the various lipids in a membrane randomly distributed between the two monolayers of what makes up the lipid bilayer? The Lipid Composition of Membranes The Asymmetry of the Membrane Lipids • The lipid bilayer consists of two distinct leaflets that have a distinctly different lipid composition- How do we know? • (experiment: treated intact RBC with lipid digesting enzyme called phospholipase) • https://figures.boundless-cdn.com/20013/large/0302phospholipid-bilayer.jpeg -found that 80% of PC, 20% PE and 10% PS of the membrane is hydrolyzed • The different lipids exhibit different properties, therefore providing distinct functionalities to each leaflet • For example, glycolipids are found on the exoplasmic side, while proteins responsible for transmitting signals from the membrane to the inside of the cell are in the cytosolic side • Phosphatidylethanolamine is in the inner leaflet and tends to promote the curvature of the membrane Fig 4.10: • Thus, the lipid bilayer is composed of two semi-stable, independent monolayers having different physical and chemical properties SM: sphingomyelin PC: phosphatidylcholine PS: phosphatidylserine PE: phosphatidylethanolamine PI: phosphatidylinositol Cl: cholesterol Membrane Proteins: The Mosaic Part of the Fluid Mosaic Model • Mosaic: all membranes are lipidprotein constructs • Strong support for this model came from freeze-fracture experiments • Freeze-fracture technique divides the phospholipid leaflets of the membrane. Membrane Proteins • Membrane proteins have different affinities to the core (lipophilic) of membranes, and so they differ in the way they interact with the lipid bilayer • Membrane proteins are grouped into 3 classes: Integral Peripheral Lipid-anchored Membrane Proteins: Integral Proteins • Integral proteins: Penetrate and pass through lipid bilayer (i.e. transmembrane); make up 25 -30% of all encoded proteins and 60% of current drug targets Spanning segment • These proteins act mostly as: – Receptors – Channels (for ions and other solutes) – Agents for electron transport • These proteins are amphipathic: Fig 4.13a: – Hydrophobic domains anchor them in the bilayer (van der Waals with fatty acyl chains); this creates a seal which maintains the permeability barrier – Hydrophilic regions (mostly globular proteins) form functional domains outside of the bilayer Membrane Proteins: Integral Proteins • Hydrophobic transmembrane domains make integral membrane proteins difficult to isolate in a soluble form • Their isolation from membranes requires detergents like ionic SDS, which denatures proteins, or nonionic Triton X-100, doesn’t alter a protein’s tertiary structure (diagram) – Detergents are amphipathic and can substitute for phospholipids in stabilizing and solubilizing integral proteins • After purification, the protein’s amino acid composition, molecular mass, and amino acid sequence can be determined Ionic Detergent https://www.bio-rad.com/ Fig 4.16: Solubilization of membrane proteins with detergents Non-Ionic Detergent Can you quantify protein or see its expression after isolating it from the cells? •PLoS ONE 8(3):e58181 Membrane Proteins: Integral Proteins • Some of the problems facing scientists studying these proteins are: (1) They are present at low numbers per cell (2) They are unstable in the detergent-containing solutions required for their extraction (3) They are prone to aggregation (4) They are heavily glycosylated and cannot be expressed as recombinant proteins in other types of cells Membrane Proteins: Integral Proteins Identifying Transmembrane Domains • Protein segments embedded within the membrane, or transmembrane domains, have a string of about 20 mostly nonpolar amino acids that span the lipid bilayer as an a helix • Fully charged residues can be found in transmembrane helices, but they tend to be near one of the ends of the helix close to the polar environment Fig 4.18: Glycophorin A, an integral protein with a single transmembrane domain with a Gly-X-X-X-Gly sequence Q)How do scientists know the structure of a membrane protein and its orientation within the lipid bilayer? Membrane Proteins: Integral Proteins Identifying Transmembrane Domains • By knowing the amino acid sequence of an integral protein, we can identify transmembrane segments using a hydropathy plot • Hydrophobicity of amino acids can be determined from their lipid solubility or energy required to transfer them from a nonpolar into an aqueous medium – Transmembrane segments are peaks in the hydrophobic side of the spectrum • Orientation of the transmembrane segment within the bilayer? – The flanking side that is more positively charged (amino acids) is typically extended toward the cytoplasmic side! Fig 4.20: Hydropathy plot example Membrane Proteins: Peripheral Proteins • Peripheral proteins: Attached to the membrane by weak electrostatic interactions and are located entirely outside of bilayer on either the extracellular or cytoplasmic side (noncovalent bonding to the polar head group of the lipid bilayer and/or to an integral membrane protein) • They are easily solubilized and extracted with highsalt solutions • Peripheral proteins typically have a dynamic relationship with the membrane, being recruited or released as needed • They can act as: – – – Mechanical support for membranes Enzymes (Electron Transport Chain) Factors that transmit signals Fig 4.13 b: Peripheral proteins Membrane Proteins: Lipid-anchored proteins • Lipid-anchored membrane proteins are covalently linked to a lipid molecule that is situated within the bilayer • They are distinguished both by the types of lipid anchor and their orientation: • – On the outer-leaflet, lipid-anchored proteins are mostly associated via an oligosaccharide to a phosphatidylinositol – While inner-leaflet proteins are anchored to membrane lipids by long hydrocarbon chains They act as: – – Adhesion molecules Enzymes Lipid-anchored proteins Fig 4.13 c: Review Properties and history of studies on Plasma Membrane (PM) Lipid composition of membranes (Phosphoglycerides, Sphingolipids and Cholesterol) Fluid Mosaic Model Membrane Proteins (Integral., Peripheral and Lipid anchor) Factors affecting fluidity Q) Why membrane fluidity is so important? Q) Is it easy to move things in highly ordered and organized structure? Q) Can a non-viscous environment hold structure? Q) What should be the best? Membrane Lipids and Membrane Fluidity • The physical state of membrane lipids can be described by their fluidity (or viscosity) • At higher temperatures ( e.g. 37oC), the lipid of membranes exists in a relatively fluid state, and is described as a two-dimensional liquid crystal – Molecules retain a specified orientation; the long axes are parallel, yet individual lipids can rotate around their axis or move laterally within the plane. • If the temperature is slowly lowered to the transition temperature, the lipid is converted to a frozen crystalline gel and movement is greatly restricted Fig 4.23: Structure of the lipid bilayer depends on the temperature: a) above and b) below the transition temperature. Membrane Lipids and Membrane Fluidity http://www.nutrientsreview.com/wp-content/uploads/2014/12/Trans-cis-fatty-acid.jpg • • Saturated fatty acids resemble a straight, flexible rod. Unsaturated fats could either be cis or trans fats, cis-unsaturated fatty acids have crooks in the chain at the sites of a double bond. Cis fats are beneficial and promote good cholesterol while trans fats are harmful to cardiovascular health (especially from unnatural sources) • Factors affecting membrane fluidity: – Saturated chains pack together more tightly than those containing unsaturated chains – The greater the degree of unsaturation of the fatty acids of the bilayer, the lower the temperature before the bilayer gels – The shorter the fatty acyl chains, the lower its melting temperature. • Cholesterol abolishes sharp transition temperatures and creates a condition of intermediate fluidity: dampens temperature effects Membrane Lipids and Membrane Fluidity Q) Can you explain the transition temperature for two membranes? The first membrane has a transition temperature of 7 oC and the other has 32 oC. Hydrocarbon chain? Degree of saturation? Less hydrocarbons- more fluidity More double bonds-more fluidity Membrane Lipids and Membrane Fluidity Q) Keeping in mind the lipid contents, do you think phospholipid contents will be changed in summer or winter if you analyzed the membrane by taking tissue samples from the organisms? WHY? You collected membrane samples from the same animal in winter and in summer and analyzed the lipid contents. What would be the lipid composition of membrane when you analyze it in winter versus summer. Think in terms of hydrocarbons and unsaturation https://www.google.com/imgres?imgurl=https%3A%2F%2Fmedia.istockphoto.com%2Fid %2F152 https://www.google.com/url?sa=i&url=https%3A%2F%2Fwww.dreamstime.com%2Fphotos-images% In winter -less hydrocarbons and unsaturated (lower degree of saturation) than the membrane in summer. Membrane Lipids and Membrane Fluidity The Importance of Membrane Fluidity • Membrane fluidity provides a compromise between a rigid, ordered structure lacking mobility and a completely fluid, non-viscous liquid lacking structural organization and mechanical support • Molecules can come together, carry out a necessary reaction, and move apart • Membranes arise from preexisting membranes, and their growth occurs by the insertion of lipids and proteins into the fluid matrix • Cellular processes, including cell movement, cell growth, cell division, formation of intercellular junctions, secretion, and endocytosis, depend on the movement of membrane components and would probably not be possible if membranes were rigid, non-fluid structures Membrane Lipids and Membrane Fluidity Maintaining Membrane Fluidity • Internal temperatures of most organisms fluctuate with the external temperature, so cells respond by altering phospholipid composition. If the temperature is lowered, cells can remodel membranes to make them more cold resistant (i.e. keep them fluid at colder temperatures) • Remodeling is accomplished by: – (1) desaturating single bonds in fatty acyl chains to form double bonds, and – (2) reshuffling chains between different phospholipid molecules to make ones that have two unsaturated fatty acids • Desaturation is catalyzed by desaturases • Reshuffling is accomplished by phospholipases, which split the fatty acid from the glycerol backbone, and acyl- transferases, which transfer fatty acids between phospholipids • In addition, the cell changes the types of phospholipids being synthesized in favor of ones containing more unsaturated fatty acids at colder temperatures The Dynamic Nature of the Plasma Membrane • A phospholipid can move laterally within the same leaflet with considerable ease – A phospholipid can diffuse from one end of a bacterium to the other end in ~ 1 sec., but it takes hours to days to move across to the other leaflet • To flip-flop, the hydrophilic head of the lipid must pass through the internal hydrophobic sheet of the membrane not too favourable! • Flippases are enzymes that move certain phospholipids from one leaflet to the other • Visualizing the fact that membrane proteins can move became the cornerstone of the “fluid mosaic model” Fig 4.25: The possible movements of phospholipids in a membrane The Dynamic Nature of the Plasma Membrane Q) Are proteins stagnant or mobile in the plasma membrane? The Dynamic Nature of the Plasma Membrane The Diffusion of Membrane Proteins after Cell Fusion Cell fusion to reveal mobility of membrane proteins: fusion of human and mouse cells • Cell fusion is a technique whereby two different types of cells, or cells from two different species, can be fused to produce one cell with a common cytoplasm and a single, continuous plasma membrane – • Fig 4.26: Cell fusion be induced by certain viruses, electric shocks, or with polyethylene glycol Labeled proteins have shown that membrane proteins can move between fused cell The Dynamic Nature of the Plasma Membrane Restrictions on Protein and Lipid Mobility • Proteins can be labeled and tracked by fluorescence recovery after photobleaching (FRAP) and single particle tracking (SPT) • If the labelled proteins are mobile, then random movements of these molecules should produce a gradual reappearance of fluorescence (rate) • The extent of recovery provides a measure of the percentage of molecules that are free to move • It turns out: Measuring the diffusion rates of membrane proteins by FRAP: variable nature of fluorescence recovery is dependent upon the protein examined – 1) in live cells, movement is slow; – 2) only 30-70 % are mobile In single-particle tracking (SPT) , individual membrane protein molecules are labeled, usually with fluorescent molecular tags that emit light under a microscope Fig 4.27: The Dynamic Nature of the Plasma Membrane Control of Membrane Protein Mobility • Proteins can be: – A: Randomly mobile (A), – B: Immobile- because of underlying skeleton – C: Mobile in a directed manner as a result of interaction with other proteins • Protein movements are limited by interactions with: – D: Interaction with other integral proteins – E: The cytoskeleton (restricted by fences formed by proteins of the membrane skeleton – F: Restricted by extracellular materials Fig 4.28: Patterns of movement of integral membrane proteins The Dynamic Nature of the Plasma Membrane Q) Do lipids move freely or their diffusion is restricted like proteins? The Dynamic Nature of the Plasma Membrane Membrane Lipid Mobility • Just like membrane proteins, phospholipid diffusion is also restricted within the bilayer (even though they are smaller) • Phospholipids are confined for very brief periods to certain areas and then hop from one confined (by “fences”) area to another • Fences restricting motion are constructed of rows of integral membrane proteins bound to the membrane skeleton by their cytoplasmic domains Fig 4.29: Experimental demonstration that diffusion of phospholipids within the plasma membrane is confined The Dynamic Nature of the Plasma Membrane Membrane Domains and Cell Polarity • Most membranes vary in protein composition and mobility (i.e. they do not have a homogenous plasma membrane) • Example: Epithelial cells of the intestinal wall or kidney tubules are highly polarized whose surfaces carry out different functions – The apical plasma membrane absorbs substances from the lumen and possesses different enzymes – The lateral plasma membrane interacts with neighboring epithelial cells – The basal membrane adheres to an underlying basement membrane Fig 4.30: Differentiated functions of the plasma membrane of an epithelial cell. The Dynamic Nature of the Plasma Membrane SEM of human erythrocytes and membrane ghosts SDS–PAGE of membrane proteins Fig 4.32: • The Red Blood Cell: An Example of Plasma Membrane Structure – Homogeneous preparation of membrane “ghosts” can be prepared by hemolysis. – Membrane proteins can be purified and characterized by fractionation using SDS-PAGE electrophoresis. © 2013 John Wiley & Sons, Inc. All rights reserved. Review The physical state of membrane lipids can be described by their fluidity (or viscosity) Factors affecting membrane fluidity: Saturated chains versus unsaturated chains The greater the Degree of unsaturation of the fatty acids of the bilayer, the lower the temperature before the bilayer gels The shorter the fatty acyl chains, the lower its melting temperature. Cholesterol abolishes sharp transition temperatures and creates a condition of intermediate fluidity: dampens temperature effects Less hydrocarbons- more fluidity More double bonds-more fluidity Membrane Fluidity Maintained by (Remodeling) Desaturating bonds (- to = bonds) by reshuffling enzymeschains between different phospholipid molecule (desaturases, phospholipases and acyl-transferases) Flippases are enzymes that move certain phospholipids from one leaflet to the other Are proteins mobile? Cell fusion experiment How can we label and track proteins? Fluorescence recovery after photobleaching (FRAP) and single particle tracking (SPT) How are proteins located in membrane? a) Randomly mobile b) Immobile c) Mobile in a directed manner d) Interaction with integral proteins e) Cytoskeleton (restriction by fences) f) Restricted by extracellular materials