Chapter 4 Enzymes PDF
Document Details
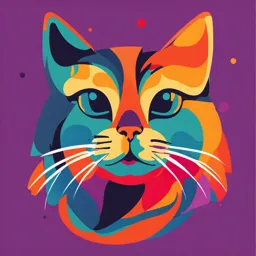
Uploaded by ParamountLlama
Mindanao State University – General Santos
Tags
Summary
This document is an introduction to enzymes, a crucial part of biological processes. It discusses their function in mediating chemical reactions, their impact on life processes, and the historical context of their discovery . The document also details the importance of chemical catalysts in biological reactions, showcasing specific processes and components.
Full Transcript
CHAPTER 4 Enzymes Image: https://www.helmholtz-hips.de/en/news-events/news/detail/news/mirror-mirror-new- enzyme-group-converts-amino-acid-into-its-mirror-image/ Introduction Thermus aquaticus is a rod-shaped bacterium originally discov...
CHAPTER 4 Enzymes Image: https://www.helmholtz-hips.de/en/news-events/news/detail/news/mirror-mirror-new- enzyme-group-converts-amino-acid-into-its-mirror-image/ Introduction Thermus aquaticus is a rod-shaped bacterium originally discovered in a hot spring in Yellowstone National Park Can survive at temperatures between 50°C and 80°C Introduction How do these organisms survive at these extreme temperatures that would cook the life-forms with which we are more familiar? Part of the answer lies in the structure of the enzymes that carry out all the work of the cells. These enzymes are held together by many more attractive forces than the structure of the low-temperature version of the same enzyme. Thus, these proteins are stable and functional even at temperatures above the boiling point of water. Introduction Why was its discovery important? Polymerase Chain Reaction (PCR) is a laboratory technique for rapidly producing (amplifying) millions to billions of copies of a specific segment of DNA, which can then be studied in greater detail. Steps in PCR: Denaturation of helical DNA (94-96˚C) Annealing (68˚C) Elongation (72˚) Taq polymerase from T. aquaticus can withstand the temperature constraints of PCR. Image: https://www.britannica.com/science/polymerase-chain-reaction Introduction Biological catalysis was first recognized and described in the late 1700s, in studies on the digestion of meat by secretions of the stomach. Research continued in the 1800s with examinations of the conversion of starch to sugar by saliva and various plant extracts. In the 1850s, Louis Pasteur concluded that fermentation of sugar into alcohol by yeast is catalyzed by “ferments.” He postulated that these ferments were inseparable from the structure of living yeast cells; this view, called vitalism, prevailed for decades. Then in 1897 Eduard Buchner discovered that yeast extracts could ferment sugar to alcohol, proving that fermentation was promoted by molecules that continued to function when removed from cells. Introduction Frederick W. Kühne later gave the name enzymes (from the Greek “en” = inside and “zymos” = yeast) to the molecules detected by Buchner. The isolation and crystallization of urease by James Sumner in 1926 was a breakthrough in early enzyme studies. Sumner found that urease crystals consisted entirely of protein, and he postulated that all enzymes are proteins. In the absence of other examples, this idea remained controversial for some time. In 1930s John Northrop and Moses Kunitz crystallized pepsin, trypsin, and other digestive enzymes and found them also to be proteins. Introduction During this period, J. B. S. Haldane wrote a treatise titled Enzymes. Although the molecular nature of enzymes was not yet fully appreciated, Haldane made the remarkable suggestion that weak bonding interactions between an enzyme and its substrate might be used to catalyze a reaction. This insight lies at the heart of our current understanding of enzymatic catalysis. Introduction Living organisms seethe with metabolic activity. Thousands of chemical reactions are proceeding very rapidly at any given instant within all living cells. Virtually all of these transformations are mediated by enzymes—proteins (and occasionally RNA; ribozymes) specialized to catalyze metabolic reactions. They catalyze the reactions that break down food molecules to allow the cell to harvest energy. They also catalyze the biosynthetic reactions that produce the great variety of molecules required for cellular life. About a quarter of the genes in the human genome encode enzymes, a testament to their importance to life. Enzymes Proteins as a class of macromolecules are highly effective catalysts for an enormous diversity of chemical reactions because of their capacity to specifically bind a very wide range of molecules. By utilizing the full repertoire of intermolecular forces, enzymes bring substrates together in an optimal orientation, the prelude to making and breaking chemical bonds. They catalyze reactions by stabilizing transition states, the highest-energy species in reaction pathways. By selectively stabilizing a transition state, an enzyme determines which one of several potential chemical reactions actually takes place. https://www.news-medical.net/life-sciences/Enzyme-Mechanisms.aspx; https://byjus.com/chemistry/substrate/ Enzymes Some enzymes require no chemical groups for activity other than their amino acid residues. Others require an additional chemical component called a cofactor—either one or more inorganic ions, such as Fe2+, Mg2+, Mn2+, or Zn2+ (Table 6–1), or a complex organic or metalloorganic molecule called a coenzyme. Enzymes Enzymes Why do apoenzymes need cofactors? Cofactors provide additional chemically reactive functional groups besides those present in the amino acid side chains of apoenzymes. All metal ions must be supplied to the human body through dietary mineral intake. Almost any type of diet will provide adequate amounts of needed metallic cofactors because they are needed in very small (trace) amounts. Coenzymes are synthesized within the human body using building blocks obtained from other nutrients. Most often, one of these building blocks is a B vitamin or B vitamin derivative. Vitamins must be obtained through dietary intake. https://www.toppr.com/ask/en-ae/question/firmly-attached-organic-cofactor-of-holoenzyme-is/ Enzymes A coenzyme or metal ion that is very tightly or even covalently bound to the enzyme protein is called a prosthetic group. A complete, catalytically active enzyme together with its bound coenzyme and/or metal ions is called a holoenzyme. The protein part of such an enzyme is called the apoenzyme or apoprotein. https://www.toppr.com/ask/en-ae/question/firmly-attached-organic-cofactor-of-holoenzyme-is/ Once the cofactor binds to the apoenzyme (b), the active site takes on the correct configuration, the enzyme- substrate complex forms, and the reaction occurs. NAD+ Enzymes Permanent attachment is NOT an absolute requirement for a coenzyme to be an active part of an enzyme. Sometimes a coenzyme temporarily binds to the amino acid portion of an enzyme at the time it is needed and then it is released after the reaction has occurred. The coenzyme NAD+ provides an example of such coenzyme behavior. NADH https://sites.tufts.edu/alcoholmetabolism/the-biological-pathway/the-answer/ NAD+ NADP+ FAD Enzymes Finally, some enzyme proteins are modified covalently by phosphorylation, glycosylation, and other processes. Many of these alterations are involved in the regulation of enzyme activity. https://www.thermofisher.com/ph/en/home/life-science/protein- biology/protein-biology-learning-center/protein-biology-resource- library/pierce-protein-methods/overview-post-translational- modification.html#:~:text=Protein%20post%2Dtranslational%20modifications %20(PTMs,or%20degradation%20of%20entire%20proteins. Enzymes The substances transformed in these reactions catalyzed by enzymes are often organic compounds that show little tendency for reaction outside the cell. An excellent example is glucose, a sugar that can be stored indefinitely on the shelf with no deterioration. Most cells quickly oxidize glucose, producing carbon dioxide and water and releasing lots of energy: Glucose represents thermodynamic potentiality: Its reaction with oxygen is strongly exergonic (can release energy), but it doesn’t occur under normal conditions. On the other hand, enzymes can catalyze such thermodynamically favorable reactions, causing them to proceed at extraordinarily rapid rates. Enzymes Nomenclature and classification of enzymes Three important aspects of the enzyme-naming process are the following: 1. The suffix -ase identifies a substance as an enzyme. Thus urease, sucrase, and lipase are all enzyme designations. The suffix -in is still found in the names of some of the first enzymes studied, many of which are digestive enzymes. Such names include trypsin, chymotrypsin, and pepsin. 2. The type of reaction catalyzed by an enzyme is often noted with a prefix. An oxidase enzyme catalyzes an oxidation reaction hydrolase enzyme catalyzes a hydrolysis reaction 3. The identity of the substrate is often noted in addition to the type of reaction. glucose oxidase – catalyzes the oxidation of glucose pyruvate carboxylase – catalyzes the carboxylation of pyruvate succinate dehydrogenase – catalyzes the dehydrogenation of succinate Infrequently, the substrate but not the reaction type is given: e.g. urease – catalyzes the hydrolysis of urea lactase – catalyzes the hydrolysis of lactose Nomenclature and classification of enzymes Enzymes are grouped into six major classes on the basis of the types of reactions they catalyze. 1. Oxidoreductase – catalyzes an oxidation–reduction reaction. Because oxidation and reduction are NOT independent processes but linked processes that must occur together, an oxidoreductase requires a coenzyme that is oxidized or reduced as the substrate is reduced or oxidized Nomenclature and classification of enzymes An organic oxidation reaction is an oxidation that increases the number of C—O bonds and/or decreases the number of C—H bonds An organic reduction reaction is a reduction that decreases the number of C—O bonds and/or increases the number of C—H bonds. Increased C-O, Increased C-H Decreased C-H Phenolase and enzymatic browning Phenolase and enzymatic browning Can be prevented or slowed in several ways: 1. Immersion in cold water slows the browning process. The lower temperature decreases enzyme activity, and the water limits the enzyme’s access to oxygen. 2. Refrigeration slows enzyme activity even more 3. boiling temperatures destroy (denature) the enzyme. A long-used method for 4. Phenolase works very slowly in the acidic environment created by the lemon juice. In addition, the vitamin C (ascorbic acid) present in lemon juice functions as an antioxidant. It is more easily oxidized than the phenolic-derived compounds, and its oxidation products are colorless. Nomenclature and classification of enzymes 2. Transferase - catalyzes the transfer of a functional group from one molecule to another. Two major subtypes: a. transaminase - transfer of an amino group from one molecule to another. b. Kinases - transfer of a phosphate group from adenosine triphosphate (ATP) to give adenosine diphosphate (ADP) and a phosphorylated product (a product containing an additional phosphate group); Kinases play a major role in energy-harvesting processes involving ATP. Nomenclature and classification of enzymes Nomenclature and classification of enzymes Transglutaminases catalyze a reaction between a glutamine residue in a protein and a lysine residue in the same or another protein, resulting to the formation of large polymers of protein that are very tightly linked to one another. Meat glue can be used to make consistent, uniform portions of meat or fish from smaller scraps. https://www.esclusivoinc.com/sh op/frozen-fish/frozen-imitation- crab-stick-9cm-sushi-stick/ Nomenclature and classification of enzymes 3. Hydrolase - catalyzes a hydrolysis reaction in which the addition of a water molecule to a bond causes the bond to break. Nomenclature and classification of enzymes 3. Hydrolase - catalyzes a hydrolysis reaction in which the addition of a water molecule to a bond causes the bond to break. If fresh pineapple, kiwi, or papaya is added to a gelatin dessert powder, such as Jello, the gelatin will not gel. Why is this so? Water molecules in the gelatin solution become trapped within the gelatin network, forming a semi-rigid gel. These fruits contain a protease (a hydrolase) that catalyzes the hydrolysis of peptide (amide) linkages in gelatin preventing the hydrogel from forming. If canned pineapple is added to gelatin it will gel. The protease present is deactivated when the pineapple was cooked prior to packaging. https://www.science-sparks.com/jelly-will-it-set/; https://chembam.com/resources-for-students/the- chemistry-of/gelatin/ Nomenclature and classification of enzymes 4. Lyase - catalyzes the addition of a group to a double bond or the removal of a group (H2O, CO2, NH3) to form a double bond in a manner that does not involve hydrolysis or oxidation; Nomenclature and classification of enzymes 5. Isomerase - catalyzes the isomerization (rearrangement of atoms) of a substrate in a reaction, converting it into a molecule isomeric with itself. Nomenclature and classification of enzymes 6. Ligase - catalyzes the bonding together of two molecules into one with the participation of ATP Nomenclature and classification of enzymes 6. Ligase - catalyzes the bonding together of two molecules into one with the participation of ATP Nomenclature and classification of enzymes 1. The AMP nucleotide, which is attached to a lysine residue in the enzyme’s active site, is transferred to the 5′-phosphate. 1 2. The AMP—phosphate bond is attacked by the 3′-OH, forming the covalent bond and releasing AMP. To allow the enzyme to carry out further reactions, ATP must replenish the AMP in the enzyme’s active site. 2 https://bitesizebio.com/10279/how-dna-ligation-works/ Malate dehydrogenase peptidase Aspartate transaminase (a transferase) Gibbs Free Energy Is a Useful Thermodynamic Function for Understanding Enzymes Gibbs Free Energy (G) – A thermodynamic property that is a measure of useful energy, or the energy that is capable of doing work. To understand how enzymes operate, we need to consider only two thermodynamic properties of the reaction: (1) the free-energy difference (∆G) between the products and reactants (2) the energy required to initiate the conversion of reactants into products (Ea). Gibbs Free Energy Is a Useful Thermodynamic Function for Understanding Enzymes The free-energy change provides information about the spontaneity but NOT the rate of a reaction The free-energy change of a reaction (∆G) tells us if the reaction can take place spontaneously: 1. A reaction can take place spontaneously only if ∆G is negative (∆G0). An input of free energy is required to drive such a reaction. These reactions are termed endergonic. Gibbs Free Energy Is a Useful Thermodynamic Function for Understanding Enzymes 4. The ∆G of a reaction depends only on the free energy of the products (the final state) minus the free energy of the reactants (the initial state). The ∆G of a reaction is independent of the molecular mechanism of the transformation. For example, the ∆G for the oxidation of glucose to CO2 and H2O is the same whether it takes place by combustion or by a series of enzyme-catalyzed steps in a cell. 5. The ∆G provides no information about the rate of a reaction. A negative ∆G indicates that a reaction can take place spontaneously, but it does not signify whether it will proceed at a perceptible rate. The rate of a reaction depends on the free energy of activation (∆G‡ ), which is largely unrelated to the ∆G of the reaction. Gibbs Free Energy Is a Useful Thermodynamic Function for Understanding Enzymes How does an enzyme speed up a chemical reaction? It changes the path by which the reaction occurs, providing a lower energy route for the conversion of the substrate into the product(s). Thus, enzymes speed up reactions by lowering the activation energy of the The same equilibrium reaction. The energy difference between point is reached but reactant (substrate) and product is not much more quickly in changed. It is only the activation energy that the presence of an is reduced. enzyme. Enzymes alter only the reaction rate and not the reaction equilibrium. Enzymes Accelerate Reactions by Facilitating the Formation of the Transition State X‡ denotes the transition state: ▪ transitory molecular structure that is no longer the substrate but is not yet the product. ▪ the least-stable and most-seldom occupied species along the reaction pathway because it is the one with the highest free energy. The difference in free energy between the transition state and the substrate is called the Gibbs free energy of activation or simply the activation energy (∆G‡). Enzymes Accelerate Reactions by Facilitating the Formation of the Transition State The formation of an enzyme–substrate complex is the first step in enzymatic catalysis Enzymes bind to and then alter the structure of the substrate to promote the formation of the transition state. What is the evidence for the existence of an enzyme–substrate complex? 1. At constant concentration of enzyme, the reaction rate increases with increasing substrate concentration uncatalyzed until a maximal velocity is catalyzed reached (Figure b; further increases in substrate concentration have no effect on the rate of the reaction) Enzymes Accelerate Reactions by Facilitating the Formation of the Transition State What is the evidence for the existence of an enzyme–substrate complex? 2. The spectroscopic characteristics of many enzymes and substrates change on the formation of an ES complex. These changes are particularly striking if the enzyme contains a colored prosthetic group 3. X -ray crystallography (below) has provided high-resolution images of substrates and substrate analogs bound to the active sites of many enzymes https://www.creative-biostructure.com/x-ray-crystallography-platform_60.htm Enzymes Accelerate Reactions by Facilitating the Formation of the Transition State The active sites of enzymes have some common features 1. The active site is a three-dimensional cleft, or crevice, formed by groups that come from different parts of the amino acid sequence: indeed, residues far apart in the amino acid sequence may interact more strongly than adjacent residues in the sequence, which may be sterically constrained from interacting with one another. In lysozyme, an enzyme that degrades the cell walls of some bacteria, the important groups in the active site are contributed by residues numbered 35, 52, 62, 63, 101, and 108 in the sequence of 129 amino acids. https://www.creative-biostructure.com/x-ray-crystallography-platform_60.htm Enzymes Accelerate Reactions by Facilitating the Formation of the Transition State The active sites of enzymes have some common features 2. The active site takes up a small part of the total volume of an enzyme. Experiments show that the minimum size requires about 100 amino acid and that all amino acids in the protein, not just those at the active site, are ultimately required to form a functional enzyme https://en.wikipedia.org/wiki/Active_site Enzymes Accelerate Reactions by Facilitating the Formation of the Transition State The active sites of enzymes have some common features 3. Active sites are unique microenvironments. Water is usually excluded unless it is a reactant. The nonpolar microenvironment of the cleft enhances the binding of substrates as well as catalysis. Nevertheless, the cleft may also contain polar residues, some of which may acquire special properties essential for substrate binding or catalysis. Enzymes Accelerate Reactions by Facilitating the Formation of the Transition State The active sites of enzymes have some common features 4. Substrates are bound to enzymes by multiple weak attractions. These weak reversible contacts are mediated by electrostatic interactions, hydrogen bonds, and van der Waals forces. Van der Waals forces become significant in binding only when numerous substrate atoms simultaneously come close to many enzyme atoms through the hydrophobic effect. Hence, the enzyme and substrate should have complementary shapes. The directional character of hydrogen bonds between enzyme and substrate often enforces a high degree of specificity, as seen in the RNA-degrading enzyme ribonuclease Enzymes Accelerate Reactions by Facilitating the Formation of the Transition State The active sites of enzymes have some common features 5. The specificity of binding depends on the precisely defined arrangement of atoms in an active site. Because the enzyme and the substrate interact by means of short-range forces that require close contact, a substrate must have a matching shape to fit into the site. lock-and-key model (1890); The lock-and-key model explains the action of numerous enzymes. It is, however, too restrictive for the action of many other enzymes. induced fit model Moreover, the substrate may bind to only certain conformations of the enzyme, in what is called conformation selection. Models of Enzyme Action An enzyme–substrate (ES) complex is the intermediate reaction species that is formed when a substrate binds to the active site of an enzyme. Models of Enzyme Action An enzyme–substrate (ES) complex is the intermediate reaction species that is formed when a substrate binds to the active site of an enzyme. In this model, the enzyme changes shape on substrate binding. The active site forms a shape complementary to the substrate only after the substrate has been bound. Enzymes Accelerate Reactions by Facilitating the Formation of the Transition State The binding energy between enzyme and substrate is important for catalysis Enzymes lower the activation energy, but where does the energy to lower the activation energy come from? Free energy is released by the formation of a large number of weak interactions between a complementary enzyme and its substrate. The free energy released on binding is called the binding energy. Only the correct substrate can participate in most or all of the interactions with the enzyme and thus maximize binding energy, accounting for the exquisite substrate specificity exhibited by many enzymes. Furthermore, the full complement of such interactions is formed only when the substrate is converted into the transition state. Thus, the maximal binding energy is released when the enzyme facilitates the formation of the transition state. The energy released by the interaction between the enzyme and the substrate can be thought of as lowering the activation energy. the full complement of weak interactions is formed only when the substrate is converted into the transition state Enzymes Accelerate Reactions by Facilitating the Formation of the Transition State The binding energy between enzyme and substrate is important for catalysis Enzymes lower the activation energy, but where does the energy to lower the activation energy come from? Binding energy – the free energy that is released by the formation of a large number of weak interactions between a complementary enzyme and its substrate Only the correct substrate can participate in most or all of the interactions with the enzyme and thus maximize binding energy, accounting for the exquisite substrate specificity exhibited by many enzymes. Furthermore, the full complement of such interactions is formed only when the substrate is converted into the transition state. Thus, the maximal binding energy is released when the enzyme facilitates the formation of the transition state. The energy released by the interaction between the enzyme and the substrate can be thought of as lowering the activation energy. The Transition State and Product Formation Transition state - a state in which the substrate is in an energetically unstable intermediate form, having features of both the substrate and the product The Transition State and Product Formation What kinds of transition state changes might occur in the substrate that would make a reaction proceed more rapidly? 1. The enzyme might put “stress” on a bond and thereby promote bond breakage The Transition State and Product Formation What kinds of transition state changes might occur in the substrate that would make a reaction proceed more rapidly? 2. An enzyme may facilitate a reaction by bringing two reactants close to one another and in the proper orientation for reaction to occur. The Transition State and Product Formation What kinds of transition state changes might occur in the substrate that would make a reaction proceed more rapidly? 2. The active site of an enzyme may modify the pH of the microenvironment surrounding the substrate. For instance, it may serve as a donor or an acceptor of H+. This would cause a change in the pH in the vicinity of the substrate without disturbing the normal pH elsewhere in the cell. (Application) HIV Protease Inhibitors and Pharmaceutical Drug Design structural analogs of the normal HIV protease substrate can be designed and used as candidates as HIV protease inhibitors https://clinicalinfo.hiv.gov/ https://proteopedia.org/wiki/index.php/Immunodeficiency_virus_protease Specificity of the Enzyme-Substrate Complex Enzyme specificity is the extent to which an enzyme’s activity is restricted to a specific substrate, a specific group of substrates, a specific type of chemical bond, or a specific type of chemical reaction. The degree of enzyme specificity is determined by the active site. Specificity of the Enzyme-Substrate Complex Types of specificity 1. Absolute specificity—the enzyme will catalyze only one reaction. This most restrictive of all specificities is not common. Examples: Aminoacyl tRNA synthetases exhibit absolute specificity. For many synthetases, selectivity is achieved by binding to a pocket in the catalytic site, the location where the charging reaction takes place. The correct amino acid has the highest binding affinity for the binding pocket of the catalytic site; therefore, its binding is favored over the other 19 amino acids. Amino acids that are larger than the correct one are excluded from the catalytic site based on size. Also, amino acids whose side chains display a negative charge could be excluded from the binding pocket of the catalytic site for an amino acid whose side chain carries a positive charge. Therefore, in many cases, differences in size and chemistry among amino acids suffice for effective discrimination. Catalase catalyzes the conversion of hydrogen peroxide (H2O2) to O2 and H2O. Hydrogen peroxide is the only substrate it will accept. In some cases, however, the synthetase must distinguish between amino acids that are so similar in size and chemical properties that effective discrimination based solely on selective binding is not possible. https://www.labxchange.org/library/items/lb:LabXchange:71db3759:html:1 https://alchetron.com/Aminoacyl-tRNA-synthetase Specificity of the Enzyme-Substrate Complex Types of specificity 2. Group specificity—the enzyme will act only on molecules that have a specific functional group, such as hydroxyl, amino, or phosphate groups. Examples: Carboxypeptidase is group specific; it cleaves amino acids, one at a time, from the carboxyl end of a peptide chain. Hexokinase catalyzes the addition of a phosphoryl group to the hexose sugar glucose in the first step of glycolysis. Hexokinase can also add a phosphoryl group to several other six-carbon sugars. Specificity of the Enzyme-Substrate Complex Types of specificity 3. Linkage specificity—the enzyme will act on a particular type of chemical bond, irrespective of the rest of the molecular structure. Linkage specificity is the most general of the common specificities. Example: Phosphatases hydrolyze phosphate–ester bonds in all types of phosphate esters. Proteases, such as trypsin, chymotrypsin, and elastase, are enzymes that selectively hydrolyze peptide bonds. Thus, these enzymes are linkage specific. 4. Stereochemical specificity—the enzyme will act on a particular stereoisomer. Chirality is inherent in an enzyme active site because amino acids are chiral compounds. An l-amino acid oxidase will catalyze the oxidation of the L-form of an amino acid but not the D-form of the same amino acid. Because we use only D-sugars and L-amino acids, the enzymes involved in digestion and metabolism recognize only those particular stereoisomers. Factors That Affect Enzyme Activity Enzyme activity is a measure of the rate at which an enzyme converts substrate to products in a biochemical reaction. Factors that affect enzyme activity include: (1) Temperature (2) pH (3) substrate concentration, and (4) enzyme concentration Environmental effects: Temperature Increased number of (1) Temperature substrate-enzyme collisions Maximum rate Temperature is a measure of the kinetic energy (energy of denaturation motion) of molecules. At higher temperatures molecules are moving faster and colliding more frequently. This concept applies to collisions between substrate molecules and enzymes. As Optimum T the temperature of an Uncatalyzed Catalyzed enzymatically catalyzed reaction increases, so does the Beyond the optimum T, the increased energy begins to cause rate (velocity) of the reaction. disruptions in the tertiary structure of the enzyme (denaturation) Environmental effects: Temperature For human enzymes, the optimum temperature is around 37°C, normal body temperature. A person who has a fever where body core temperature exceeds 40°C can be in a life- threatening situation because such a temperature is sufficient to initiate enzyme denaturation. The “destroying” effect of temperature on bacterial enzymes is used in a hospital setting to sterilize medical instruments and laundry. In high-temperature, high-pressure vessels called autoclaves, super-heated steam is used to produce a temperature sufficient to denature bacterial enzymes. Although instruments can be sterilized by dry heat (160°C) applied for at least 2 hours (h) in a dry air oven, autoclaving is a quicker, more reliable procedure. The autoclave works on the principle of the pressure cooker. Air is pumped out of the chamber, and steam under pressure is pumped into the chamber until a pressure of 2 atmospheres (atm) is achieved. The pressure causes the temperature of the steam, which would be 100°C at atmospheric pressure, to rise to 121°C. Within 20 minutes (min), all the bacteria and viruses are killed. Additional info that you may find interesting so I’m sharing it. LOL. https://surfguppy.com/colligative-property/how-does-atmospheric-pressure-affect-boiling-point/ Environmental effects: pH (2) pH Optimum pH is the pH at which an enzyme exhibits maximum activity. The charge on acidic and basic amino acids located at the active site depends on pH. Small changes in pH (less than one unit) can result in enzyme denaturation and subsequent loss of catalytic activity. Biochemical buffers help maintain the optimum pH for an enzyme. Environmental effects: pH Optimum pH usually falls within the physiological pH range of 7.0–7.5. Exceptions: Pepsin, which is active in the stomach, functions best at a pH of 2.0. On the other hand, trypsin, which operates in the small intestine, functions best at a pH of 8.0. The amino acid sequences present in pepsin and trypsin are those needed such that the R groups present can maintain protein tertiary structure at low (2.0) and high (8.0) pH values, respectively. At pH outside the optimum, protonation or These acidic conditions significantly reduce deprotonation of groups on the substrate could also the enzymatic activity of any harmful take place. The interaction between the altered microorganisms present in pickled foods. substrate and the enzyme active site may be less efficient than normal—or even impossible. An interesting bacterial adaptation to an acidic environment occurs within the human stomach. https://www.inspiredtaste.net/57807/cabbage-kimchi/ Chemical Connections: H. pylori and Stomach Ulcers Many years prior to the discovery of the causal agent, stomach and duodenal ulcers were thought to be due to excess stomach acid, emotional stress and spicy food, among others. J. Robin Warren, a clinical pathologist who had examined many stomach biopsy specimens noticed a parallel between the severity of the inflammation and the number of bacteria present. In 1981, he met Barry James Marshall, a trainee doctor. Marshall was looking for a research topic and learned that Warren had a list of patients whose gastric biopsies showed “curved” bacteria. https://www.ahmadcoaching.com/2020/11/what-is-a-lysosome.html Chemical Connections: H. pylori and Stomach Ulcers Marshall was unsuccessful in developing an animal model, so he decided to experiment upon himself. In 1984, following a baseline endoscopy which showed a normal gastric mucosa, he drank a culture of the organism. Three days later he developed nausea and achlorhydria (absence of hydrochloric acid in the gastric secretions). Vomiting occurred and on day 8 a repeat endoscopy and biopsy showed marked gastritis and a positive H. pylori culture. At day 14, a third endoscopy was performed and he then began treatment with antibiotics and bismuth. He recovered promptly and thus had fulfilled Koch’s postulates for the role of H. pylori in gastritis. https://www.ahmadcoaching.com/2020/11/what-is-a-lysosome.html Professor Marshall and Dr Warren were awarded the 2005 Nobel Prize in Medicine for their 1982 discovery of “the bacterium Helicobacter pylori and its role in gastritis and peptic ulcer disease.” Chemical Connections: H. pylori and Stomach Ulcers How do the enzymes present in the H. pylori bacterium can function in the acidic environment of the stomach? Present on the surface of the bacterium is the enzyme urease, an enzyme that converts urea to the basic substance ammonia. The ammonia then neutralizes acid present in its immediate vicinity; a protective barrier is thus created. The urease itself is protected from denaturation by its complex quaternary structure. https://www.ahmadcoaching.com/2020/11/what-is-a-lysosome.html Environmental effects: pH Lysosomes -called “suicide bags” (Christian de Duve, 1956) because they are membrane-bound vesicles containing about fifty different kinds of hydrolases that degrade large biological molecules into small molecules. If the hydrolytic enzymes of the lysosome were accidentally released into the cytoplasm of the cell, the result would be the destruction of cellular macromolecules and death of the cell. Because of this danger, the cell invests a great deal of energy in maintaining the integrity of the lysosomal membranes. An additional protective mechanism relies on the fact that lysosomal enzymes function optimally at an acid pH (pH 4.8). Should some of these enzymes leak out of the lysosome or should a lysosome accidentally rupture, the cytoplasmic pH of 7.0–7.3 renders them inactive. https://www.ahmadcoaching.com/2020/11/what-is-a-lysosome.html Environmental effects: Substrate Concentration Enzyme-catalyzed reaction must occur in two stages: 1. The formation of an enzyme-substrate complex. This binding of the substrate to the active site of the enzyme is a rapid step. 2. Conversion of substrate into product and release of the product and enzyme. This step is slower and limits the rate of the overall reaction. https://www.ahmadcoaching.com/2020/11/what-is-a-lysosome.html Environmental effects: Substrate Concentration At its maximum rate, the active sites of all the enzyme molecules are occupied by a substrate molecule. The active site is the region of the enzyme that specifically binds the substrate and catalyzes the reaction. A new molecule of substrate cannot bind to the enzyme molecule until the substrate molecule already held in the active site is converted to product and released. Ultimately, the reaction rate is dependent on the amount of enzyme that is available. https://www.ahmadcoaching.com/2020/11/what-is-a-lysosome.html Environmental effects: Substrate Concentration An enzyme’s turnover number is the number of substrate molecules transformed per minute by one molecule of enzyme under optimum conditions of temperature, pH, and saturation. Environmental effects: Enzyme Concentration Because enzymes are not consumed in the reactions they catalyze, the cell usually keeps the number of enzymes low compared with the number of substrate molecules. This is efficient; the cell avoids paying the energy costs of synthesizing and maintaining a large work force of enzyme molecules. Thus, in general, the concentration of substrate in a reaction is much higher than that of the enzyme. If the amount of substrate present is kept constant and the enzyme concentration is increased, the reaction rate increases because more substrate molecules can be accommodated in a given amount of time. Extremophiles and Extremozymes An extremophile is a microorganism that thrives in extreme environments, environments in which humans and most other forms of life could not survive. Extremophile environments include the hydrothermal areas of Yellowstone National Park and hydrothermal vents on the ocean floor where temperatures and pressures can be extremely high. Acidophiles (optimal growth at pH levels of 3.0 or below), alkaliphiles (optimal growth at pH levels of 9.0 or above), halophiles (high salinity, a salinity that exceeds 0.2 M NaCl needed for growth), hyperthermophiles (a temperature between 80°C and 121°C needed to thrive), piezophiles (a high hydrostatic pressure needed for growth), xerophiles (extremely dry conditions needed for growth), and cryophiles (a temperature of 15°C or lower needed for growth). https://www.oist.jp/news-center/news/2023/1/18/testing-their- mettle-how-bacteria-deep-sea-vents-deal-toxic-metal-environments Extremophiles and Extremozymes The enzymes present in extremophiles are called extremozymes. An extremozyme is a microbial enzyme active at conditions that would inactivate human enzymes as well as enzymes present in other types of higher organisms. Because industrial processes usually require higher temperatures and pressures than do physiological processes, extremozymes have characteristics that have been found to be useful. The enzymes present in some detergent formulations, which must function in hot water, are the result of research associated with high-temperature microbial enzymes. Similarly, cold-water-wash laundry detergents contain enzymes originally characterized in cold- https://www.heb.com/product-detail/arm- environment microbial organisms. hammer-bio-enzyme-power-botanical- springs-detergent/1975962 Extremophiles and Extremozymes The development of commercially useful enzymes using extremophile sources involves the following general approach: 1. Samples containing the extremophile are gathered from the extreme environment where it is found. 2. DNA material is extracted from the extremophile and processed. 3. Macroscopic amounts of the DNA are produced using the polymerase chain reaction (Next chapter). 4. The macroscopic amount of DNA is analyzed to identify the genes present that are involved in extremozyme production. 5. Genetic engineering techniques are used to insert the extremozyme gene into bacteria, which then produce the extremozyme. 6. The process is then commercialized. https://www.heb.com/product-detail/arm- hammer-bio-enzyme-power-botanical- springs-detergent/1975962 Regulation of enzyme activity Regulation of enzyme activity within a cell is a necessity for many reasons; mainly due to energy conservation (If the cell runs out of chemical energy, it will die). Illustrations: 1. A cell that continually produces large amounts of an enzyme for which substrate concentration is always very low is wasting energy. The production of the enzyme needs to be “turned off.” 2. A product of an enzyme-catalyzed reaction that is present in plentiful (more than needed) amounts in a cell is a waste of energy if the enzyme continues to catalyze the reaction that produces the product. The enzyme needs to be “turned off.” Many mechanisms exist by which enzymes within a cell can be “turned on” (1) feedback control associated with allosteric enzymes, (2) proteolytic enzymes and proenzymes/zymogens, and (3) covalent modification Regulation of enzyme activity: feedback control associated with allosteric enzymes Many, but not all, of the enzymes responsible for regulating cellular processes are allosteric enzymes. ◀ Characteristics of allosteric enzymes are as follows: 1. All allosteric enzymes have quaternary structure; that is, they are composed of two or more protein subunits. 2. All allosteric enzymes have two kinds of binding sites: those for substrate and those for regulators. 3. Active and regulatory binding sites are distinct from each other in both location and shape. In most cases the regulatory site is on one protein chain and the active site is on another. 4. Binding of a molecule at the regulatory site causes changes in the overall three- dimensional structure of the enzyme, including structural changes at the active site. Regulation of enzyme activity: feedback control associated with allosteric enzymes Regulation of enzyme activity: feedback control associated with allosteric enzymes If D is no longer needed, it is a waste of cellular energy to continue to produce it. To avoid this waste of energy, the cell uses feedback inhibition, in which the product can shut off the entire pathway for its own synthesis. In this example, the product, D, acts as a negative allosteric effector on one of the early enzymes of the pathway, E1. When D is present in excess, it binds to the effector-binding site, causing the active site to close so that it cannot bind to substrate A. Thus, A is not converted to B and the entire pathway ceases to operate. The product, F, has turned off all the steps involved in its own synthesis. When the concentration of D drops, it will dissociate from the effector binding site. When this occurs, the enzyme is once again active. Thus, feedback inhibition is an effective metabolic on-off switch. Feedback control is not the only mechanism by which an allosteric enzyme can be regulated; it is just one of the more common ways. Regulators of a particular allosteric enzyme may be products of entirely different pathways of reaction within the cell, or they may even be compounds produced outside the cell (hormones). Regulation of enzyme activity: proteolytic enzymes and proenzymes/zymogens Proenzyme or zymogen – inactive form of enzyme; converted by proteolysis (hydrolysis of the protein) to the active form when it has reached the site of its activity. Most digestive and blood- clotting enzymes are proteolytic enzymes. https://healthjade.net/trypsin/ https://www.quora.com/What-is-the-relationship-between-pepsin-and-pepsinogen Regulation of enzyme activity: covalent modification Covalent modification is a process in which enzyme activity is altered by covalently modifying the structure of the enzyme through attachment of a chemical group to or removal of a chemical group from a particular amino acid within the enzyme’s structure. The most common type of protein modification is phosphorylation (by protein kinases) or dephosphorylation (by phosphatases) of an enzyme. Typically, the phosphoryl group is added to (or removed from) the R group of a serine, tyrosine, or threonine in the protein chain of the enzyme. Notice that these three amino acids have a free —OH in their R group, which serves as the site for the addition of the phosphoryl group. For some enzymes, the active (“turned-on” form) is the phosphorylated version of the enzyme (e.g. triacylglycerol lipase); however, for other enzymes, it is the dephosphorylated version that is active (e.g. glycogen synthase). Inhibition of Enzyme Activity Enzyme inhibitors - chemicals that can bind to enzymes and either eliminate or drastically reduce their catalytic ability. Examples: arsenic binds to the thiol groups of cysteine amino acids in the proteins, interfering with the formation of disulfide bonds needed to stabilize the tertiary structure of enzymes Penicillin inhibits several enzymes that are involved in the synthesis of bacterial cell walls Three (3) modes of inhibition: (1) irreversible inhibition (2) reversible competitive inhibition (3) reversible noncompetitive inhibition Irreversible enzyme inhibitors In general, such inhibitors do NOT have structures similar to that of the enzyme’s normal substrate. Usually bind very tightly, sometimes even covalently, to the enzyme. This generally involves binding of the inhibitor to one of the R groups of an amino acid in the active site. Inhibitor binding may block the active site binding groups so that the enzyme-substrate complex cannot form. Alternatively, an inhibitor may interfere with the catalytic groups of the active site, thereby effectively eliminating catalysis. Irreversible inhibitors, which include snake venoms and nerve gases, generally inhibit many different enzymes. Irreversible enzyme inhibitors The neurotransmitter acetylcholine is released following a nerve impulse It diffuses across the nerve synapse (the space between the nerve and muscle cells) and binds to the acetylcholine receptor protein (R) in the postsynaptic membrane of the muscle cell Na+ and K+ ions then flow into and out of the cell, respectively. This generates the nerve impulse and causes the muscle to contract. Irreversible enzyme inhibitors Sarin Reversible enzyme inhibitors Recall: Molecular shape and charge distribution are key determining factors in whether an enzyme accepts a molecule. Two types: (a) Reversible competitive inhibitors: often referred to as structural analogs; that is, they are molecules that resemble the structure and charge distribution of the natural substrate for a particular enzyme. Because of this resemblance, the inhibitor can occupy the enzyme active site. However, no reaction can occur, and enzyme activity is inhibited. This inhibition is competitive because the inhibitor and the substrate compete for binding to the enzyme active site. The degree of inhibition depends on their relative concentrations. If the inhibitor is in excess or binds more strongly to the active site, it will occupy the active site more frequently, and enzyme activity will be greatly decreased. Folic acid is a vitamin required for the transfer of methyl groups in the biosynthesis of methionine and the nitrogenous bases required to make DNA and RNA. Humans cannot synthesize folic acid and must obtain it from the diet. Bacteria, on the other hand, must make folic acid because they cannot take it in from the environment. para-Aminobenzoic acid (PABA) is the substrate for an early step in folic acid synthesis. If the correct substrate (PABA) is bound by the enzyme, the reaction occurs, and the bacterium lives. However, if the sulfa drug is present in excess over PABA, it binds more frequently to the active site of the enzyme. No folic acid will be produced, and the bacterial cell will die. In addition to the folic acid supplied in our diets, we obtain folic acid from our intestinal bacteria. https://openstax.org/books/microbiology/pages/14-3-mechanisms-of-antibacterial-drugs Reversible competitive inhibitors The formation of an enzyme–competitive inhibitor complex is a reversible process because it is maintained by weak interactions (hydrogen bonds, etc.). With time (a fraction of a second), the complex breaks up. The empty active site is then available for a new occupant. Substrate and inhibitor again compete for the empty active site. Thus the active site of an enzyme binds either inhibitor or normal substrate on a random basis. If inhibitor concentration is greater than substrate concentration, the inhibitor dominates the occupancy process. The reverse is also true. Competitive inhibition can be reduced by simply increasing the concentration of the substrate. Reversible noncompetitive inhibitors A noncompetitive enzyme inhibitor is a molecule that decreases enzyme activity by binding to a site on an enzyme other than the active site. The substrate can still occupy the active site, but the presence of the inhibitor causes a change in the structure of the enzyme sufficient to prevent the catalytic groups at the active site from properly effecting their catalyzing action. Examples of noncompetitive inhibitors include the heavy metal ions Pb2+, Ag+, and Hg2+. The binding sites for these ions are sulfhydryl (—SH; also called thiol) groups located away from the active site. Metal sulfide linkages are formed, an effect that disrupts secondary and tertiary structure. Unlike the situation in competitive inhibition, increasing the concentration of substrate does not completely overcome the inhibitory effect in this case. Reversible noncompetitive inhibitors Proteolytic enzymes The specificity of chymotrypsin depends upon the presence of a hydrophobic pocket, a cluster of hydrophobic amino acids brought together by the three-dimensional folding of the protein chain. The flat aromatic side chains of certain amino acids (tyrosine, tryptophan, phenylalanine) slide into this pocket, providing the binding specificity required for catalysis at the catalytic site These enzymes are called serine proteases because they have the amino acid serine in the catalytic region of the active site that is essential for hydrolysis of the peptide bond. Serine protease mechanism: https://www.youtube.com/watch?v=6kYpu1 eZZHs pancreatic serine proteases